Abstract
Myricetin (Myr) is a naturally occurring flavonoid exhibiting diverse biological and pharmacological properties, but its characteristics such as water insolubility, poor aqueous stability, and poor bioavailability limit its clinical application, including in ophthalmology. To increase its clinical application in ophthalmology, Myr was designed to be encapsulated in a polyvinyl caprolactam–polyvinyl acetate–polyethylene glycol graft copolymer (PVCL-PVA-PEG) polymeric micelles to increases its aqueous solubility, stability, and corneal permeability to promote its efficacy in eye disease treatments. Thus, the Myr micelle ophthalmic solution was prepared and characterized encapsulation efficiency (EE), micelle size, and zeta potential. The chemical stability of Myr and the short-term storage stability of the Myr micelle ophthalmic solution were evaluated, followed by in vitro cytotoxicity and in vivo ocular irritation; in vitro cellular uptake and in vivo corneal permeation; and in vitro antioxidant activity and in vivo anti-inflammatory efficacy were also further evaluated. Myr could be incorporated into micelles with high EE. PVCL-PVA-PEG micelles significantly enhanced Myr’s aqueous solubility and chemical stability. The Myr micelle ophthalmic solution also showed high storage stability. In rabbits, the Myr micelle ophthalmic solution displayed good in vitro cellular tolerance. Remarkable improvements in in vitro cellular uptake and in vivo corneal permeation were also observed in the Myr micelle ophthalmic solution, and significant improvements in the in vitro antioxidant activity and in vivo anti-inflammatory efficacy were also obtained. Overall, these results supported that the Myr micelle ophthalmic solution could be a promising nanomedicine for ocular tissues.
Introduction
Myricetin (Myr), is a natural flavonol compound that possesses multiple pharmacological properties, such as anti-inflammatory, antioxidant, anticancer, and antimicrobial activities, and is beneficial for overall health (Semwal et al., Citation2016; Hou et al., Citation2018; Zhang, Feng et al., Citation2018). Within ophthalmology, many studies have also shown Myr’s potential benefits. The anti-inflammatory property of Myr is beneficial in ocular degenerative and inflammatory diseases, including dry eye and chronic anterior uveitis (Hodges et al., Citation1999; Laabich et al., Citation2007; Chen et al., Citation2014; Yang et al., Citation2018). Myr’s antioxidant activity also aids some eye diseases, especially ocular surface and corneal diseases such as dry eye, which contribute to oxidative stress (Yin et al., Citation2018). Though Myr has various pharmacological benefits and a good safety profile, the lack of an ocular formulation significantly hinders its clinical application. The main reason for this problem lies in its poor water solubility and low aqueous stability (Yao et al., Citation2014; Tang et al., Citation2016; Qian et al., Citation2017). Thus, ocular delivery is a challenging task.
Poorly soluble drugs are usually developed as traditional ocular topical formulations such as suspensions, emulsions, and ointments. However, these formulations usually cause eye discomfort and vision interference, with some even causing adverse effects such as irritation and eye redness. Another approach is the use of lipidic excipients to dissolve the drug, but this often causes adverse effects including discharge and ocular burning. Therefore, a topical ophthalmic formulation for poorly soluble drugs such as Myr that also has high efficacy and good ocular tolerance is desired in ophthalmology.
Self-assembled micelle systems, known as the main delivery method for poorly soluble drugs, may address this need. This system has several advantages, including enhancing drug solubility and improving drug stability. Over the past decades, polymeric micelles have been widely found to be one of the most promising nano-drug delivery systems for the treatment of ocular diseases (Mandal et al., Citation2017). In several reports, polyvinyl caprolactam–polyvinyl acetate–polyethylene glycol graft copolymer (PVCL–PVA–PEG), a new amphiphilic polymeric excipient with low toxicity and high solubilization ability, was selected to form a micelle system to enhance aqueous stability and solubility (Saydam et al., Citation2017; Zeng et al., Citation2017; Wang et al., Citation2018). Some of the advantages of PVCL-PVA-PEG are being an authorized pharmaceutical excipient, and showing high performance, low toxicity, and excellent biocompatibility. (https://pharmaceutical.basf.com/en/Drug-Formulation/Soluplus.html, accessed 30 Dec. 2018) Another is that this polymer has very low critical micelle concentration (CMC, 7.6 mg/L), contributing to the high stability of the self-assembled micelles (Guo, Zhang et al., Citation2015; Ju et al., Citation2018). All these characters indicate PVCL-PVA-PEG’s great potential for fabricating the micelle ophthalmic solution of poorly soluble drugs such as Myr (Guo, Zhang et al., Citation2015; Guo et al., Citation2016; Li et al., Citation2017, Citation2018).
However, there is still a dearth of literature dealing with the development of an ophthalmic solution based on PVCL-PVA-PEG micelles for the solubility, stability, and ocular delivery of Myr (Myr micelle ophthalmic solution). This study might address the above gaps.
Materials and methods
Chemical reagents and animals
Details of materials and animal use are described in the Supporting Information (SI). The animal care and procedures were conducted according to the Principles of Laboratory Animal Care. All animals were healthy and free from clinically observable ocular abnormalities. The use of animals in this study adhered to the Association for Research in Vision and Ophthalmology (ARVO) Statement for the Use of Animals in Ophthalmic and Vision Research, and the animal study was approved by the Qingdao University of Science and Technology Ethics Committee for Animal Experimentation (approval document no. 2017-1, Qingdao, China).
Preparation and characterizations of Myr micelle ophthalmic solution
The Myr micelle ophthalmic solution was prepared by the thin-film hydration method (Guo, Zhang et al., Citation2015; Hu et al., Citation2017; Li et al., Citation2017). Coumarin-6 (Cou6, 0.5 mg) was added to fabricate Cou6-labeled Myr micelles with the same method mentioned above to explore the in vitro cellular uptake/in vivo corneal permeability (Guo, Cui et al., Citation2015; Guo, Zhang et al., Citation2015; Zhou et al., Citation2017). Details of this section of information are described in the SI.
Physicochemical characterizations of Myr micelles
A dynamic light scattering (DLC) with the Malvern system (Malvern MS2000, UK) was used to determine the micelle size, the polydispersity index (PdI), and the zeta potential of micelles. The micelles used here were diluted with a concentration of Myr (1 mg/ml). Micelle surface morphologies were observed through transmission electron microscopy (TEM, JEM-1200EX, JEOL Ltd., Tokyo, Japan). The Myr entrapment efficiency (EE) in micelles was quantified by the high performance liquid chromatography (HPLC) method as previously reported by Yao et al. (Citation2014).
Differential scanning calorimetry (DSC), infrared (IR) absorption spectrophotometry, and X-ray diffraction (XRD) studies were carried out on free Myr powder, PVCL-PVA-PEG, physical mixtures of free Myr and PVCL-PVA-PEG, and lyophilized Myr micelle optimized formulations. These procedures have previously been described in detail (Li et al., Citation2017).
Stability analysis
Chemical stability of Myr encapsulated in micelles versus free Myr
The chemical stability of Myr was investigated with the chemical degradation trends of Myr under UV–Vis photochemical excitation with timely online monitoring, according to a previously published method, with minor modifications (Leung & Kee, Citation2009; Yang et al., Citation2012). Details of the chemical stability of Myr encapsulated in micelles versus free Myr are described in SI.
Short-term storage stability of Myr micelle ophthalmic solution
To assess its short-term storage stability, the Myr micelle ophthalmic solution was stored at 25 °C and 4 °C for 12 weeks in glass vials, and it was protected from light. Three vials (three samples) were withdrawn at 2-week intervals and analyzed for zeta potential, micelle size, remaining Myr in micelles, and PdI, as described earlier (Verma et al., Citation2013; Sanchez-Lopez et al., Citation2016).
Measurement of antioxidant activity
2,2′-Azinobis (3-ethylbenzothiazoline 6-sulfonate) (ABTS) free-radical scavenging assay
The ABTS assay was explored as previously described (Biskup et al., Citation2013). The final concentrations of Myr used in the ABTS assay were 15.6, 31.3, 62.5, 125, 250, and 500 μg/ml. The corresponding PVCL-PVA-PEG concentrations with a PVCL-PVA-PEG/Myr weight ratio of 18:1 (0.28, 0.56, 1.13, 2.25, 4.50, and 9.00 mg/ml of PVCL-PVA-PEG, respectively) were also tested.
Ferric-reducing antioxidant potential assay
The antioxidant capacity of Myr was also conducted with a ferric-reducing antioxidant potential (FRAP) assay (Jahanshiri et al., Citation2012; Ge et al., Citation2015), with the same formulation information of the tested samples as the ABTS test.
In vitro parallel artificial membrane permeability assay
A parallel artificial membrane permeability assay (PAMPA) was performed with a previously reported method (Feng et al., Citation2008; Bennion et al., Citation2017; Zhang, Polyakov et al., Citation2018), and details of this test information are described in Supplementary Information.
Cell culture tests
In vitro cytotoxicity evaluation
The in vitro cytotoxicity of the Myr micelles was assessed by a methyl thiazolyl tetrazolium (MTT) assay, as previously reported (Guo, Zhang et al., Citation2015; Li et al., Citation2017; Song et al., Citation2018). Both the long-term and short-term cytotoxicities were evaluated. In the long-term incubation experiment, the Myr micelle solution was diluted with a culture medium to 0.78, 1.56, 3.13, 6.25, 12.50, 50.00, 100.00, 200.00, and 400.00 μg/ml and was incubated for 24, 48, and 72 h. In the second experiment, cytotoxicity of the original Myr micelle solution was tested following 1 h incubation (as eye drops are quickly cleared from the ocular surface after instillation, this duration was accepted as a sufficient evaluation of any toxic effects; Di Tommaso et al., Citation2011). The controls consisted of benzalkonium chloride (BAC, 10 and 100 μg/ml).
Cellular uptake test
Cellular uptake of Cou6-labeled Myr micelles was performed using direct visualizing with fluorescence microscope observation and quantitative determination with the flow cytometry method as we previously reported (Song et al., Citation2018). The cellular uptake test details are located in Supplementary Information.
The animal tests
Ocular irritation evaluation
Ocular irritation evaluation was explored in New Zealand albino rabbit eyes with a modified Draize test as previously reported (Guo, Zhang et al., Citation2015; Li et al., Citation2017; Song et al., Citation2018).
Corneal permeation evaluation
The in vivo corneal permeating profiles of Cou6-labeled Myr micelles was revealed in mouse eyes using both visualization with fluorescence microscope observation and quantitative determination with the HPLC method as was previously reported (Song et al., Citation2018). The Cou6-loaded Myr micelles ophthalmic solution group and the free Cou6 solution group—both with 30 mice each—were explored here. Each group was subdivided evenly into three time point groups (10 mice in each time point group) randomly. Both eyes of each mouse were administered 4 eye drops, 5 µl each, 10 min apart. The mice were sacrificed at 30, 60, or 120 min after the last instillation. Then, the corneas were carefully cut off, rinsed with normal saline, and gently blotted with filter paper. Two corneas from one mouse were pooled as one sample. Then, eight of the cornea samples were precisely weighed and homogenized in methanol with a ratio of 0.2 ml of methanol per 1 mg of cornea. The homogenates were further analyzed with HPLC. Another four corneas from two mice of each time group were prepared as frozen sections and observed with the fluorescence microscope. Identical exposure time, brightness, and contrast settings were used to obtain comparative digital images from different samples.
In vivo anti-inflammatory activity
An induced eye inflammation animal model was utilized to explore the anti-inflammatory activity of the Myr micelle ophthalmic solution and evaluate its potential use in ocular inflammation treatment, as previously reported (Li et al., Citation2017). The anti-inflammatory activity of the Myr micelle ophthalmic solution and the free Myr solution were evaluated with diclofenac sodium eye drops (5 ml: 5 mg) as a positive formulation and phosphate buffer (PBS) as a negative formulation.
Data and statistical analysis
The data were expressed as means ± SD. All data were analyzed with SPSS software, version 11.5 (SPSS, Chicago, IL, USA). An independent-samples t test was used to analyze and compare Cou6 in cellular uptake and in vivo cornea permeation tests between the Cou6-labeled Myr micelle groups and the free Cou6 solution groups. A nonparametric analysis of variance (Kruskal–Wallis test) followed by post hoc Mann–Whitney U tests were conducted to compare clinical scores for anti-inflammatory activities among individual groups. Data were considered statistically significant at p < .05.
Results
Preparation and characterization of Myr micelles
Myr could be encapsulated into the micelles as self-assembled from polymer PVCL-PVA-PEG; however, the characterizations, such as EE, micelle size, PdI, and zeta potential, highly depended on the weight ratio of PVCL-PVA-PEG/Myr (Figure S1). When the weight ratio of PVCL-PVA-PEG/Myr was 6:1, only 15.3% of the Myr could be encapsulated into the micelles, but it sharply increased to 99.85% when the weight ratio of PVCL-PVA-PEG/Myr was15:1. When the weight ratio of PVCL-PVA-PEG/Myr was 18:1, the Myr reached the peak of EE, as no increase could be observed. The micelle size and PdI, two parameters of nanoparticles, were also highly dependent on the weight ratio of PVCL-PVA-PEG/Myr. Both the micelle size and PdI decreased when the weight ratio of PVCL-PVA-PEG/Myr increased from 6:1 to 18:1. However, the zeta potential remained constant (∼−2.5 mV) with the weight ratio change of PVCL-PVA-PEG/Myr.
Figure 1. Profiles of Myr micelle ophthalmic solution with an 18:1 PVCL-PVA-PEG/Myr weight ratio. (A) The appearance, (B) morphology of micelles observed with transmission electron microscopy (×50k magnification, bar = 100 nm), (C) micelle size distribution; and (D) zeta potential distribution of the Myr micelle ophthalmic solution. PVCL-PVA-PEG: polyvinyl caprolactam–polyvinyl acetate–polyethylene glycol graft copolymer; Myr: myricetin.
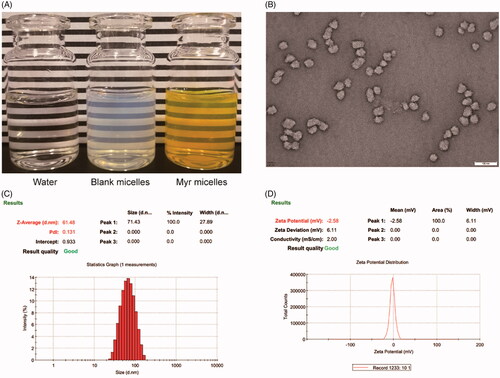
As Myr micelles with an 18:1 weight ratio of PVCL-PVA-PEG/Myr showed high when encapsulated (99.5 ± 0.52%), small micelle size (60.72 ± 1.09 nm), uniform size distribution (PdI = 0.140 ± 0.018), and stable zeta potential (− [2.29 ± 0.33] mV), these Myr micelles were further tested (). This micelle ophthalmic solution with a weight ratio of PVCL-PVA-PEG/Myr 18:1 displayed a transparent, dark yellow appearance (). The viscosity of the PVCL-PVA-PEG micelle ophthalmic solution was 20.53 ± 1.44 mPa s. The micelles that were observed with TEM showed as spheroids with good dispersibility (). The micelle size observed using the TEM was somewhat smaller than that determined by DLS. An explanation for this phenomena might be the micelles shrank during the drying process of the sample preparation for TEM observation.
IR spectroscopy, DSC, and XRD measurements indicated the presence of amorphous Myr in the PVCL-PVA-PEG micelle preparation and also indicated that the PVCL-PVA-PEG could retard Myr crystallization during micelle formation (Figures S2–S4).
Stability evaluation
Chemical stability of Myr encapsulated in micelles versus free Myr
The maximum absorbance of Myr in the free Myr solution and the Myr micelle solution was found to decrease over time, and the pH values of solutions made a significant influence on Myr’s chemical stability (Figure S5). However, there was a big difference between the decreasing profiles of the free Myr solution and in the Myr micelle solution. A representation of the time-dependent spectral changes (absorbance decrease) of Myr is presented in Figure S5(A), and the decays of the absorbance at 375 nm due to the degradation of free Myr or Myr micelles with different pH levels of PBS are presented in Figure S5(B). For the free Myr solution, the spectra of Myr in the aqueous medium have a single peak (∼ 375 nm). This peak decreased slowly over time in an acidic environment, such as pH 6.0; in this test, the t1/2 was 3.79 h. The t1/2 values decreased slowly as the pH values increased from 6.0 to 6.8, but dramatic decreases could be observed at pH 7.0 and 7.4. Especially for the free Myr pH 7.4 solution, the peak (∼375 nm) rapidly decreased under the scanning and excitation of UV light—the peak (∼375 nm) diminished and a new single peak (∼427 nm) was produced, where the absorption at maximum decreases to roughly 28.6% of the original value in 1 h, with the t1/2 0.55 h (Figure S1). These results indicated that free Myr was not chemically stable in aqueous solutions, especially in neutral and alkaline aqueous solutions.
A decrease in the absorbance of Myr in the Myr micelle solution was also found to decrease over time, but the decrease was much slower when compared to that of the same pH value of the free Myr solution. The t1/2 of Myr in the Myr micelle solution was 22.94-, 22.85-, 23.20-, and 23.82-fold higher than that of Myr in the Myr micelle solution with pH values of 6.0, 6.2, 6.5, and 6.8, respectively (Table S1). The Myr in Myr micelle solution showed greater stability than the free Myr solution in the neutral and alkaline aqueous solutions. The t1/2 of the Myr in the Myr micelle solution was 32.91- and 60.25-fold higher than that of Myr in the free Myr solution, with pH values of 7.0 and 7.4, respectively (Table S1). These results clearly highlighted the intrinsic ability of micelle encapsulation to significantly improve Myr’s chemical stability, especially in neutral and alkaline aqueous solutions.
Short-term storage stability of Myr micelle ophthalmic solution
The Myr micelle ophthalmic solution exhibited excellent storage stability. After 12 weeks of storage at 4 °C and 25 °C, the appearance of the Myr micelle ophthalmic solution was unaltered. The micelle size, PdI, and zeta potential of the Myr micelles exhibited no big changes with the initial value (Figure S6). The initial value of the Myr in micelles (4.0 mg/ml) was set as 100.0 ± 0.0%. The Myr remaining in the micelles during the storage was 98.15 ± 1.96%, and 92.96 ± 1.83% after 12 weeks of storage at 4 °C and 25 °C, respectively. Therefore, Myr micelle ophthalmic solution is suitable to store at 4 °C condition.
Antioxidant activity
The antioxidant activities of Myr in the micelles were quantified via both ABTS and FRAP assays and were then compared to those of the free Myr solution in ethanol. These were prepared at the same concentration, incubation time, and under the same experiment conditions (incubation in the dark). Data are displayed in Figure S7. Regarding the ABTS analysis, the antioxidant activities increased with the increasing concentration/incubation time in both the encapsulated Myr in micelles and the free Myr solution. However, for the antioxidant activities of detail concentration and incubation time, the difference in antioxidant activity could be observed between the encapsulated Myr in micelles and the free Myr solution. For example, the antioxidant activity of 15.6 μg/ml Myr in micelles reached 20.2% only with 15 min of incubation, and increased to 44.8% with 120 min incubation. However, the antioxidant activity in the free Myr solution was much lower. For the 15.6 μg/ml of free Myr solution, no antioxidant activity was observed with 15 min, and just 6.1% was observed after 120 min of incubation. Regarding the FRAP evaluation, the result was similar to that received with the ABTS evaluation: unlike the free Myr solution, an improved antioxidant activity could be observed to encapsulate Myr in micelles.
Cell culture tests
In vitro cytotoxicity test
The short-term and long-term in vitro cytotoxic effects of Myr micelles in Human corneal epithelial cells (HCECs; ATCC CRL-11135) were presented in . In the long-term cytotoxic evaluation, the survival rate of HCECs decreased with the increasing concentration/incubation time of Myr micelles. A high cell viability (>90%) was observed with the concentration ≤100 μg/ml after 24 h, ≤12.5 μg/ml after 48 h, and ≤6.25 μg/ml after 72 h of incubation. Though some cytotoxicities were observed at the highest concentration of 400 μg/ml of Myr micelle solution, the cell viabilities were still significantly higher than those of 10 μg/ml BAC. The BAC was widely used with concentration of 100 μg/ml in many commercial eye drops. Therefore, these results revealed that the Myr micelles ophthalmic solution exhibited good cell tolerance in long-term incubation. The results from short-term incubation also confirmed that Myr micelles ophthalmic solution exhibited good cell tolerance, as no cytotoxicity was observed in either the Myr micelles ophthalmic solution or the free Myr solution with 4 mg/ml of Myr. However, the 0.1 mg/ml BAC was observed with only 39.5 ± 4.0% cell viability, and even 0.01 mg/ml BAC was observed with just 84.7 ± 5.3% cell viability. Therefore, Myr micelles ophthalmic solution with 4 mg/ml of Myr should be a safe level for its in vivo application.
Figure 2. Cytotoxicity evaluation with MTT assay (n = 3). HCECs (ATCC CRL-11135) cultivated for (A) 24 h, (B) 48 h, and (C) 72 h with a series of Myr micelle ophthalmic solution; (D) HCECs incubated with Myr micelle ophthalmic solution for 1 h and with BAC as a reference. Absorbances were normalized to the untreated control cultures, which represented 100% viability. Myr: myricetin; BAC: benzalkonium chloride.
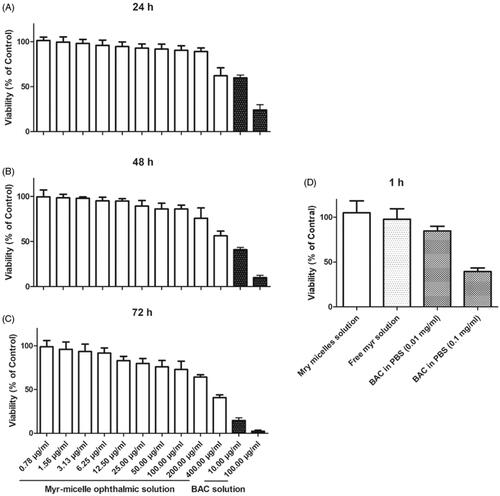
Cellular uptake test
The cellular uptake characteristics of Cou6-labeled Myr micelles were evaluated as part of an in vitro corneal permeating test. As shown in , the green fluorescence of Cou6 was dramatically increased over incubation time from 5 to 90 min, confirming that the cellular uptake of Cou6-labeled Myr micelles by HCECs was incubation time dependent. Comparatively, the fluorescence intensity of the cells incubated with the free Cou6 solution was much weaker than that of the Cou6-labeled Myr micelles.
Figure 3. Cellular uptake characteristics of the Myr micelle ophthalmic solution. (A) Uptake characteristics of the Cou6-labeled Myr micelle or free Cou6 into HCECs was observed with fluorescence microscope (bar = 10 μm). (B) Uptake of Cou6-labeled Myr micelles or free Cou6 into HCECs was quantitatively determined with flow cytometry.
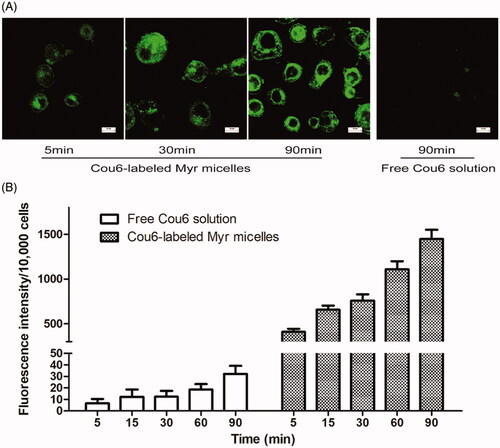
By quantifying the cellular uptake profile of Cou6-labeled Myr micelles, the internalized fluorescence intensity within the cultured HCECs was determined by flow cytometer (). These results confirmed that the cellular uptake of the Cou6-labeled Myr micelles and the free Cou6 by HCECs was incubation time dependent. The analyses showed dramatic increasing fluorescence intensity in HCECs for Cou6-labeled Myr micelles compared to the free cou6 solution, which was in accordance with the fluorescence microscope observation. For the Cou6-labeled Myr micelles, the fluorescence intensity was up to 60.2-, 53.0-, 60.5-, 58.5-, and 43.9-fold stronger than that of the free Cou6 solution after 5, 15, 30, 60, and 90 min, respectively (compared to the free Cou6 solution group p < .05). These results indicated that the Cou6-labeled Myr micelles had the potential to deliver more Myr into the cornea; this may be attributed to the fact that PVCL-PVA-PEG encapsulation could highly improve the solubility and stability of Myr, with only a nanometer of micelles improving the cellular uptake of these micelles.
The animal tests
Ocular irritation studies
After 13 ocular instillations of the Myr micelles ophthalmic solution in rabbit eyes, a notable ocular tolerance was observed, and no tear shedding or irritation were found during the entire test. No damage was observed in the conjunctiva, cornea, or iris (). The average total scores according to the Draize test were lower than 2 throughout the whole test. The fluorescent staining observation revealed that no corneal lesions were found throughout the experiment. In addition, the histopathology evaluation revealed that the Myr micelles ophthalmic solution-treated cornea exhibited an intact epithelial layer with no stromal swelling (corneal edema). Also, inflammatory cell infiltration was not observed in the whole cornea tissue. The BAC group (0.01% BAC was widely used in commercial eye drops) also showed no significant differences in the appearance of the various tissues compared with the control tissue (the PBS group).
Figure 4. Ocular tolerance observation of the Myr micelle ophthalmic solution. (A) Representative slit-lamp biomicroscopic images of rabbit eyes 24 h after topical instillations. (B) Representative histopathologic images of rabbit corneas 24 h after topical instillations. Myr: myricetin; BAC: benzalkonium chloride. Bar = 100 μm.
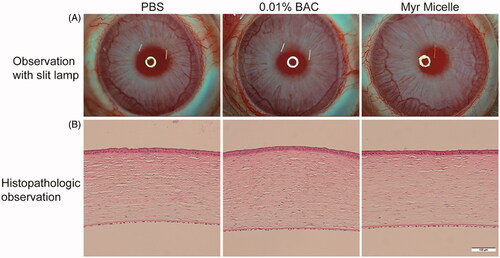
Corneal permeation studies in mouse eyes
Quantitative corneal permeation results are shown in . The Cou6 concentrations in mouse corneas treated with the Cou6-labeled Myr micelle ophthalmic solution were significantly increased and were 4.36-, 2.54-, and 2.54-fold higher when compared with those for the free Cou6 solution at the 30, 60, and 120 min time points, respectively.
Figure 5. Corneal permeation results in mouse eyes. (A) Cou6 concentration in corneas after administration with 4 eye drops, 5 µl each, 10 min apart. (*compared to the free Coumarin-6 [Cou6] p<.05, n = 8). (B) Fluorescence microscope observation of cornea sections, bar = 10 μm.
![Figure 5. Corneal permeation results in mouse eyes. (A) Cou6 concentration in corneas after administration with 4 eye drops, 5 µl each, 10 min apart. (*compared to the free Coumarin-6 [Cou6] p<.05, n = 8). (B) Fluorescence microscope observation of cornea sections, bar = 10 μm.](/cms/asset/04089402-9586-4225-8e99-ce9517be5310/idrd_a_1622608_f0005_c.jpg)
In , visualizing results of the corneal permeation are shown. The fluorescence intensity was much stronger in the corneal epithelial layer at 30 min after instillation of the Cou6-labeled Myr micelle ophthalmic solution. The fluorescence intensity in the stroma and endothelium and the deep layers of the cornea was considerably weaker compared to the corneal epithelium. The fluorescence intensity in the whole cornea (epithelium, stroma, and endothelium) decreased as time increased. However, the fluorescence intensity of the free Cou6 solution group remained dramatically weaker compared with those from the Cou6-labeled Myr micelle ophthalmic solution group. No fluorescence could be observed at 1 and 2 h time points after instillation of the free Cou6 solution.
In vivo anti-inflammatory prevention efficacy
The anti-inflammatory efficacies of the Myr micelle ophthalmic solution and the free Myr solution versus diclofenac sodium eye drops as positive and PBS as negative controls is shown in . Diclofenac sodium eye drops showed significantly high anti-inflammatory efficacy during the whole experiment (compared to the PBS group p < .05), but no significant anti-inflammatory efficacy was observed in the 4 mg/ml of free Myr solution throughout the experiment (compared to the PBS group p > .05). The Myr micelle ophthalmic solution exhibited dosage-dependent anti-inflammatory efficacy. For the Myr micelle ophthalmic solution with 4 mg/ml of Myr, significant high anti-inflammatory efficacies were observed throughout the experiment, and these efficacies were comparable with those from diclofenac sodium eye drops (compared to the diclofenac sodium eye drops group p > .05). While the Myr micelle ophthalmic solution with 1.3 mg/ml of Myr showed just partial anti-inflammatory efficacy in the experiment (compared to the PBS group at 120, 180, 240, and 360 min time points p < .05), and no significant anti-inflammatory efficacy was observed in the 0.4 mg/ml Myr micelle ophthalmic solution throughout the experiment (compared to the PBS group p > .05).
Figure 6. Anti-inflammatory efficacy of Myr micelles and free Myr on the inflammation induced by sodium arachidonate solution (SAS) in rabbit eyes compared with diclofenac sodium eye drops (5 ml: 5 mg) and PBS (mean ± SD, n = 6, *p<.05 compared to the PBS group, $p<.05 compared to the 4 mg/ml of free Myr solution group, #p<.05 compared to the diclofenac sodium eye drops group, &p<.05 compared to the 4 mg/ml of Myr micelles group). Myr: myricetin; DIC: diclofenac sodium eye drops.
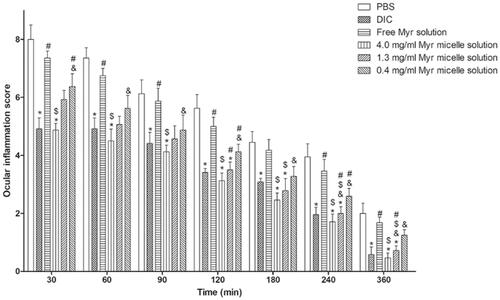
Discussion
Despite offering various pharmacological effects to the field of ophthalmology, the ocular delivery of Myr remains a challenge due to its insolubility and instability in water, which limits its applications. Micellar solubilization had been used for many years for solubility, stability, and bioavailability enhancement of those poorly soluble and unstable drugs. Despite wide research and knowledge on micelles, especially polymeric micelles as ocular drug delivery systems, no approved formulation is available in the market to date. One of the main reasons for this is these micelles formulations’ are very low water stability hinders its fabrication into aqueous eye drops with lengthy storage, and it usually requires freeze-drying to keep its storage stability (Gupta et al., Citation2000; Kanoujia et al., Citation2014; Yu, Chen et al., Citation2018). A redissolving procedure was needed for these freeze-drying formulations before use; this was not only inconvenient but also a challenge to these sterile preparations. The other main reason was the micelles formulations were highly dependent on the nanomaterials. Though many researchers explored ocular delivery with micelle formulation, most of their explored nanomaterials were not authorized pharmaceutical excipients, and the ocular biocompatibility of these materials has been the subject of debate (Song et al., Citation2018). An ultra-small nanocarrier based on polyoxyl 15 hydroxystearate (Kolliphor® HS15, HS15) micelles was explored to evaluate the formulation potential of Myr to ocular topical administration in previous report (Hou et al., Citation2019). Though the in vivo ocular tolerance tests revealed that the micelle formulations based on HS15 showed excellent ocular tolerance (Zhou et al., Citation2017; Hou et al., Citation2019), the ocular safety concerns of HS15 and its formulation should be kept in mind, and a recent publication also hinted the safety concern of this nonionic surfactant HS15 used in drug delivery formulations (Cavanagh et al., Citation2019).
To address these problems, micelles based on PVCL-PVA-PEG were developed in this study to enhance the aqueous solubility and stability of Myr to fabricate the ophthalmic solution. PVCL-PVA-PEG is an approved excipient that enables new solving approaches for solubility and bioavailability for poorly soluble drugs (Varela-Garcia et al., Citation2018). Recently, it was exploited as a nanocarrier based on the self-assembling micelle characterization of this amphiphilic polymer, and this micelle was explored to ocular delivery based on its excellent ocular biocompatibility (Guo, Zhang et al., Citation2015, Guo et al., Citation2016; Li et al., Citation2017, Citation2018; Varela-Garcia et al., Citation2018). Myr could be nearly completely encapsulated into the PVCL-PVA-PEG micelles with an optimized PVCL-PVA-PEG/Myr weight ratio. A PVCL-PVA-PEG micelle ophthalmic solution of 4 mg/ml of Myr was explored in this study, and this aqueous solubility was 261.6-fold higher than its free aqueous solubility (15.23 μg/ml in PBS with pH 6.5, unpublished data). The storage stability of the Myr micelle ophthalmic solution was exciting. The Myr remaining in the micelles was as high as 98.15 ± 1.96% after 12 weeks of storage at 4 °C, and this storage profile was similar with our previous reports (Li et al., Citation2017). Long-term storage stability is still under investigation. The low CMC of PVCL-PVA-PEG contributes to the high encapsulation and high storage stability.
It was reported that the pH was the strongest influence of the stability of Myr in an aqueous solution, and Myr underwent rapid apparent first-order degradation under basic pH conditions (Franklin & Myrdal, Citation2015; Xiang et al., Citation2017). Considering the accepted pH values of eye drops, a pH range of 6.0–7.4 was tested in the chemical stability of Myr in an aqueous solution to optimize the suitable pH value to this Myr micelle ophthalmic solution. The free Myr underwent rapid pH dependent degradation in this test, especially to a solution with pH 7.4 (t1/2=0.55 h). The PVCL-PVA-PEG micelle encapsulation significantly improved the stability of the Myr, and it displayed a more retarded ability, especially to a solution with pH 7.4 (with t1/2 61.25-fold higher than the free Myr). The pH 6.5 level was used to formulate the Myr micelle ophthalmic solution in this study, because there was no ocular irritation and Myr has a relative chemical stability in an aqueous solution at this pH level. The great improvement of Myr’s chemical stability in PVCL-PVA-PEG micelle could be due to the protection effect of the inner hydrophobic core of the micelles against the aqueous environment. It was interesting to note that PVCL-PVA-PEG micelles displayed higher potential of improving of Myr’s chemical stability than that potential from HS15 micelles encapsulation reported. (The t1/2 of Myr in the PVCL-PVA-PEG micelle solution was 2.61-, 3.93-, 7.48-, 19.20-, 34.28-, and 36.43-fold higher than that of Myr in the HS15 micelle solution with pH values of 6.0, 6.2, 6.5, 6.8, 7.0, and 7.4, respectively. Data were calculated from this article and our previous report (Hou et al., Citation2019).) The ultra-low CMC of PVCL-PVA-PEG might contribute this high potential of improvement of Myr’s chemical stability (the CMC of PVCL-PVA-PEG was 3.68 × 10−8 M, and HS15 was 1.16 × 10−3 M in artificial tears; Guo, Zhang et al., Citation2015; Hou et al., Citation2019). Low CMC of the formulation is desired to prevent the disruption of micelles by factors of environment including pH value.
Although Myr has various pharmacological applications, the antioxidative activity is the most important characteristic, and many pharmacological activities such as anti-inflammatory and neuroprotective activity are derived from the antioxidant activity. So, the in vitro antioxidant activities were evaluated in this study using ABTS and FRAP assays, two widely used in vitro antioxidant activity evaluations (Biskup et al., Citation2013). Both these evaluations confirmed that the in vitro antioxidant activity was improved in the Myr after encapsulation in micelles. The greatly improved solubility and chemical stability may be important factors contributing to its antioxidant activity improvement.
It was reported in several publications that PVCL-PVA-PEG had notable in vitro cellular and in vivo ocular tolerance in its ocular topical use (Guo, Zhang et al., Citation2015; Li et al., Citation2017). To our best knowledge, this article was the first report to develop Myr as an ophthalmic formulation. Therefore, the ocular safety of this formulation must be adequately revealed. Both in vitro and in vivo tests to evaluate the toxicity of the Myr micelle formulation were performed in this article, and the results were promising. Both the long-term incubation and short-term incubation confirmed that the Myr micelle ophthalmic solution exhibited good cell tolerance, as no cytotoxicity was observed in either the Myr micelles ophthalmic solution or the free Myr solution with 4 mg/ml of the Myr. The in vivo ocular irritation studies also confirmed that the Myr micelle ophthalmic solution had good ocular tolerance. BAC is widely used as a preservative in eye drops at a concentration of 0.05–0.1 mg/ml. It was widely reported that the BAC displayed significant in vitro cytotoxicity and in vivo ocular surface toxicity/irritation (Uematsu et al., Citation2011; Onizuka et al., Citation2014). The cytotoxicity evaluation also confirmed that the BAC displayed high cytotoxicity. However, no obvious irritation was observed in the BAC group rabbit eye test, and this result seemed to disagree with those reported (Uematsu et al., Citation2011; Onizuka et al., Citation2014). One explanation might be that healthy rabbits without any eye diseases were used in this test, and the healthy eyes showed good tolerance during the testing.
The improved solubility and chemical stability, and the small micelle size characteristic of the PVCL-PVA-PEG micelles may benefit in vitro/in vivo corneal permeation. Membrane permeation was 6.70-fold higher for the Myr micelle ophthalmic solution than for the free Myr after 3.5 h of membrane permeation (Figure S8), as determined by in vitro PAMPA, a model specifically designed to measure passive membrane permeability. The cellular uptake was greatly improved in the Myr micelles than in the free Myr. The in vivo corneal permeation test also confirmed a greater improvement in the Myr micelles than in the free Myr. It was also interesting to conclude that PVCL-PVA-PEG micelle displayed higher potential of improving of Myr’s in vivo corneal permeation than that potential from HS15 micelles encapsulation reported. (The in vivo corneal permeation of Cou6-labeled Myr PVCL-PVA-PEG micelle ophthalmic solution was 2.56-, 0.78-, and 1.11-fold higher than that of Cou6-labeled Myr HS15 micelle ophthalmic solution at the 30, 60, and 120 min time points, respectively. Data were calculated from this manuscript and our previous report (Hou et al., Citation2019).) And these results seemed some contradictory to the existing knowledge, as generally speaking, smaller particles are able to more efficiently enter a cell or tissue (the micelle size was about 60 nm to Myr PVCL-PVA-PEG micelles and about 12 nm to Myr HS15 micelles; Hou et al., Citation2019). While the Myr PVCL-PVA-PEG micelle ophthalmic solution had higher viscosities than that of Myr HS15 micelle ophthalmic solution (the viscosity of Myr HS15 micelle ophthalmic solution was 5.43 ± 0.92 mPa s, unpublished data), it might improve the in vivo corneal permeation profiles.
The greatly improved solubility and chemical stability, and the significant improvement of antioxidative activity and corneal permeation of the Myr micelles might be reflected in its pharmacological activities. An acute inflammatory animal model induced with ocular topical SAS was used here to evaluate the anti-inflammatory activity of the Myr micelle ophthalmic solution. Dosage-related anti-inflammatory efficacies of Myr micelle ophthalmic solutions were observed. The micelles with 4 mg/ml of Myr reflected a comparably effective anti-inflammatory effect with diclofenac sodium eye drops (a commonly used nonsteroidal anti-inflammatory eye drop used in ophthalmology with low risks of the severe side effects; Yu, Zhang et al., Citation2018), while the free Myr displayed no efficacy during the whole test. It was worth to mention that both PVCL-PVA-PEG micelle ophthalmic solution Myr 4 mg/ml, and the HS15 micelles with Myr 10 mg/ml reflected a comparable effective anti-inflammatory effect with diclofenac sodium eye drops. These indicated that a high anti-inflammatory effect could be obtained from Myr PVCL-PVA-PEG micelle.
Conclusion
A PVCL-PVA-PEG micelle formulation was explored to enhance the aqueous solubility, stability, corneal permeation, and ocular anti-inflammatory efficacy of Myr. Myr could be highly encapsulated into micelles, and this Myr micelle ophthalmic solution had no significant cytotoxicity and displayed notable in vivo ocular tolerance. PVCL-PVA-PEG micelles significantly enhanced the aqueous solubility and stability of Myr, making it sufficiently flexible for formulation as Myr eye drops. Myr micelles also greatly improved their in vitro cellular uptake and in vivo corneal permeation as well as in vitro antioxidant and in vivo anti-inflammatory activity. Thus, this research demonstrated that Myr micelles are a promising ocular formulation.
Supporting_Information-Revised.docx
Download MS Word (3.6 MB)Disclosure statement
The authors report no conflicts of interest in this work.
Additional information
Funding
References
- Bennion BJ, Be NA, McNerney MW, et al. (2017). Predicting a drug's membrane permeability: a computational model validated with in vitro permeability assay data. J Phys Chem B 121:5228–37.
- Biskup I, Golonka I, Gamian A, et al. (2013). Antioxidant activity of selected phenols estimated by ABTS and FRAP methods. Postepy Hig Med Dosw 67:958–63.
- Cavanagh RJ, Smith PA, Stolnik S. (2019). Exposure to a nonionic surfactant induces a response akin to heat-shock apoptosis in intestinal epithelial cells: implications for excipients safety. Mol Pharmaceutics 16:618–31.
- Chen R, Hollborn M, Grosche A, et al. (2014). Effects of the vegetable polyphenols epigallocatechin-3-gallate, luteolin, apigenin, myricetin, quercetin, and cyanidin in primary cultures of human retinal pigment epithelial cells. Mol Vis 20:242–58.
- Di Tommaso C, Torriglia A, Furrer P, et al. (2011). Ocular biocompatibility of novel cyclosporin A formulations based on methoxy poly(ethylene glycol)-hexylsubstituted poly(lactide) micelle carriers. Int J Pharm 416:515–24.
- Feng B, Mills JB, Davidson RE, et al. (2008). In vitro P-glycoprotein assays to predict the in vivo interactions of P-glycoprotein with drugs in the central nervous system. Drug Metab Dispos 36:268–75.
- Franklin SJ, Myrdal PB. (2015). Solid-state and solution characterization of myricetin. AAPS PharmSciTech 16:1400–8.
- Ge W, Li D, Chen M, et al. (2015). Characterization and antioxidant activity of beta-carotene loaded chitosan-graft-poly(lactide) nanomicelles. Carbohydr Polym 117:169–76.
- Guo C, Cui F, Li M, et al. (2015). Enhanced corneal permeation of coumarin-6 using nanoliposomes containing dipotassium glycyrrhizinate: in vitro mechanism and in vivo permeation evaluation. RSC Adv 5:75636–47.
- Guo C, Zhang Y, Yang Z, et al. (2015). Nanomicelle formulation for topical delivery of cyclosporine A into the cornea: in vitro mechanism and in vivo permeation evaluation. Sci Rep 5:12968.
- Guo C, Li M, Qi X, et al. (2016). Intranasal delivery of nanomicelle curcumin promotes corneal epithelial wound healing in streptozotocin-induced diabetic mice. Sci Rep 6:29753.
- Gupta AK, Madan S, Majumdar DK, et al. (2000). Ketorolac entrapped in polymeric micelles: preparation, characterisation and ocular anti-inflammatory studies. Int J Pharm 209:1–14.
- Hodges LC, Kearse CE, Green K. (1999). Intraocular pressure-lowering activity of phenolic antioxidants in normotensive rabbits. Curr Eye Res 19:234–40.
- Hou W, Hu S, Su Z, et al. (2018). Myricetin attenuates LPS-induced inflammation in RAW 264.7 macrophages and mouse models. Future Med Chem 10:2253–64.
- Hou Y, Zhang F, Lan J, et al. (2019). Ultra-small micelles based on polyoxyl 15 hydroxystearate for ocular delivery of myricetin: optimization, in vitro, and in vivo evaluation. Drug Deliv 26:158–67.
- Hu M, Zhang J, Ding R, et al. (2017). Improved oral bioavailability and therapeutic efficacy of dabigatran etexilate via Soluplus-TPGS binary mixed micelles system. Drug Dev Ind Pharm 43:687–97.
- Jahanshiri Z, Shams-Ghahfarokhi M, Allameh A, et al. (2012). Effect of curcumin on Aspergillus parasiticus growth and expression of major genes involved in the early and late stages of aflatoxin biosynthesis. Iran J Public Health 41:72–9.
- Ju RJ, Mu LM, Li XT, et al. (2018). Development of functional docetaxel nanomicelles for treatment of brain glioma. Artif Cells Nanomed Biotechnol 46:1180–90.
- Kanoujia J, Kushwaha PS, Saraf SA. (2014). Evaluation of gatifloxacin pluronic micelles and development of its formulation for ocular delivery. Drug Deliv Transl Res 4:334–43.
- Laabich A, Manmoto CC, Kuksa V, et al. (2007). Protective effects of myricetin and related flavonols against A2E and light mediated-cell death in bovine retinal primary cell culture. Exp Eye Res 85:154–65.
- Leung MH, Kee TW. (2009). Effective stabilization of curcumin by association to plasma proteins: human serum albumin and fibrinogen. Langmuir 25:5773–7.
- Li M, Xin M, Guo C, et al. (2017). New nanomicelle curcumin formulation for ocular delivery: improved stability, solubility, and ocular anti-inflammatory treatment. Drug Dev Ind Pharm 43:1846–57.
- Li M, Xin M, Song K, et al. (2018). Evaluation of polyvinyl caprolactam-polyvinyl acetate-polyethylene glycol graft copolymer nanomicelle for trigeminal ganglion neurons delivering with intranasal administration. Curr Eye Res 43:406–14.
- Mandal A, Bisht R, Rupenthal ID, et al. (2017). Polymeric micelles for ocular drug delivery: from structural frameworks to recent preclinical studies. J Control Rel 248:96–116.
- Onizuka N, Uematsu M, Kusano M, et al. (2014). Influence of different additives and their concentrations on corneal toxicity and antimicrobial effect of benzalkonium chloride. Cornea 33:521–6.
- Qian J, Meng H, Xin L, et al. (2017). Self-nanoemulsifying drug delivery systems of myricetin: formulation development, characterization, and in vitro and in vivo evaluation. Colloids Surf B Biointerfaces 160:101–9.
- Sanchez-Lopez E, Egea MA, Cano A, et al. (2016). PEGylated PLGA nanospheres optimized by design of experiments for ocular administration of dexibuprofen-in vitro, ex vivo and in vivo characterization. Colloids Surf B Biointerfaces 145:241–50.
- Saydam M, Cheng WP, Palmer N, et al. (2017). Nano-sized soluplus(R) polymeric micelles enhance the induction of tetanus toxin neutralising antibody response following transcutaneous immunisation with tetanus toxoid. Vaccine 35:2489–95.
- Semwal D, Semwal R, Combrinck S, et al. (2016). Myricetin: a dietary molecule with diverse biological activities. Nutrients 8:90.
- Song K, Xin M, Yu H, et al. (2018). Novel ultra-small micelles based on rebaudioside A: a potential nanoplatform for ocular drug delivery. Int J Pharm 552:265–76.
- Tang X-J, Huang K-M, Gui H, et al. (2016). Pluronic-based micelle encapsulation potentiates myricetin-induced cytotoxicity in human glioblastoma cells. Int J Nanomedicine 11:4991–5002.
- Uematsu M, Kumagami T, Shimoda K, et al. (2011). Polyoxyethylene hydrogenated castor oil modulates benzalkonium chloride toxicity: comparison of acute corneal barrier dysfunction induced by travoprost Z and travoprost. J Ocul Pharmacol Ther 27:437–44.
- Varela-Garcia A, Concheiro A, Alvarez-Lorenzo C. (2018). Soluplus micelles for acyclovir ocular delivery: formulation and cornea and sclera permeability. Int J Pharm 552:39–47.
- Verma P, Gupta RN, Jha AK, et al. (2013). Development, in vitro and in vivo characterization of Eudragit RL 100 nanoparticles for improved ocular bioavailability of acetazolamide. Drug Deliv 20:269–76.
- Wang L-L, He D-D, Wang S-X, et al. (2018). Preparation and evaluation of curcumin-loaded self-assembled micelles. Drug Dev Ind Pharm 44:563–9.
- Xiang D, Wang C-g, Wang W-q, et al. (2017). Gastrointestinal stability of dihydromyricetin, myricetin, and myricitrin: an in vitro investigation. Int J Food Sci Nutr 68:704–11.
- Yang Q, Li Y, Luo L. (2018). Effect of myricetin on primary open-angle glaucoma. Transl Neurosci 9:132–41.
- Yang R, Zhang S, Kong D, et al. (2012). Biodegradable polymer-curcumin conjugate micelles enhance the loading and delivery of low-potency curcumin. Pharm Res 29:3512–25.
- Yao Y, Lin G, Xie Y, et al. (2014). Preformulation studies of myricetin: a natural antioxidant flavonoid. Pharmazie 69:19–26.
- Yin Y, Zong R, Bao X, et al. (2018). Oxidative stress suppresses cellular autophagy in corneal epithelium. Invest Ophthalmol Vis Sci 59:3286–93.
- Yu X, Zhang Z, Yu J, et al. (2018). Self-assembly of a ibuprofen-peptide conjugate to suppress ocular inflammation. Nanomedicine 14:185–93.
- Yu Y, Chen D, Li Y, et al. (2018). Improving the topical ocular pharmacokinetics of lyophilized cyclosporine A-loaded micelles: formulation, in vitro and in vivo studies. Drug Deliv 25:888–99.
- Zeng Y-C, Li S, Liu C, et al. (2017). Soluplus micelles for improving the oral bioavailability of scopoletin and their hypouricemic effect in vivo. Acta Pharmacol Sin 38:424–33.
- Zhang N, Feng H, Liao H-H, et al. (2018). Myricetin attenuated LPS induced cardiac injury in vivo and in vitro. Phytother Res 32:459–70.
- Zhang Q, Polyakov NE, Chistyachenko YS, et al. (2018). Preparation of curcumin self-micelle solid dispersion with enhanced bioavailability and cytotoxic activity by mechanochemistry. Drug Deliv 25:198–209.
- Zhou T, Zhu L, Xia H, et al. (2017). Micelle carriers based on macrogol 15 hydroxystearate for ocular delivery of terbinafine hydrochloride: in vitro characterization and in vivo permeation. Eur J Pharm Sci 109:288–96.