Abstract
Osteoarthritis (OA) is a progressive and degenerative disease, which is no longer confined to the elderly. So far, current treatments are limited to symptom relief, and no valid OA disease-modifying drugs are available. Additionally, OA relative joint is challenging for drug delivery, since the drugs experience rapid clearance in joint, showing a poor bioavailability. Existing therapeutic drugs, like non-steroidal anti-inflammatory drugs (NSAIDs) and corticosteroids, are not conducive for long-term use due to adverse effects. Though supplementations, including chondroitin sulfate and glucosamine, have shown beneficial effects on joint tissues in OA, their therapeutic use is still debatable. New emerging agents, like Kartogenin (KGN) and Interleukin-1 receptor antagonist (IL-1 ra), without a proper formulation, still will not work. Therefore, it is urgent to establish a suitable and efficient drug delivery system for OA therapy. In this review, we pay attention to various types of drug delivery systems and potential therapeutic drugs that may escalate OA treatments.
1. Introduction
Osteoarthritis (OA), known as a progressive and degenerative disease, constantly impacts the lives of a considerable proportion of adults globally. Osteoarthritis patients have a higher risk of death than the general population (Nüesch et al. Citation2011). Researchers have found that almost 27 million people in the US suffered from OA (Lawrence et al. Citation2008). It was estimated that an overall crude incidence for the total Alberta population was 1040 per 100,000 person-years (Sun et al. Citation2007). Many studies have already investigated that the incidence of OA is related to gender and age. As a result of population aging, the rates in both men and women increased, while women may have much more possibilities to have joints problems. At the same time, women aged beyond 50 but less than 60 years old have the highest incidence (Lawrence et al. Citation2008). With its high prevalence, OA has put tremendous pressure both on individuals and the social economic. Over 28 years, direct medical costs on OA were $129,600 in US (Losina et al. Citation2015).
Generally, treatments of osteoarthritis can be categorized into three aspects: non-pharmacological treatments, pharmacological treatments, and surgical treatments (). Non-pharmacological treatments include patient education recommended by the European League Against Rheumatism (EULAR), diet (as obesity takes an important role in the pathology of OA), and notably emphasis on exercise (Rannou & Poiraudeau Citation2010). Others involve electrotherapy/neuromuscular electrical stimulation, Spa therapy, strength training, etc. (McAlindon et al. Citation2014). All of these are effective; however, with extended periods, many patients fail to fulfill the therapies. The most common techniques used in the clinical for cartilage defects and degeneration are repaired by marrow stimulation arthroscopic debridement and articular cartilage replacement procedures (Simon & Jackson Citation2018). These procedures are high technical requirements and depend on whether the cartilage is healthy or not.
Figure 1. Introduction of OA. (A) Current OA treatment, including surgery, drugs, diet, patient education, and exercise. (B) Normal parasagittal section of the knee. (C) Schematic illustration of osteoarthritis. The pathobiology of OA and potential occurring sites in human body. Compared to (B), it demonstrates that OA is a disease that affects the entire joint structure, including the articular cartilage, synovium, subchondral bone, joint capsule, and other components of the joint.
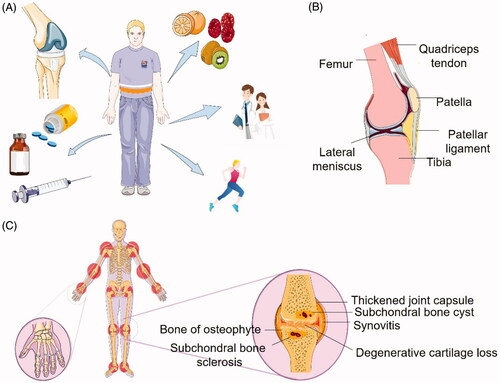
As for pharmacological treatments, there are various agents for clinicians to select, such as selective cyclooxygenase (COX)-2 inhibitors, non-steroidal anti-inflammatory drugs (NSAIDs), corticosteroids, even analgesics. However, oral administration of NSAIDs may cause a lot of side effects, like upper gastrointestinal (GI) complications, and cardiovascular (CV) (Pelletier et al. Citation2016). Unfortunately, a study has already shown that topical NSAIDs are not only inferior to oral NSAIDs during the first week of therapy but also arises more local side effects (Lin et al. Citation2004). What’s more, there was no significant difference between topical NSAIDs and placebo of efficacy after two weeks. As for glucocorticoids, one of the most widely recommended therapeutic agents for knee OA (Bannuru et al. Citation2019); clinical trials have indicated that their analgesic effect goes down within 2–4 weeks (Glyn-Jones et al. Citation2015). Supplementations and new agents like chondroitin, glucosamine, hyaluronic acid (HA), and Kartogenin (KGN) emerged; they play different roles in OA treatments. KGN can induce mesenchymal stem cells (MSCs) into chondrocytes (Johnson et al. Citation2012; Fazal-Ur-Rehman et al. Citation2016). It has been demonstrated that HA has chondroprotective effects, maintains the rheological homoeostasis of the synovial fluid and so on (Bishnoi et al. Citation2014). Except for the disadvantages of traditional treatments, another challenge is from the synovial joint itself. As a barrier, synovial joints play a key role in delivering therapies to the target sites. Meanwhile, therapeutic agents would be rapidly cleared due to synovial fluids exchange. As a result, when adopting intra-articular injection (IA), it needs frequent injection which causes financial burdens, leads to alteration and damage of the cartilage, and causes more pain to patients with less and less compliance. Apparently, with the need for curing OA increasing rapidly, it facilitates researchers to discover better therapeutics and new drug delivery systems.
In this review, we focus on different types of delivery systems that have been proposed or have been examined to date to treat OA. Their advantages and innovations compared to traditional formulations are the main considerations. Alternatively, potential agents delivered by novel carriers performed better in suppressing or deterring the progression of OA will also be discussed here. Hoping our review will bring new ideas and thoughts for researchers and clinicians.
2. Osteoarthritis site lesion
Osteoarthritis is a disease that occurs in the entire joint, in dealing with articular cartilage degradation, synovium inflammation, subchondral bone thickened, osteophytes formed, ligaments and menisci degeneration (Bottini et al. Citation2016) (. Articular cartilage is an alymphatic, avascular, and aneural connective tissue, composed of chondrocytes and extracellular matrix (ECM). Chondrocytes are dispersed inside in the ECM which is composed of collagen, proteoglycans, lipids, and glycoproteins. The loss of matrix in OA refers to the unbalance of biosynthetic and degradation. During the former one, chondrocytes attempt to restore the damaged ECM with an increase in anabolic activities, however, resulting in a metabolic imbalance. Varies enzymes are produced in the latter phase; aggrecanases (especially, ADAMTS-4 and ADAMTS-5) and matrix metalloproteinase (MMP-3, MMP-7, MMP-13, etc.) are the two major catabolic enzymes in the progression of OA (Tetlow et al. Citation2001). In the early stage, the degradation of aggrecan is mainly by MMP-3 and ADAMTS-5. It seems that MMP-13 is the most important one in OA, and the expression is highly increased, as it efficiently cleaves type II collagen (Coll-II, accounts for nearly 90% of the collagen). The process of degradation of the collagen network constantly occurs, which will ultimately reach an irreversible state. Although it is not deemed OA to inflammatory arthritis, mediators of inflammatory are still detected in the articular cartilage. Tumor necrosis factor (TNF) and interleukin-1β (IL-1β) can not only influence chondrocyte metabolism but also induce chondrocytes producing inflammatory mediators, such as nitric oxide (NO), and prostaglandin E2 (Sandell & Aigner Citation2001). The substances mentioned above all can be considered as potential targets.
Subchondral bone locates just beneath the articular cartilage and can be categorized into two moieties: subchondral bone plate and subchondral trabecular bone. They hold the post of shock absorbing, supporting the normal joints (Li et al. Citation2013). In general, there are few biomarkers for subchondral bone have been identified which can be used as targets in OA treatment. Only type I collagen can be regarded as a biochemical marker of bone metabolism (Brandt et al. Citation2000). The connection between subchondral bone and articular cartilage is intimate as they possess the mutual function of bearing loads (Lajeunesse & Reboul Citation2003).
The knee menisci located between the articular surfaces of the tibia and femur in the medial and lateral compartments of the joint are two semicircular fibrocartilage structures. It absorbs shocks and transmits loads with knee joint moving and loading. Moreover, it also has benefits for joint stability, proprioception, and joint lubrication. The major components of the meniscus are collagen, proteoglycans, and water. The first two are responsible for tension, compression and shear stress resisting. Compared with cartilage, it mainly contains the type I collagen and the concentrations of the hydrophilic proteoglycans in the collagen matrix are much lower. It seems that meniscus damage might lead to OA and OA might cause meniscus damage. Common adult meniscus pathological changes often relate to traumatic or degenerative meniscal tears. An experiment showed that pathologic changes occurred in menisci was associated with NO production and meniscus cell apoptosis (Hellio Le Graverand et al. Citation2001a). Graverand et al. using rabbits for experimental osteoarthritis noticed that structural changes in rabbit knee menisci were time dependent (Hellio Le Graverand et al. Citation2001b). After anterior cruciate ligament (ACL) transaction, they found complex molecular changes in the medial and lateral meniscus, comprising elevation in the number of apoptotic cells, an increase in the mRNA expressions of type I collagen, aggrecan, and COX-2, while a significant decreasing tendency took place in decorin, TNF-α and IGF-2. The contents of matrix, as well as deposition and cell alternation, occurred in the early stage in the medial meniscus.
Ligaments work as joints stabilizers and joint motion guiders, owning seldom vascular. They have comparatively few cells described as “fibroblasts”; most of them appear as dense, white bands blending with surrounding tissues. The failure and instability of the ligaments may lead to OA in some way, and many focuses have been paid on ACL (Castaneda et al. Citation2012). Studies have shown that there was a link between meniscus and ACL in the progression of OA. A review has demonstrated the potential components for targeting the injured ACL (Fleming et al. Citation2005), suggesting that due to different activity degrees, MMPs, NO and exogen may have impacts on ACL by different mechanisms. For instance, MMP-1 degrades fibrillary collagen and elastin, and the former one may be a viscoelastic part of the ligaments (Frank & Shrive Citation1996).
3. Drug delivery systems for OA therapy
Local treatment via intra-articular injection is an appropriate strategy due to that OA only affects the joints. However, when small molecular drugs were introduced into IA space, they were easily and quickly removed by blood vessels and lymphatics. For example, methotrexate only achieved a half-life of 0.59–2.9 h after IA injection, and it was 1.9 h and 5.2 h for ibuprofen and diclofenac, respectively (Larsen et al. Citation2008). Furthermore, many drugs were hydrophobic molecules, and they were classified into type II or IV by BCS (biopharmaceutical classification system). A crystal suspension would be formed in IA space, providing a risk of crystal deposition and crystal synovitis. Therefore, a suitable drug delivery system was required for these drugs to increase the solubility and prolong their retention time in the articular cavity. Biodegradable and bioeliminable materials have been engineered to prepare drug delivery systems for IA injection. It has a great diversity of benefits, including but not limited to enhancing the stability of encapsulated drugs, decreasing toxicity, reducing adverse effects, improving pharmacokinetics, and targeting specific site (Niazvand et al. Citation2017; Wang et al. Citation2017; Yao et al. Citation2017a, Citation2017b; Kou et al. Citation2018a, Citation2018b, Citation2018c, Citation2018d; Yao et al. Citation2018). In this section, we will present several interesting biomaterials that have been tested as drug delivery systems for OA therapy, and their advantages and disadvantages will be discussed. Following that, these biomaterials-engineered drug delivery systems and potential drugs will be introduced.
3.1 Emerging biomaterials utilized for OA drug delivery
3.1.1 Chitosan
Chitosan is a derivation from chitin. Its favorable biological properties including biocompatible, biodegradable, and non-toxicity, make chitosan as a promising drug delivery carrier. In addition, chitosan is a water-soluble polymer which makes the process of preparation easier and milder. Chitosan nanoparticles can load vary macromolecules and drugs, besides it can target different tissues or organs through different routes. Using DNA-loaded chitosan-graft-polyethylenimine nanoparticles showed great success and high efficient in transfecting chondrocytes and synoviocytes. Lu et al. added hyaluronic acid in the vectors which enhanced the transfection efficiency (Lu et al. Citation2011). What’s more, using chitosan nanoparticles as drug carriers not only improves drugs’ retention time in cartilage but also alleviates osteoarthritis. Zhou et al. synthesized novel chitosan NPs loading berberine chloride (Zhou et al. Citation2015). It exhibited efficient anti-apoptosis activity in vivo as well as good stability and desiring releasing profile in vitro. The chitosan nanoparticles conjugated with Kartogenin (KGN) showed chondrogenic and chondroprotective properties (Kang et al. Citation2014). Chitosan oligosaccharide-conjugated pluronic F127 was prepared to deliver KGN and diclofenac in a single system; it exhibited excellent anti-inflammatory effects (Kang et al. Citation2016). Additionally, the use of Pluronic provided thermosensitivity. Both NPs possessed longer retention time in cartilage, which offers a promising direction for OA treatment (Kang et al. Citation2014, Citation2016a). Moreover, the abilities of free radicals scavenging and ostoinduction were provided through encapsulating inorganic material TiO2 into chitosan nanocarriers (Zhao et al. Citation2006). There are other forms of chitosan nano-delivery platforms, for example, chitosan/polyethylene oxide nanofibers, also showing great promise for cartilage tissue engineering (Subramanian et al. Citation2005; Wright et al. Citation2014).
3.1.2 Poly (lactic-co-glycolic) acid (PLGA)
Poly (lactic-co-glycolic) acid (PLGA) is a linear and biodegradable polymer. As a delivery carrier, PLGA is suitable for a wide range of biomolecules and can control substances release behaviors. Brown et al. studied the influencing factors of PLGA in OA treatment (Brown et al. Citation2019). They found out that modified with a quaternary ammonium cation on the surface of PLGA get the best retention behavior (). Cationic PLGA/Eudragit RL nanoparticles showed desirable and prolonged retention time in joints over 4 weeks (Kim et al. Citation2015). The results demonstrated that PLGA-based positively surface-charged NPs were promising tools in IA injection, and there was no sign demonstrating that this formulation had abnormal adverse effects. Niazvand et al. designed curcumin-loaded PLGA nanoparticles to test whether it can improve oral curcumin’s bioavailability (Niazvand et al. Citation2017). The formed nanoparticles not only had a better absorption but also boosted curcumin’s pharmacological effect on mono-iodoacetate-induced osteoarthritis of rats. Titania-loaded PLGA NPs were designed to evaluate the ability for bone regeneration (Liu et al. Citation2005). Because of the added nanophase Titania, the human-made composite attached osteoblasts tightly, further improving its functions with the proofs that more collagen, alkaline phosphatase, and protein generated. PLGA nano-scaffolds mimicking matrix may offer a new sight in tissue engineering and in turn treating OA. For instance, Arg-Gly-Asp (RGD), a cell adhesive peptide, immobilized onto the surface of a PLGA nanofiber mesh became a biomimetic substrate, which enhanced cell proliferation, attachment and spreading (Kim & Park Citation2006). Luo et al. combined graphene oxide (GO) into PLGA modified nanofiber scaffolds (Luo et al. Citation2015), and the novel scaffolds strengthened MSCs differentiation. As mentioned above, NO can be an available biomarker for OA. Herein, Jin et al. developed PLGA nanoparticles encapsulated NO sensing molecules (DAF-FM molecules) for detecting the progression of OA (Jin et al. Citation2017). In OA animal models, the fluorescence signal could be detected and increased during the experiment period, indicating its ability to monitor OA development. In cell culture, with PLGA degraded over time, the encapsulated DAF-FM molecules released up to 144 h and its stability to hydrolysis improved.
Figure 2. Ex vivo cartilage retention studies for PLGA NPs, indicating the interaction between NPs and cartilage. (A) Histological sections for (i) health cartilage and (ii) enzymatically digested OA cartilage. (B) Cytotoxicity of PLGA NPs to the cartilage (n = 6). (C) Quantified analysis of NPs’ retained in explant cartilage after incubation (n = 5). (D) Cross section of health and OA sample after incubated with NPs observed with a fluorescence microscopy (Scale bar = 100 µm). α, p < 0.05, β, p < .01 representing the significance between saline and synovial fluid treatments. Reprinted with permission from Brown et al. (Citation2019).
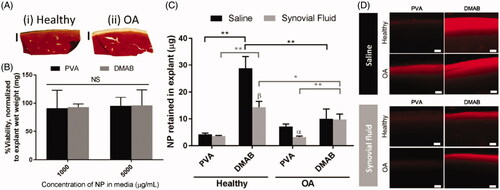
3.1.3 Poly (N-isopropylacrylamide) (pNiPAM)
Poly (N-isopropylacrylamide) (pNiPAM) is a thermoresponsive polymer for multiple bio-applications. It is worth noticing that its lower critical solution temperature (between 31 and 33 °C) allows pNiPAM becoming soluble below 31 °C (Mishra et al. Citation2014). Thus, it can load hydrophilic agents. Maudens et al. utilized pNiPAM thermo-responsive profile forming a homogeneous environment spontaneously (Maudens et al. Citation2018). As illustrated in , pNiPAM-modified HA showed a prolonged lifetime in vivo. McMasters et al. (Citation2017) modified pNiPAM with poly (ethylene glycol) (PEG) to produce hollow nanoparticles. The novel NPs can be able to directly deliver loaded peptides to the target sites and then function there. Another study also used the pNiPAM carrier to deliver the same peptide (Bartlett et al. Citation2013). As results demonstrated, the modified pNiPAM delivery system selectively targeted impaired sites. It is indicated that pNiPAM is a promising platform to treat OA.
Figure 3. Cartilage persistence of conventional HA and thermosensitive HA Nano 1 conjugated with cyanine 5. In vivo imaging system showed the retention of HA and HA Nano 1 after (A, B) intra-articular injection in an OA mouse model and (C, D) subcutaneous injection in healthy mice (n = 4). (E) Fluorescence micrographs of right knee 2 months after OA induction (F: femur, T: tibia, scale bar = 300 µm). (F) Fluorescence micrographs of the skin at 21 days after injection (D: Dermis, scale bar = 500 µm), and mice received an injection of PBS as the sham group. Reprinted with permission from Maudens et al. (Citation2018).
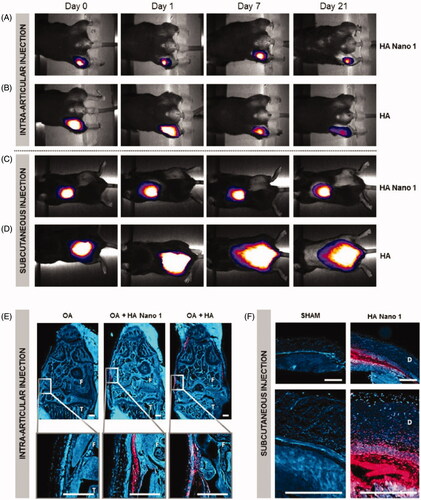
3.1.4 Avidin
Avidin is a widely used positively charged carrier. According to the related reports, avidin holds a weak and reversible electrostatic interaction with cartilage, resulting in a quick penetration into the whole layers of cartilage and an extremely long retention time in cartilage (Bajpayee et al. Citation2014). Bajpayee et al. used avidin as a cationic nano-delivery carrier (Bajpayee et al. Citation2016), and the release of dexamethasone (Dex) was sustained up to 3 weeks. Additionally, this kind of avidin-Dex nanoparticles exhibited a more powerful ability to suppress the procedure of catabolism and restrain GAG loss compared to free Dex.
3.1.4 Other biomaterials
Much more biomaterials with good biocompatibility have been tested for OA drug delivery and/or OA therapy. Polysaccharides, including chitosan, chondroitin sulfate (Bishnoi et al. Citation2014), hyaluronic acid (Jin et al. Citation2010), alginate (Tsukuda et al. Citation2015), etc. have been utilized for OA drug delivery. Especially, some of them could not function as a carrier but also as a macromolecule drug, like chondroitin sulfate and hyaluronic acid. The use of intra-articular HA for knee OA has been recommended in the clinic (Bannuru et al. Citation2019), and HA-based drug delivery system also displayed delightful results. Taken together, the drug-in-drug concept might give more benefits to relief OA development. Synthetic biocompatible polymers also exhibited good properties, like increasing drug solubility, sustained drug release, and prolonged drug retention. Except the mentioned PLGA and pNiPAM above, poly(lactic acid) (PLA) (Laroui et al. Citation2007), poly(caprolactone) (PCL) (Morgen et al. Citation2013), polyester amide (PEA) (Rudnik-Jansen et al. Citation2017), etc. have also been employed to make micro- or nano-scale particle for intra-articular drug delivery to treat OA. In addition, some other biomaterials, like phospholipid, dendrimer, and Pluronic, were also selected for exploration of OA drug delivery systems. To avoid too much overlap, these materials and these materials-derived formulations would be introduced in the following section.
3.2 Varying drug delivery systems
3.2.1 Micro-scale particles
Studies on advanced micro-techniques seeking for delayed drug release profiles, reducing the toxicity of agents and improving therapeutic effect, are widely undergoing. It is a good way to control drug release behavior and prolong residence time in target place (summarized in ). For instance, Zhang and coworkers used PLGA as a micro-vector (Zhang et al. Citation2011). As a result, it showed a sustained-release profile to 80% in 80 days. The area under the curve of plasma concentration in PLGA microspheres was much less than the free drug group, and the maximum plasma concentration was detected at 60 h. All of these validated that the PLGA microspheres could not only control drug release but also protect the loaded drug from rapid clearance. The reason may relate to the particle size (above 5 μm). Another research also found the proof that microparticles with 10 μm had more beneficial than 1 μm (Butoescu et al. Citation2009b), such as sustained release, increased half-life period, and prolonged therapeutic effect. In their follow-up study, they confirmed that the synthesized microspheres diminished systemic side effects in rat models (Zhang & Huang Citation2012). Another advantage of the micro-delivery system is reducing repeated injections. Goto et al. considered fluvastatin-loaded PLGA microspheres as a potential monthly treatment (Goto et al. Citation2017). A single injection of the microspheres successfully inhibited cartilage degeneration and significantly increased the amount of Coll-II. Because of the sustained-release property, the treatment effect of injecting parathyroid hormone 1–34 [PTH(1–34))-contained PLGA microspheres every 15 days was similar to injecting PTH(1–34) every 3 days for 5 weeks in OA rats (Eswaramoorthy et al. Citation2012). Furthermore, PLGA microspheres are also used for bio-macromolecules delivery for OA therapy and displaying promoted effect (Morille et al. Citation2013, Citation2016). Elsaid et al. encapsulated interleukin-1 receptor antagonist (IL1-ra) into PLGA microspheres (Elsaid et al. Citation2016). It was shown that these microspheres did not influence the bioactivity of IL 1-ra, even enhanced its disease-modifying effect. From the results, we can find that PLGA IL-1 ra treatment highly halted cartilage degeneration as well as synovial pathology compared with PBS or PLGA treatments. Meanwhile, Safranin O and H&E results exhibited the cartilage integrity and normal appearing synovium of the PLGA IL-1 ra-treated group.
Table 1. The micro-scale particles applied for OA therapy.
Other polymeric materials are also used to form micro-scale delivery system for OA remedy. Chitosan microsphere is a potent tool to maintain a prolonged effective plasma concentration. Abd-Allah et al. made chitosan/tripolyphosphate (TPP) microspheres, in which TPP was used as an anionic cross-linker (Abd-Allah et al. Citation2016). The contained lornoxicam was gradually released over 8 days in vitro and retained in joint during the whole period of the study. OA rats treated with this formulation showed a long-term suppressed inflammatory activity in vivo with relative parameters superior to lornoxicam solution-treated group. Arunkumar et al. investigated the potential of polycaprolactone (PCL) microparticles to deliver drugs for OA (Arunkumar et al. Citation2016b). As in vivo results showed, the drug was slowly released, and the whole microparticles were still detected in the joint after one month. Sulistio and co-researchers developed polyurethane-based microgel, which provided a prolonged release of diclofenac and the ability to retain in the joint cavity (Sulistio et al. Citation2019). They proposed a view that free diclofenac in microgel for short-term effect while gradually released conjugated diclofenac for long-term therapeutic effect. It was clearly showed in Figure S1, a strong signal was detected in the formulation-treated group at all time points.
3.2.2 Nano-scale particles
Compared to the microparticles, nanoparticles could also increase the drug solubility, control drug release, and prolong the residence time. Nanoparticles were usually prepared as solid drug carriers by biodegradable polymers or lipids, and they could encapsulate almost all kinds of hydrophobic drugs due to the similar property and some hydrophilic drugs by nanoprecipitation method. It has been demonstrated that nanoparticles reduced the plasma concentration of loaded drugs compared with free drug administration, confirming the controlled release profile (Jain et al. Citation2014). Morgen et al. developed a kind of nanoparticles by crosslinking PCL(polycaprolactone)-PEO(poly ethylene oxide) diblock copolymer with dextrin (Morgen et al. Citation2013). The nanoparticles showed prolonged residence time in rat knees after IA injection. It was shown that 70% of nanoparticles were retained in the joint up to one week. Even though nano-scale particles could avoid elimination by the microvascular pathway to some extent, the retention time for them is still less than the micro-scale particles due to the bigger particle size (Natarajan et al. Citation2011). However, what should not be overlooked is that nano-sclae particle exhibited enhanced penetration into cartilage. Taking avidin as a carrier, it could penetrate the full thickness of cartilage due to the reversible interaction between carriers and proteoglycans (Bajpayee et al. Citation2014). Some other studies on nano-scale particles for OA drug delivery have been summarized in .
Table 2. The nano-scale particles applied for OA therapy.
3.2.3 Hydrogels
Hydrogel is a kind of three-dimensional polymer network with high water content similar to living tissues. Hydrogel is usually considered as a good drug platform due to the bio-adhesive property to the epithelium and mucosa, the flexibility in sizes and the toughness. These properties indicated the superiority of hydrogel in tissue engineering, including promoting the accumulation of GAG in the matrix, enhancing chondrocytes proliferation and transplantation. Choi et al. modified chitosan hydrogels contained Col II and Chondroitin sulfate (ChS) for cartilage regeneration (Choi et al. Citation2014). Its morphology and cell adhesion ability are shown in . As results shown in , after cultured with chondrocytes, cellular condensation enhanced, chondrogenesis, and cell–matrix adhesion increased. Furthermore, the prolonging drug’s residence time in desired sites, in turn, promoted the therapeutic effects of the drug (Garcia et al. Citation2019). For instance, Massaro et al. formed hydrogel based on halloysite and laponite to deliver KGN (Massaro et al. Citation2019). From Figure S2, we can see that the release of KGN was significantly prolonged through the hybrid carrier.
Figure 4. Morphology of hydrogels and evaluation using 3D cultured chondrocytes via histological analysis. (A) SEM images of hydrogels showing the interior morphology of hydrogels (upper images, scale bar = 5 µm) and cell adhesion to the hydrogels (lower images, scale bar = 10 µm). (B) Histological analysis of chondrocytes in hydrogel systems during 6-week culture, including H&E (top images and quantified data of aggregate area at 6 weeks), safranin-O (middle images and quantified data of sGAG at 6 weeks), and immunohistochemical staining of Col II (bottom images and quantified data of Col II at 6 weeks) (scale bar = 200 µm). n = 3; *p < .05 and **p < .01 compared with MeGC; ##p < .01 compared with other groups. Reprinted with permission from Choi et al. (Citation2014).
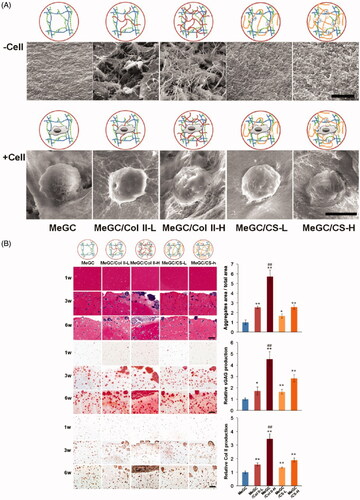
Alginate is an anionic polymer in nature with biocompatibility and low-toxicity, suitable to be utilized in sustained drug delivery systems. Tsukuda et al. tested the potential of ultra-purified low endotoxin alginate (UPLE-alginate) in a canine OA model (Tsukuda et al. Citation2015). No synovitis or degenerative changes of articular cartilage were found in any knees. Histologic scores were much better in the therapeutic group than the control one (medial femoral condyle: p = .009, lateral femoral condyle: p = .009). It could be concluded that the administration of UPLE-alginate through IA injection does delay the progression of OA in beagle dogs. Krebs and coworkers produced alginate hydrogels consisting of calcium phosphate–DNA (Krebs et al. Citation2010). Controlled drug release and osteoblasts (OB) formation were observed after the local injection of the prepared hydrogels. Fernandez et al. incorporated decorated plasmid DNA (pDNA) into alginate hydrogels and successfully transfected MSCs (Gonzalez-Fernandez et al. Citation2016). A significant rise in sulfated-glycosaminoglycan (sGAG) and collagen production was achieved, indicating the great possibility for applications in bone regeneration. Periodate-oxidized alginate hydrogels were injectable without inflammation or oxidative pressure (Balakrishnan et al. Citation2014). It was elucidated that the alginate hydrogels could promote chondrocytes proliferation and attaching to increase GAG, collagen and aggrecan deposition in the matrix, indicating the superior ability to restore cartilage.
3.2.4 Liposomes
Liposomes are colloidal particles consisting of a lipid bilayer. As a drug vehicle, liposomes can load numerous kinds of agents regardless of hydrophobicity and with desirable payloads. When applying in the treatment of OA, Yeh et al. validated the increasing uptake in bone tissue via combining relative agent in liposomes (Yeh et al. Citation2015). Frisbie et al. investigated the potential of diclofenac liposomal cream (DLC) on OA horses (Frisbie et al. Citation2009). It was shown that treatment with DLC significantly reduced the sclerosis of the radial wrist and the erosion of the cartilage. The prominent therapeutic effects for increasing the content of glycosaminoglycan in articular cartilage and decreasing PGE2 concentration were observed. Additionally, no cases of treatment-related side effects were found. Sivan et al. studied those liposomes carrying different phosphatidylcholines (PCs) could perform as highly effective cartilage biolubricants (Sivan et al. Citation2010). Williams et al. developed liposomal formulations that suppressed joint inflammation in arthritis rats after a single injection (Williams et al. Citation1996). Liposomes prevent inside drugs from metabolic process and obtain prolonged half-lives by reducing its size or modulating its surface (mostly using PEG) (Daraee et al. Citation2016). Some modified liposomes have abilities to target specific cells or designated place (cartilage surface, synovium membrane, articular space). Comparing with free drugs, liposomes significantly decreased the cytotoxicity and adverse effect.
3.2.5 Micelles and dendrimers
Micelles usually comprise a core–shell structure. As promising carrier platforms, micelles have favorable biocompatibility. Matsuzaki et al. investigated the treatment effect of rapamycin micelles against OA through IA injection (Matsuzaki et al. Citation2014). Compared with the group of gelatin hydrogels alone, rapamycin-micelles showed long-term effect, and the OA score remained decreased. Zhang et al. fabricated amphiphilic micelles loading indomethacin (Zhang et al. Citation2007). Comparing with administrating indomethacin solution, micelles carried indomethacin performed delayed drug release behavior. Of note, severe gastrointestinal stimulation was avoided via local injection of this kind of liposomes.
Dendrimers, derived from the Greek word dendron, can be described vividly as a widely branched 3 D architecture. They have three major constructions: the core, the interior, and the shell. They are used to encapsulate and dissolve hydrophobic drugs into the interior. Dendrimers are water-soluble, polyvalent, and monodispersed, and with a nano-diameter, allowing for easily crossing the bio-barriers. It is confirmed that dendrimers could be used as drug carriers for loading hydrophobic drugs and controlled drug release. Dendrimers have been used in the treatment of OA; for instance, different studies have validated that indomethacin-loaded polyamidoamine (PAMAM) dendrimer can target inflammatory regions of arthritic rats (Hayder et al. Citation2011; Hu et al. Citation2017). Partly PEGylated PAMAM dendrimers were used as the platforms to deliver KGN in the study of Hu et al. (Hu et al. Citation2017). They formed two types: KGN was conjugated to the surface of PAMAM (PPK), and the other one was conjugating KGN to the end group of PEG (KPP), and the sizes of both dendrimers were below 40 nm. After IA injection in healthy and OA knees, signals of cyanine 7-PEG-PAMAM still could be detected in the rats after 21 days, while free Cy7 did not show any signal after 24 h. Cells treated with KPP showed the highest expressions and immunostaining of chondrogenic markers. The enhanced delivery and therapeutic effects of KGN was ascribed to the formed dendrimers would persist in the joint cavity.
3.2.6 Combinational drug delivery systems
Combinational drug delivery platforms in treating OA were referred to the combination of different types mentioned above. For instance, beads or microspheres dispersed in a hydrogel or gel (Oprenyeszk et al. Citation2013; Arunkumar et al. Citation2016a; Xia et al. Citation2017), gelatin hydrogels comprised micelles (Matsuzaki et al. Citation2014), nanoparticles-in-microparticles system (Butoescu et al. Citation2009a; Chen et al. Citation2014), and others. Results gained from different researches validated the unique properties of combination structures. Kang et al. synthesized self-assembled PEGylated KGN micelles, and they were contained in HA hydrogels (Kang et al. Citation2017). The in vitro consequences showed that the KGN had a rapid release from micelles while it had a sustained release in hydrogels. In vivo, the articular cartilage from HA/PEG/KGN hydrogels-treated rats had similar COL II and aggrecan staining to the normal rats’ articular cartilage. In the study of Bian et al., compared with gels loaded agents solely, they suggested that HA hydrogels carried both MSCs and TGF-β-loaded microspheres acquired favorable mechanical properties and superior matrix content (Bian et al. Citation2011). The microspheres also protected TGF-β from rapid clearance at the beginning, with a prolonged release time up to 6 d. Arunkumar et al. and Chen et al. reported similar strategies to avoid burst release and gain better drug retention (Chen et al. Citation2014; Arunkumar et al. Citation2016a). Arunkumar et al. could detect the drug in plasma after six weeks (Arunkumar et al. Citation2016a). Chen et al. measured that the burst release of combination forms descended 9% (Chen et al. Citation2014). Obviously, the combination of different kinds of preparations could amplify the therapeutic efficacy, indicating the great potential of them for further study and clinical use.
3.3 Potential therapeutic drugs
An abundant scrutiny focuses have been paid on the pathology of OA. It is undeniable that factors influencing the balance between anabolism and catabolism exert impacts on the early or advanced stage of OA more or less. It seems that IL-1β, TNF-α, and IL-6 are the main proinflammatory cytokines that facilitate OA onset and deterioration. Others including mediators (NO, prostaglandin E2), growth factors [transforming growth factor-β (TGF-β), BMPs, insulin-like growth factor-1 (IGF-1), fibroblast growth factors (FGFs)], and enzymes (MMPs, aggrecanases) increasing or decreasing all have been found in OA patients. Additionally, NF-кB signaling is regarded as a key pathway in the cartilage defect. In this section, we will focus on the drugs used for OA therapy.
3.3.1 Small molecular drugs
Corticosteroids are widely used in arthritis treatment since they have potent in anti-inflammatory and anti-catabolic. They are capable of blocking phospholipase A2, thereby inhibiting the arachidonic acid cascade and the generation of kinins, prostaglandins, and other inflammatory mediators. It has been proved that low dose of corticosteroids such as dexamethasone, triamcinolone, and prednisolone, exerts effects on down-regulating the gene expression level of MMPs (MMP-1, MMP-3, and MMP-13) in chondrocytes, reducing GAG loss and up-regulating the synthesis of proteoglycan in cartilage explants exposed to TNF-α (Lu et al. Citation2011). The latent side effects of using corticosteroids have been reviewed (Schumacher & Chen Citation2005), and they are varying from the central nervous system (headache, visual loss) to local organs and tissues (gastrointestinal dysfunction), and even causing immune system disability. Osteoporosis is more common occurred among long-term use of patients, and high doses of injection repeatedly result in systemic toxicity (Leonard et al. Citation2004). Consequently, modifying corticosteroids appears to be particularly important. Saadat et al. designed triamcinolone loaded nano-scale micelles for OA therapy (Saadat et al. Citation2015). Compared with free triamcinolone, the drug from micelles detected in plasma was lesser due to the sustained release behavior. In turn, it would minimize systemic adverse effects. Lee et al. provided another form, nanotubes, to relieve this kind of side effects and dose problem (Lee et al. Citation2016). Triamcinolone conjugated on PEG-fabricated carbon nanotubes not only increased intracellular uptake but also suppressed inflammatory responses. It was succeeded in fabricating dexamethasone with different types of nanomaterials to seek prolonged release profile, for example, avidin nano-carriers or kartogenin conjugated chitosan nanosystem (Kang et al. Citation2014; Bajpayee et al. Citation2017; Bajpayee et al. Citation2016). Amjadian et al. explored the synergistic effect between nano-hydroxyapatite and dexamethasone (Amjadian et al. Citation2016). The sustained release of dexamethasone led to more osteogenic differentiation, indicating the capacity of tissue regenation. Zhao and coworkers developed near-infrared light-responsive nanosheets that can efficiently control the release of dexamethasone (Zhao et al. Citation2019). In Figure S3, dexamethasone release behavior was related to the density of NIR light. Thus, this platform could control drug release in the joint cavity and got desired therapeutic effects.
Non-steroidal anti-inflammatory drugs (NSAIDs) are well-known and widely used in inflammation. It also downregulates proinflammatory mediators in OA, including IL-1β, TNF-α, NO, MMP-13, cathepsin K, and aggrecanases. In addition, NSAIDs have effects on pain reduction. Varadi et al. suggested transdermal ibuprofen could allay knee OA in a randomized trial (Varadi et al. Citation2013). However, the adverse reactions, low penetration and short action time limited the long-term and frequent use of NSAIDs. The ibuprofen-loaded nanostructure lipid carrier-based gels had a great potential of skin penetration and ulteriorly accelerated anti-inflammatory efficacy in chronic OA (Suto et al. Citation2016). Kim et al. developed piroxicam-loaded cationic NPs which were selectively detained in the joint and sustained released the drug for OA therapy (Kim et al. Citation2016). Canto et al. encapsulated piroxicam into liposomes, which had an increase anti-inflammatory effect with a minimum dose (Canto et al. Citation1999). Javed and coworkers fabricated lecithin-gold nanocarriers for diacerein delivery (Javed et al. Citation2016). The improved inflammation suppression activity of diacerein may relate to the structure of the delivery system, increased oral bioavailability, and the controlled release characteristic.
Curcumin is a naturally active polyphenol derived from the rhizome of the herb Curcuma longa. Many studies have been performed to determine the biological activities of curcumin, and one of the branches is focused on its impacts on chondrocytes, cartilage and further on the rheumatic disease. Evidence suggested that curcumin has an influence on mitigating the progression of OA disease; even relive OA-related pain through effecting diverse cytokines and related gene expression (Zhang et al. Citation2016). It decreases PGE2, MMP-3 excretion from explants, and inhibits IL-6, IL-8 with a time-depended and dose-depended behavior (Clutterbuck et al. Citation2013). Curcumin prevents chondrocytes from apoptosis by suppressing IL-1β, TNF-α, and COX-2 meanwhile decreases ECM degradation (Henrotin et al. Citation2010, Citation2013). What should not be overlooked is that curcumin regulates NF-кB signaling and in turn meditates IL-1β and MMPs gene expression (Henrotin et al. Citation2010). With all these merits, a barrier that impedes the utilization of curcumin is the low bioavailability. What’s more, its metabolic process is rapid, and the metabolites do not possess the same anti-inflammatory or anti-catabolic activities as curcumin (Henrotin et al. Citation2010). Researchers prepared curcumin encapsulated PLGA nanoparticles and obtained satisfactory results in bioavailability and retention time (Zabihi et al. Citation2014). The study of Zhang et al. validated that nano-scale curcumin remained its anti-inflammatory and anti-catabolic characteristics (Zhang et al. Citation2016).
3.3.2 Macromolecule
Hyaluronic acid (HA) is one of the important contents of both cartilage and synovial. Studies have demonstrated that HA owns several qualities including chondroprotective effect, stabilizing and maintaining the integrity of cartilage. HA exhibits its clinical efficiency in experimental animal models and patients (Navarro-Sarabia et al. Citation2011). Owing to its viscous and elastic nature, high molecular weight of HA maintains the rheological homoeostasis of the synovial fluid and also contributes to shock absorption, lubrication and provides nutrition for the joint tissue. It has positive impacts on suppressing gene expression of inflammatory mediators (MMP-3 and IL-1β) and NO, protection of matrix degradation as well as enhancing matrix proteins’ biosynthesis, improving joint function (Brandt et al. Citation2000). The administration of HA through IA injections to relieve pains in OA patients was noticed. Food and Drug Administration (FDA) has already proved several kinds of HA injections, like Hylan G-F 20®, Hyalgan®, Adant®, and Synvisc-one®. However, most HA preparations only remain in the joint for a few hours (half-life, 17 h). With extremely rapid clearance and quickly degradation in vivo by enzymatic or hydrolytic reactions, localization of response in biological environments is likely to be a problem. Thus, repeated injections are required, however, arising more side effects and poor patient’s compliance. Hence, various methods have been developed to improve the treatment. Taking advantage of Michael addition, Jin et al. modified HA with PEG in different concentrations (Jin et al. Citation2010). As such, it delayed the degradation procedure of HA and extended the retention time. Conjugating HA onto a self-assembled nano-polymer under body temperature offered a new strategy for prolonged HA and drug retention time (Maudens et al. Citation2018). Chen et al. fabricated HA coated bovine serum albumin nanoparticles, which had a significant long retention time in the articular cavity (more than 14 d) after IA injection (Chen et al. Citation2013). Moreover, these spontaneous NPs may have further improvements in delivering other macro or small molecules. The efficiency of HA hydrogel in treating chondral lesion in rabbit knees has been demonstrated. Chen et al. combined ADAMTS-5 inhibitor, 114810, with HA hydrogel and investigated its potential for OA treatment (Chen et al. Citation2014). The results showed that this kind of HA hydrogel could promote cartilage healing, suppress osteochondral defects and halt the progression of degeneration, providing a new strategy for OA therapy. Patterson et al. designed a scaffold system with HA to deliver cationic proteins (Patterson et al. Citation2010). Under the action of electrostatic, it displayed a continuous release behavior.
Chondroitin sulfate (ChS), belongs to glycosaminoglycan (GAG), has been used in the therapy of OA. ChS has anti-inflammatory activity and varies biological functions, such as anti-oxidation, anti-thrombosis, and insignificant immunogenicity. A review showed that nanocarriers decorated by ChS could target CD44; utilizing ChS can enhance gene transfection of nanoparticles, and stabilize metal nanoparticles (Bishnoi et al. Citation2014). Also, Chs is the major constituent of articular cartilage and becomes a homing carrier to bind chondrocytes (Zhao et al. Citation2015). Jain et al. modified diacerein-loaded solid lipid nanoparticles with ChS (ChS-DC-SLN) to target articular cartilage (Zhao et al. Citation2015). In that study, the concentration of rhein (an active diacerein metabolin) at the targeted site (cartilage) increased remarkably in case of ChS-DC-SLN than that of the normal drug. In the meantime, the ChS-DC-SLN showed an extended release, and the bioavailability of the diacerein increased (Zhao et al. Citation2015). Yin et al. decorated platinum nanoparticles (PtNPs) with ChS (Mueller & Tuan Citation2011). It exhibited not only a green alternative approach but also a potential platform for treating OA.
KAFAKLAARLYRKALARQLGVAA (abbreviated KAFAK) is a cell-penetrating peptide, and it has been confirmed that KAFAK could regulate p38 mitogen-activated protein kinase (MAPK) activated protein kinase 2 (MK2) activities and exert effects on anti-inflammatory (Bartlett et al. Citation2013; Poh et al. Citation2015). Bartlett II et al. had examined the efficiency of applying anionic-polymer platforms for delivering KAFAK (Bartlett et al. Citation2012). The colloidal nanoparticles were stable and protected KAFAK from blood elimination. In their further research, in order to improve the KAFAK release profile, N, O-dimethacryloyl hydroxylamine (DMHA) was applied, and it enabled selective release over 4 d and allowed for degradation under physiological condition (Bartlett & Panitch Citation2012). They proved that this system could selectively target inflamed cartilage explants. Another platform, PEGylated pNiPAM nanoparticles, also exhibited targeting characteristic as well as delayed release feature (Lin et al. Citation2016). The hollow KAFAK-loaded PEGylated pNiPAM nanoparticles were produced to be utilized in the ex vivo explanted cartilage (McMasters et al. Citation2017). Obviously, the hollow particles had greater loading efficiency, longer release time and enhanced cell uptake than the solid ones, which in turn, strengthened the ability of KAFAK to inhibit IL-6.
Interleukin-1 receptor antagonist (IL-1 ra) is a promising therapeutic in modifying OA (Elsaid et al. Citation2016). Whitmire et al. developed 300 nm particles to delivery IL-1ra by IA injection (Whitmire et al. Citation2012). The IL-1Ra-loaded nanoparticles overcame rapid clearance by fluid. In their further study, they proved that localized delivery of larger size nanoparticles had a more desirable retention time in joints.
Others like insulin, due to promoting cells growth, may have an impact on suppressing OA. Cai et al. not only confirmed the single insulin effects on OA but also developed a delivery system which slowly released insulin (Cai et al. Citation2002). Insulin improved the synthesis of PG in the media with or without IL-1. It is found that basic fibroblast growth factor (bFGF) has certain abundance in the ECM of cartilage (Ellman et al. Citation2008). With the ability to stimulate cellular mitogenesis (including chondrocytes, MSC and OB, bFGF) it may have potential in treating OA (Inoue et al. Citation2006).
3.3.3 Gene therapy
In short, gene therapy refers to delivering nucleic acid to the target tissue using direct methods (in vivo) or transducing (ex vivo) by cells using viral or non-viral vectors. As Madry et al. mentioned, gene therapy for OA treatment does not focus on restoring abnormal OA-relative genes; it’s more like a delivery platform to suppress the expression of genes that promote OA development or stimulate therapeutic factors overexpression (Madry & Cucchiarini Citation2016). Many reviews have already thoroughly introduced OA-related gene therapy (Madry & Cucchiarini Citation2016; Evans et al. Citation2018; Grol & Lee Citation2018); here, we are more interested in combining biomaterial system with gene therapy to treat OA.
In several research teams, chitosan seems to be a useful gene vector for OA treatment. Zhang et al. formed chitosan–DNA nanoparticles directly injected into the joints of OA rabbits (Zhang et al. Citation2006). Lu and coworkers developed different novel non-viral chitosan vectors to deliver plasmid-DNA (Lu et al. Citation2011, Citation2014). And, they both validated that non-viral copolymers were a safe and efficient approach for gene delivery to chondrocytes and synoviocytes. The contained IL-1Ra gene was successfully transfected, and the severity of histologic cartilage lesions had a significant reduction. Polymeric materials containing gene vectors may overcome delivery barriers, such as short-term efficiency, high immunogenicity. Rey-rico et al. formed polymeric micelles to control the release of TGF-β (Rey-Rico et al. Citation2017). Compared with free vector treatment, using micelles increased the levels of TGF-β transgene expression, which in turn promoted proteoglycan deposition and enhanced cell numbers. While in osteochondral defect cultures, significantly higher levels of type-II collagen deposition and less hypertrophic events were noted in the copolymer-cultured group. All of these indicated the potential of using biomaterials to remodel OA cartilage.
Hypoxia-inducible factor-2α (Hif-2α) can induce chondrocytes to produce MMPs, ADAMTs and other catabolic factors (Yang et al. Citation2010). Pi et al. developed chondrocyte-homing nanoparticles to topically deliver anti-Hif-2α siRNA and got satisfied results in halting OA cartilage degeneration (Pi et al. Citation2015). Catabolic factors including Hif-2α, MMP-13 and -9 and ADAMTS-4 were decreased after siRNA successfully transfected in vitro. The cartilage of mice that got IA injection of nanoparticle-mediated anti-Hif-2α siRNA showed integrity and synovium inflammation alleviated. Meanwhile, by using nano-system, siRNA had a prolonged resident time and higher concentration in the cartilage. Sacchetti et al. modified single-walled carbon nanotubes, which successfully delivered anti-green fluorescent protein (GFP) “third generation” morpholino antisense oligonucleotides both in healthy and OA mice (Sacchetti et al. Citation2014). After IA injection, the formulation retained in the healthy joint for 2 weeks. It broke through the ECM barrier and accumulated in the desired sites as early as 3 d after injection. Aini et al. developed nanomicelles to carry runt-related transcription factor (RUNX) 1 mRNA (Aini et al. Citation2016). After injection, cartilage-anabolic markers and exogenous protein expression increased in OA mouse model, indicating the progression of OA was highly ameliorated.
4. Conclusion and outlook
Novel delivery platforms have exhibited their benefits and advantages in halting or delaying OA and relieving associated symptoms. In comparison with traditional formulations, nano- and micro-technology, hydrogels and liposomes have already demonstrated their superiority in retarding kidney filtration, extending drug residence time and in turn getting better therapeutic efficiency, enhancing patients’ compliance. Of them, it was evidenced that microparticles displayed a more prolonged drug release than other drug delivery systems. The polymer particles developed by Maudens et al. enabled a 2-month drug bioactivity after IA injection in an OA mouse model (Maudens et al. Citation2018). Triamcinolone acetonide-loaded PLGA particles (45 µm) have been processed into the Phase III clinical trial. Furthermore, the combination of various drug delivery systems showed so many advantages, like slowing down the burst release of loaded agents, protecting them from rapid clearance and enhancing the therapeutic effects, demonstrating their superiority in OA drug delivery. It seems that the complex drug delivery system holds great promise for OA therapy in the future, not only in the lab research but also in clinical application.
While extensive progress had been made to halt or escalate OA progression through the development of nano- and micro-materials, opportunities for improvement still exist. Challenges include further clinical studies, as experimental models’ severity may not as severe as in clinics. In addition, except for the sustained release profile, we cannot neglect the penetration property of drug delivery systems. The former review has summarized average cartilage thickness of different mature animals, the thickness of common experimental animals like mouse, rats, and rabbits are within 700 μm while the cartilage thickness of human is about 1.5–2.0 mm (Bajpayee & Grodzinsky Citation2017). Thus, drug diffusion and retention behaviors are different among animal species, and rodent models might overestimate the penetration ability of drug delivery systems. This critical issue should be taken into consideration in the further study of drugs or drug delivery systems for cartilage delivery for OA therapy.
IA administration has provided many benefits and advantages for OA therapy, including but not limited to reduced side effects and toxicity, decreased dosage, and limited organ exposure. However, the clinician found some defects for IA injection in clinic. The first issue is the patient compliance. HA intra-articular injection has been used as viscosupplements to treat knee OA. Most patients get a shot a week for 3–5 weeks. The process is torturous for patients, but they have to receive that frequently. The sustained drug delivery system mentioned above might be an answer to this problem, but this issue should be taken into deep consideration all the time. Infection after intra-articular injection is another problem, and especially patients receiving glucocorticoid IA injection are at high risk of infection. Sterilization is critical for drug development and clinical drug use, but only a few studies paid attention to the sterilization of their novel drug delivery systems. Furthermore, drug delivery systems for OA therapy have made much progress and display great promise for sustained and efficient drug delivery for joint. However, no disease-modifying osteoarthritis drugs (DMOADS) are available until today. New and effective DMOADs are required for these novel drug delivery systems to fulfill the medical need for OA treatment.
Supplemental Material
Download MS Word (512.5 KB)Disclosure statement
The authors declare no competing interests.
Additional information
Funding
References
- Abd-Allah H, Kamel AO, Sammour OA. (2016). Injectable long acting chitosan/tripolyphosphate microspheres for the intra-articular delivery of lornoxicam: optimization and in vivo evaluation. Carbohydr Polym 149:263–73.
- Aini H, Itaka K, Fujisawa A, et al. (2016). Messenger RNA delivery of a cartilage-anabolic transcription factor as a disease-modifying strategy for osteoarthritis treatment. Sci Rep 6:18743.
- Amjadian S, Seyedjafari E, Zeynali B, et al. (2016). The synergistic effect of nano-hydroxyapatite and dexamethasone in the fibrous delivery system of gelatin and poly(l-lactide) on the osteogenesis of mesenchymal stem cells. Int J Pharm 507:1–11.
- Arunkumar P, Indulekha S, Vijayalakshmi S, et al. (2016a). Poly (caprolactone) microparticles and chitosan thermogels based injectable formulation of etoricoxib for the potential treatment of osteoarthritis. Mater Sci Eng C Mater Biol Appl 61:534–44.
- Arunkumar P, Indulekha S, Vijayalakshmi S, et al. (2016b). Synthesis, characterizations, in vitro and in vivo evaluation of Etoricoxib-loaded Poly (Caprolactone) microparticles – a potential Intra-articular drug delivery system for the treatment of Osteoarthritis. J Biomater Sci Polym Ed 27:303–16.
- Aydin O, Korkusuz F, Korkusuz P, et al. (2015). In vitro and in vivo evaluation of doxycycline-chondroitin sulfate/PCLmicrospheres for intraarticular treatment of osteoarthritis. J Biomed Mater Res B Res 103:1238–48.
- Bajpayee AG, De la Vega RE, Scheu M, et al. (2017). Sustained intra-cartilage delivery of low dose dexamethasone using a cationic carrier for treatment of post traumatic osteoarthritis. eCM 34:341–64.
- Bajpayee AG, Grodzinsky AJ. (2017). Cartilage-targeting drug delivery: can electrostatic interactions help? Nat Rev Rheumatol 13:183–93.
- Bajpayee AG, Quadir MA, Hammond PT, et al. (2016). Charge based intra-cartilage delivery of single dose dexamethasone using Avidin nano-carriers suppresses cytokine-induced catabolism long term. Osteoarthritis Cartilage 24:71–81.
- Bajpayee AG, Scheu M, Grodzinsky AJ, et al. (2014). Electrostatic interactions enable rapid penetration, enhanced uptake and retention of intra-articular injected avidin in rat knee joints. J Orthop Res 32:1044–51.
- Balakrishnan B, Joshi N, Jayakrishnan A, et al. (2014). Self-crosslinked oxidized alginate/gelatin hydrogel as injectable, adhesive biomimetic scaffolds for cartilage regeneration. Acta Biomater 10:3650–63.
- Bannuru RR, Osani MC, Vaysbrot EE, et al. (2019). OARSI guidelines for the non-surgical management of knee, hip, and polyarticular osteoarthritis. Osteoarthritis Cartilage. pii: S1063-4584(19)31116-1
- Bartlett RL, Medow MR, Panitch A 2nd, et al. (2012). Hemocompatible poly(NIPAm-MBA-AMPS) colloidal nanoparticles as carriers of anti-inflammatory cell penetrating peptides. Biomacromolecules 13:1204–11.
- Bartlett RL 2nd, Panitch A. (2012). Thermosensitive nanoparticles with pH-triggered degradation and release of anti-inflammatory cell-penetrating peptides. Biomacromolecules 13:2578–84.
- Bartlett RL 2nd, Sharma S, Panitch A. (2013). Cell-penetrating peptides released from thermosensitive nanoparticles suppress pro-inflammatory cytokine response by specifically targeting inflamed cartilage explants. Nanomedicine 9:419–27.
- Bedouet L, Moine L, Pascale F, et al. (2014). Synthesis of hydrophilic intra-articular microspheres conjugated to ibuprofen and evaluation of anti-inflammatory activity on articular explants. Int J Pharm 459:51–61.
- Bian L, Zhai DY, Tous E, et al. (2011). Enhanced MSC chondrogenesis following delivery of TGF-beta3 from alginate microspheres within hyaluronic acid hydrogels in vitro and in vivo. Biomaterials 32:6425–34.
- Bishnoi M, Jain A, Hurkat P, et al. (2014). Aceclofenac-loaded chondroitin sulfate conjugated SLNs for effective management of osteoarthritis. J Drug Target 22:805–12.
- Bottini M, Bhattacharya K, Fadeel B, et al. (2016). Nanodrugs to target articular cartilage: an emerging platform for osteoarthritis therapy. Nanomedicine 12:255–68.
- Brandt KD, Smith GN Jr, Simon LS. (2000). Intraarticular injection of hyaluronan as treatment for knee osteoarthritis: what is the evidence? Arthritis Rheum 43:1192–203.
- Brown S, Pistiner J, Adjei IM, et al. (2019). Nanoparticle properties for delivery to cartilage: the implications of disease state, synovial fluid, and off-target uptake. Mol Pharm 16:469–79.
- Butoescu N, Seemayer CA, Foti M, et al. (2009a). Dexamethasone-containing PLGA superparamagnetic microparticles as carriers for the local treatment of arthritis. Biomaterials 30:1772–80.
- Butoescu N, Seemayer CA, Palmer G, et al. (2009b). Magnetically retainable microparticles for drug delivery to the joint: efficacy studies in an antigen-induced arthritis model in mice. Arthritis Res Ther 11:R72.
- Cai L, Okumu FW, Cleland JL, et al. (2002). A slow release formulation of insulin as a treatment for osteoarthritis. Osteoarthritis Cartilage 10:692–706.
- Canto GS, Dalmora SL, Oliveira AG. (1999). Piroxicam encapsulated in liposomes: characterization and in vivo evaluation of topical anti-inflammatory effect. Drug Dev Ind Pharm 25:1235–9.
- Castaneda S, Roman-Blas JA, Largo R. (2012). Subchondral bone as a key target for osteoarthritis treatment. Biochem Pharmacol 83:315–23.
- Chen P, Zhu S, Wang Y, et al. (2014). The amelioration of cartilage degeneration by ADAMTS-5 inhibitor delivered in a hyaluronic acid hydrogel. Biomaterials 35:2827–36.
- Chen Z, Chen J, Wu L, et al. (2013). Hyaluronic acid-coated bovine serum albumin nanoparticles loaded with brucine as selective nanovectors for intra-articular injection. Int J Nanomedicine 8:3843–53.
- Chen Z, Liu D, Wang J, et al. (2014). Development of nanoparticles-in-microparticles system for improved local retention after intra-articular injection. Drug Deliv 21:342–50.
- Chen Z-P, Liu W, Liu D, et al. (2012). Development of brucine-loaded microsphere/thermally responsive hydrogel combination system for intra-articular administration. J Control Release 162:628–35.
- Choi B, Kim S, Lin B, et al. (2014). Cartilaginous extracellular matrix-modified chitosan hydrogels for cartilage tissue engineering. ACS Appl Mater Interfaces 6:20110–21.
- Clutterbuck AL, Allaway D, Harris P, et al. (2013). Curcumin reduces prostaglandin E2, matrix metalloproteinase-3 and proteoglycan release in the secretome of interleukin 1beta-treated articular cartilage. F1000Res 2:147.
- Daraee H, Etemadi A, Kouhi M, et al. (2016). Application of liposomes in medicine and drug delivery. Artif Cells Nanomed Biotechnol 44:381–91.
- Ellman MB, An HS, Muddasani P, et al. (2008). Biological impact of the fibroblast growth factor family on articular cartilage and intervertebral disc homeostasis. Gene 420:82–9.
- Elsaid KA, Ubhe A, Shaman Z, et al. (2016). Intra-articular interleukin-1 receptor antagonist (IL1-ra) microspheres for posttraumatic osteoarthritis: in vitro biological activity and in vivo disease modifying effect. J Exp Orthop 3:18.
- Eswaramoorthy R, Chang C-C, Wu S-C, et al. (2012). Sustained release of PTH(1-34) from PLGA microspheres suppresses osteoarthritis progression in rats. Acta Biomater 8:2254–62.
- Evans CH, Ghivizzani SC, Robbins PD. (2018). Gene delivery to joints by intra-articular injection. Hum Gene Ther 29:2–14.
- Fazal-Ur-Rehman B, Karen A H, Hongsik C. (2016). Kartogenin induced chondrogenesis of stem cells and cartilage repair. Int J Stem Cell Res Ther 3:036.
- Fleming BC, Hulstyn MJ, Oksendahl HL, et al. (2005). Ligament injury, reconstruction and osteoarthritis. Curr Opin Orthop 16:354–62.
- Frank C, Shrive N. (1996). Ligament biology, repair, and transplantation. Curr Opin Orthop 7:50–6.
- Frisbie DD, McIlwraith CW, Kawcak CE, et al. (2009). Evaluation of topically administered diclofenac liposomal cream for treatment of horses with experimentally induced osteoarthritis. Am J Vet Res 70:210–5.
- Garcia JP, Stein J, Cai Y, et al. (2019). Fibrin-hyaluronic acid hydrogel-based delivery of antisense oligonucleotides for ADAMTS5 inhibition in co-delivered and resident joint cells in osteoarthritis. J Control Release 294:247–58.
- Glyn-Jones S, Palmer AJR, Agricola R, et al. (2015). Osteoarthritis. Lancet 386:376–87.
- Gomez-Gaete C, Retamal M, Chávez C, et al. (2017). Development, characterization and in vitro evaluation of biodegradable rhein-loaded microparticles for treatment of osteoarthritis. Eur J Pharm Sci 96:390–7.
- Gonzalez-Fernandez T, Tierney EG, Cunniffe GM, et al. (2016). Gene delivery of TGF-beta3 and BMP2 in an MSC-laden alginate hydrogel for articular cartilage and endochondral bone tissue engineering. Tissue Eng Part A 22:776–87.
- Goto N, Okazaki K, Akasaki Y, et al. (2017). Single intra-articular injection of fluvastatin-PLGA microspheres reduces cartilage degradation in rabbits with experimental osteoarthritis. J Orthop Res 35:2465–75.
- Grol MW, Lee BH. (2018). Gene therapy for repair and regeneration of bone and cartilage. Curr Opin Pharmacol 40:59–66.
- Hayder M, Poupot M, Baron M, et al. (2011). A phosphorus-based dendrimer targets inflammation and osteoclastogenesis in experimental arthritis. Sci Transl Med 3:81ra35.
- Hellio Le Graverand M-P, Vignon E, Otterness IG, et al. (2001a). Early changes in lapine menisci during osteoarthritis development: Part I: cellular and matrix alterations. Osteoarthritis Cartilage 9:56–64.
- Hellio Le Graverand M-P, Vignon E, Otterness IG, et al. (2001b). Early changes in lapine menisci during osteoarthritis development: Part II: molecular alterations. Osteoarthritis Cartilage 9:65–72.
- Henrotin Y, Clutterbuck AL, Allaway D, et al. (2010). Biological actions of curcumin on articular chondrocytes. Osteoarthritis Cartilage 18:141–9.
- Henrotin Y, Priem F, Mobasheri A. (2013). Curcumin: a new paradigm and therapeutic opportunity for the treatment of osteoarthritis: curcumin for osteoarthritis management. Springerplus 2:56.
- Hu Q, Ding B, Yan X, et al. (2017). Polyethylene glycol modified PAMAM dendrimer delivery of kartogenin to induce chondrogenic differentiation of mesenchymal stem cells. Nanomedicine 13:2189–98.
- Inoue A, Takahashi KA, Arai Y, et al. (2006). The therapeutic effects of basic fibroblast growth factor contained in gelatin hydrogel microspheres on experimental osteoarthritis in the rabbit knee. Arthritis Rheum 54:264–70.
- Jain A, Mishra SK, Vuddanda PR, et al. (2014). Targeting of diacerein loaded lipid nanoparticles to intra-articular cartilage using chondroitin sulfate as homing carrier for treatment of osteoarthritis in rats. Nanomedicine 10:1031–40.
- Janssen M, Timur UT, Woike N, et al. (2016). Celecoxib-loaded PEA microspheres as an auto regulatory drug-delivery system after intra-articular injection. J Control Release 244:30–40.
- Javed I, Hussain SZ, Shahzad A, et al. (2016). Lecithin-gold hybrid nanocarriers as efficient and pH selective vehicles for oral delivery of diacerein – in-vitro and in-vivo study. Colloids Surf B Biointerfaces 141:1–9.
- Jin P, Wiraja C, Zhao J, et al. (2017). Nitric oxide nanosensors for predicting the development of osteoarthritis in rat model. ACS Appl Mater Interfaces 9:25128–37.
- Jin R, Moreira Teixeira LS, Krouwels A, et al. (2010). Synthesis and characterization of hyaluronic acid-poly(ethylene glycol) hydrogels via Michael addition: an injectable biomaterial for cartilage repair. Acta Biomater 6:1968–77.
- Johnson K, Zhu S, Tremblay MS, et al. (2012). A stem cell-based approach to cartilage repair. Science 336:717–21.
- Kang ML, Jeong SY, Im GI. (2017). Hyaluronic acid hydrogel functionalized with self-assembled micelles of amphiphilic PEGylated kartogenin for the treatment of osteoarthritis. Tissue Eng Part A 23:630–9.
- Kang ML, Kim JE, Im GI. (2016). Thermoresponsive nanospheres with independent dual drug release profiles for the treatment of osteoarthritis. Acta Biomater 39:65–78.
- Kang ML, Ko J-Y, Kim JE, et al. (2014). Intra-articular delivery of kartogenin-conjugated chitosan nano/microparticles for cartilage regeneration. Biomaterials 35:9984–94.
- Kim SR, Ho MJ, Kim SH, et al. (2016). Increased localized delivery of piroxicam by cationic nanoparticles after intra-articular injection. Dddt 10:3779–87.
- Kim SR, Ho MJ, Lee E, et al. (2015). Cationic PLGA/Eudragit RL nanoparticles for increasing retention time in synovial cavity after intra-articular injection in knee joint. Int J Nanomedicine 10:5263–71.
- Kim TG, Park TG. (2006). Biomimicking extracellular matrix: cell adhesive RGD peptide modified electrospun poly(D,L-lactic-co-glycolic acid) nanofiber mesh. Tissue Eng 12:221–33.
- Ko J-Y, Choi Y-J, Jeong G-J, et al. (2013). Sulforaphane-PLGA microspheres for the intra-articular treatment of osteoarthritis. Biomaterials 34:5359–68.
- Kou L, Bhutia YD, Yao Q, et al. (2018a). Transporter-guided delivery of nanoparticles to improve drug permeation across cellular barriers and drug exposure to selective cell types. Front Pharmacol 9:27.
- Kou L, Hou Y, Yao Q, et al. (2018b). L-Carnitine-conjugated nanoparticles to promote permeation across blood–brain barrier and to target glioma cells for drug delivery via the novel organic cation/carnitine transporter OCTN2. Artif Cells Nanomed Biotechnol 46:1605–16.
- Kou L, Sun R, Bhutia YD, et al. (2018c). Emerging advances in P-glycoprotein inhibitory nanomaterials for drug delivery. Expert Opin Drug Deliv 15:869–79.
- Kou L, Sun R, Ganapathy V, et al. (2018d). Recent advances in drug delivery via the organic cation/carnitine transporter 2 (OCTN2/SLC22A5). Expert Opin Ther Targets 22:715–26.
- Kraus VB, Conaghan PG, Aazami HA, et al. (2018). Synovial and systemic pharmacokinetics (PK) of triamcinolone acetonide (TA) following intra-articular (IA) injection of an extended-release microsphere-based formulation (FX006) or standard crystalline suspension in patients with knee osteoarthritis (OA). Osteoarthritis Cartilage 26:34–42.
- Krebs MD, Salter E, Chen E, et al. (2010). Calcium phosphate-DNA nanoparticle gene delivery from alginate hydrogels induces in vivo osteogenesis. J Biomed Mater Res A 92:1131–8.
- Lajeunesse D, Reboul P. (2003). Subchondral bone in osteoarthritis: a biologic link with articular cartilage leading to abnormal remodeling. Curr Opin Rheumatol 15:628–33.
- Laroui H, Grossin L, Léonard M, et al. (2007). Hyaluronate-covered nanoparticles for the therapeutic targeting of cartilage. Biomacromolecules 8:3879–85.
- Larsen C, Østergaard J, Larsen SW, et al. (2008). Intra-articular depot formulation principles: role in the management of postoperative pain and arthritic disorders. J Pharm Sci 97:4622–54.
- Lawrence RC, Felson DT, Helmick CG, et al. (2008). Estimates of the prevalence of arthritis and other rheumatic conditions in the United States. Part II. Arthritis Rheum 58:26–35.
- Lee YK, Choi JK, Kang YJ, et al. (2016). Triamcinolone–carbon nanotube conjugation inhibits inflammation of human arthritis synovial fibroblasts. J Mater Chem B 4:1660–71.
- Leonard M, De Boisseson MR, Hubert P, et al. (2004). Hydrophobically modified alginate hydrogels as protein carriers with specific controlled release properties. J Control Release 98:395–405.
- Li G, Yin J, Gao J, et al. (2013). Subchondral bone in osteoarthritis: insight into risk factors and microstructural changes. Arthritis Res Ther 15:223.
- Lin J, Zhang W, Jones A, et al. (2004). Efficacy of topical non-steroidal anti-inflammatory drugs in the treatment of osteoarthritis: meta-analysis of randomised controlled trials. BMJ 329:324.
- Lin JB, Poh S, Panitch A. (2016). Controlled release of anti-inflammatory peptides from reducible thermosensitive nanoparticles suppresses cartilage inflammation. Nanomedicine 12:2095–100.
- Liu H, Slamovich EB, Webster TJ. (2005). Increased osteoblast functions on nanophase titania dispersed in poly-lactic-co-glycolic acid composites. Nanotechnology 16:S601–S8.
- Losina E, Paltiel AD, Weinstein AM, et al. (2015). Lifetime medical costs of knee osteoarthritis management in the United States: impact of extending indications for total knee arthroplasty. Arthritis Care Res (Hoboken) 67:203–15.
- Lu H, Dai Y, Lv L, et al. (2014). Chitosan-graft-polyethylenimine/DNA nanoparticles as novel non-viral gene delivery vectors targeting osteoarthritis. PLoS One 9:e84703.
- Lu H-D, Zhao H-Q, Wang K, et al. (2011). Novel hyaluronic acid-chitosan nanoparticles as non-viral gene delivery vectors targeting osteoarthritis. Int J Pharm 420:358–65.
- Lu YC, Evans CH, Grodzinsky AJ. (2011). Effects of short-term glucocorticoid treatment on changes in cartilage matrix degradation and chondrocyte gene expression induced by mechanical injury and inflammatory cytokines. Arthritis Res Ther 13:R142.
- Luo Y, Shen H, Fang Y, et al. (2015). Enhanced proliferation and osteogenic differentiation of mesenchymal stem cells on graphene oxide-incorporated electrospun poly(lactic-co-glycolic acid) nanofibrous mats. ACS Appl Mater Interfaces 7:6331–9.
- Madry H, Cucchiarini M. (2016). Gene therapy for human osteoarthritis: principles and clinical translation. Expert Opin Biol Ther 16:331–46.
- Massaro M, Buscemi G, Arista L, et al. (2019). Multifunctional carrier based on *halloysite/laponite hybrid hydrogel for kartogenin delivery. ACS Med Chem Lett 10:419–24.
- Matsuzaki T, Matsushita T, Tabata Y, et al. (2014). Intra-articular administration of gelatin hydrogels incorporating rapamycin-micelles reduces the development of experimental osteoarthritis in a murine model. Biomaterials 35:9904–11.
- Maudens P, Meyer S, Seemayer CA, et al. (2018). Self-assembled thermoresponsive nanostructures of hyaluronic acid conjugates for osteoarthritis therapy. 10:1845–54.
- Maudens P, Seemayer CA, Pfefferlé F, et al. (2018). Nanocrystals of a potent p38 MAPK inhibitor embedded in microparticles: Therapeutic effects in inflammatory and mechanistic murine models of osteoarthritis. J Control Release 276:102–12.
- McAlindon TE, Bannuru RR, Sullivan MC, et al. (2014). Response to Letter to the Editor entitled “Comments on 'OARSI guidelines for the non-surgical management of knee osteoarthritis'“. Osteoarthritis Cartilage 22:890–1.
- McMasters J, Poh S, Lin JB, et al. (2017). Delivery of anti-inflammatory peptides from hollow PEGylated poly(NIPAM) nanoparticles reduces inflammation in an ex vivo osteoarthritis model. J Control Release 258:161–70.
- Mishra S, De A, Mozumdar S. (2014). Synthesis of thermoresponsive polymers for drug delivery. Methods Mol Biol 1141:77–101.
- Morgen M, Tung D, Boras B, et al. (2013). Nanoparticles for improved local retention after intra-articular injection into the knee joint. Pharm Res 30:257–68.
- Morille M, Toupet K, Montero-Menei CN, et al. (2016). PLGA-based microcarriers induce mesenchymal stem cell chondrogenesis and stimulate cartilage repair in osteoarthritis. Biomaterials 88:60–9.
- Morille M, Van-Thanh T, Garric X, et al. (2013). New PLGA-P188-PLGA matrix enhances TGF-beta3 release from pharmacologically active microcarriers and promotes chondrogenesis of mesenchymal stem cells. J Control Release 170:99–110.
- Mueller MB, Tuan RS. (2011). Anabolic/catabolic balance in pathogenesis of osteoarthritis: identifying molecular targets. Pm r 3:S3–S11.
- Natarajan V, Krithica N, Madhan B, et al. (2011). Formulation and evaluation of quercetin polycaprolactone microspheres for the treatment of rheumatoid arthritis. J Pharm Sci 100:195–205.
- Navarro-Sarabia F, Coronel P, Collantes E, et al. (2011). A 40-month multicentre, randomised placebo-controlled study to assess the efficacy and carry-over effect of repeated intra-articular injections of hyaluronic acid in knee osteoarthritis: the AMELIA project. Ann Rheum Dis 70:1957–62.
- Niazvand F, Khorsandi L, Abbaspour M, et al. (2017). Curcumin-loaded poly lactic-co-glycolic acid nanoparticles effects on mono-iodoacetate -induced osteoarthritis in rats. Vet Res Forum 8:155–61.
- Nüesch E, Dieppe P, Reichenbach S, et al. (2011). All cause and disease specific mortality in patients with knee or hip osteoarthritis: population based cohort study. BMJ 342:d1165.
- Oliveira RB, Nascimento TL, Lima EM. (2012). Design and characterization of sustained release ketoprofen entrapped carnauba wax microparticles. Drug Dev Ind Pharm 38:1–11.
- Oprenyeszk F, Chausson M, Maquet V, et al. (2013). Protective effect of a new biomaterial against the development of experimental osteoarthritis lesions in rabbit: a pilot study evaluating the intra-articular injection of alginate-chitosan beads dispersed in an hydrogel. Osteoarthritis Cartilage 21:1099–107.
- Patterson J, Siew R, Herring SW, et al. (2010). Hyaluronic acid hydrogels with controlled degradation properties for oriented bone regeneration. Biomaterials 31:6772–81.
- Pelletier J-P, Martel-Pelletier J, Rannou F, et al. (2016). Efficacy and safety of oral NSAIDs and analgesics in the management of osteoarthritis: evidence from real-life setting trials and surveys. Semin Arthritis Rheum 45:S22–S7.
- Pi Y, Zhang X, Shao Z, et al. (2015). Intra-articular delivery of anti-Hif-2alpha siRNA by chondrocyte-homing nanoparticles to prevent cartilage degeneration in arthritic mice. Gene Ther 22:439–48.
- Poh S, Lin JB, Panitch A. (2015). Release of anti-inflammatory peptides from thermosensitive nanoparticles with degradable cross-links suppresses pro-inflammatory cytokine production. Biomacromolecules 16:1191–200.
- Rannou F, Poiraudeau S. (2010). Non-pharmacological approaches for the treatment of osteoarthritis. Best Pract Res Clin Rheumatol 24:93–106.
- Rey-Rico A, Venkatesan JK, Schmitt G, et al. (2017). rAAV-mediated overexpression of TGF-beta via vector delivery in polymeric micelles stimulates the biological and reparative activities of human articular chondrocytes in vitro and in a human osteochondral defect model. Int J Nanomed 12:6985–96.
- Rudnik-Jansen I, Colen S, Berard J, et al. (2017). Prolonged inhibition of inflammation in osteoarthritis by triamcinolone acetonide released from a polyester amide microsphere platform. J Control Release 253:64–72.
- Saadat E, Shakor N, Gholami M, et al. (2015). Hyaluronic acid based micelle for articular delivery of triamcinolone, preparation, in vitro and in vivo evaluation. Int J Pharm 489:218–25.
- Sacchetti C, Liu-Bryan R, Magrini A, et al. (2014). Polyethylene-glycol-modified single-walled carbon nanotubes for intra-articular delivery to chondrocytes. ACS Nano 8:12280–91.
- Sandell LJ, Aigner T. (2001). Articular cartilage and changes in arthritis. An introduction: cell biology of osteoarthritis. Arthritis Res 3:107–13.
- Schumacher HR, Chen LX. (2005). Injectable corticosteroids in treatment of arthritis of the knee. Am J Med 118:1208–14.
- Simon TM, Jackson DW. (2018). Articular cartilage: injury pathways and treatment options. Sports Med Arthrosc Rev 26:31–9.
- Sivan S, Schroeder A, Verberne G, et al. (2010). Liposomes act as effective biolubricants for friction reduction in human synovial joints. Langmuir 26:1107–16.
- Subramanian A, Vu D, Larsen GF, et al. (2005). Preparation and evaluation of the electrospun chitosan/PEO fibers for potential applications in cartilage tissue engineering. J Biomater Sci Polym Ed 16:861–73.
- Sulistio A, Mansfeld FM, Reyes-Ortega F, et al. (2019). Intra-articular treatment of osteoarthritis with diclofenac-conjugated polymer reduces inflammation and pain. ACS Appl Bio Mater 2:2822.
- Sun J, Gooch K, Svenson LW, et al. (2007). Estimating osteoarthritis incidence from population-based administrative health care databases. Ann Epidemiol 17:51–6.
- Suto B, Berkó S, Kozma G, et al. (2016). Development of ibuprofen-loaded nanostructured lipid carrier-based gels: characterization and investigation of in vitro and in vivo penetration through the skin. Int J Nanomede 11:1201–12.
- Tetlow LC, Adlam DJ, Woolley DE. (2001). Matrix metalloproteinase and proinflammatory cytokine production by chondrocytes of human osteoarthritic cartilage: associations with degenerative changes. Arthritis Rheum 44:585–94.
- Tsukuda Y, Onodera T, Ito M, et al. (2015). Therapeutic effects of intra-articular ultra-purified low endotoxin alginate administration on an experimental canine osteoarthritis model. J Biomed Mater Res A 103:3441–8.
- Varadi G, Zhu Z, Blattler T, et al. (2013). Randomized clinical trial evaluating transdermal Ibuprofen for moderate to severe knee osteoarthritis. Pain Physician 16:E749–62.
- Wang Z, Guo W, Kuang X, et al. (2017). Nanopreparations for mitochondria targeting drug delivery system: current strategies and future prospective. Asian J Pharm Sci 12:498–508.
- Whitmire RE, Scott Wilson D, Singh A, et al. (2012). Self-assembling nanoparticles for intra-articular delivery of anti-inflammatory proteins. Biomaterials 33:7665–75.
- Williams AS, Camilleri JP, Goodfellow RM, et al. (1996). A single intra-articular injection of liposomally conjugated methotrexate suppresses joint inflammation in rat antigen-induced arthritis. Br J Rheumatol 35:719–24.
- Wright LD, McKeon-Fischer KD, Cui Z, et al. (2014). PDLA/PLLA and PDLA/PCL nanofibers with a chitosan-based hydrogel in composite scaffolds for tissue engineered cartilage. J Tissue Eng Regen Med 8:946–54.
- Xia C, Chen P, Mei S, et al. (2017). Photo-crosslinked HAMA hydrogel with cordycepin encapsulated chitosan microspheres for osteoarthritis treatment. Oncotarget 8:2835–49.
- Yang S, Kim J, Ryu J-H, et al. (2010). Hypoxia-inducible factor-2alpha is a catabolic regulator of osteoarthritic cartilage destruction. Nat Med 16:687–93.
- Yao Q, Choi JH, Dai Z, et al. (2017). Improving tumor specificity and anticancer activity of dasatinib by dual-targeted polymeric micelles. ACS Appl Mater Interfaces 9:36642–54.
- Yao Q, Kou L, Tu Y, et al. (2018). MMP-responsive 'Smart' drug delivery and tumor targeting. Trends Pharmacol Sci 39:766–81.
- Yao Q, Dai ZW, Choi JH, et al. (2017). Building stable MMP2-responsive multifunctional polymeric micelles by an all-in-one polymer–lipid conjugate for tumor-targeted intracellular drug delivery. ACS Appl Mater Interfaces 9:32520–33.
- Yeh C-C, Su Y-H, Lin Y-J, et al. (2015). Evaluation of the protective effects of curcuminoid (curcumin and bisdemethoxycurcumin)-loaded liposomes against bone turnover in a cell-based model of osteoarthritis. Drug Des Dev Ther 9:2285–300.
- Zabihi F, Xin N, Li S, et al. (2014). Polymeric coating of fluidizing nano-curcumin via anti-solvent supercritical method for sustained release. J Supercrit Fluids 89:99–105.
- Zhang JX, Yan MQ, Li XH, et al. (2007). Local delivery of indomethacin to arthritis-bearing rats through polymeric micelles based on amphiphilic polyphosphazenes. Pharm Res 24:1944–53.
- Zhang X, Yu C, Xushi, et al. (2006). Direct chitosan-mediated gene delivery to the rabbit knee joints in vitro and in vivo. Biochem Biophys Res Commun 341:202–8.
- Zhang Z, Bi X, Li H, et al. (2011). Enhanced targeting efficiency of PLGA microspheres loaded with Lornoxicam for intra-articular administration. Drug Deliv 18:536–44.
- Zhang Z, Huang G. (2012). Intra-articular lornoxicam loaded PLGA microspheres: enhanced therapeutic efficiency and decreased systemic toxicity in the treatment of osteoarthritis. Drug Deliv 19:255–63.
- Zhang Z, Leong DJ, Xu L, et al. (2016). Curcumin slows osteoarthritis progression and relieves osteoarthritis-associated pain symptoms in a post-traumatic osteoarthritis mouse model. Arthritis Res Ther 18:128.
- Zhao L, Liu M, Wang J, et al. (2015). Chondroitin sulfate-based nanocarriers for drug/gene delivery. Carbohydr Polym 133:391–9.
- Zhao X, Yu S-B, Wu F-L, et al. (2006). Transfection of primary chondrocytes using chitosan-pEGFP nanoparticles. J Control Release 112:223–8.
- Zhao Y, Wei C, Chen X, et al. (2019). Drug delivery system based on near-infrared light-responsive molybdenum disulfide nanosheets controls the high-efficiency release of dexamethasone to inhibit inflammation and treat osteoarthritis. ACS Appl Mater Interfaces 11:11587–601.
- Zhou Y, Liu S-q, Peng H, et al. (2015). In vivo anti-apoptosis activity of novel berberine-loaded chitosan nanoparticles effectively ameliorates osteoarthritis. Int Immunopharmacol 28:34–43.