ABSTRACT
A quantitative weight of evidence (QWoE) methodology was used to assess higher-tier studies on the effects of imidacloprid (IMI) on honeybees. Assessment endpoints were population size and viability of commercially managed bees and quantity of hive products. A colony-level no-observed-adverse effect concentration (NOAEC) of 25 µg IMI/kg syrup, equivalent to an oral no-observed-adverse-effect-dose of 7.3 ng/bee/d for all responses, was measured. The overall weight of evidence indicates that there is minimal risk to honeybees from exposure to IMI from its use as a seed treatment. Exposures via dusts from currently used seed coatings present a de minimis risk to honeybees when the route of exposure is via uptake in plants that are a source of pollen or nectar for honeybees. There were few higher-tier observational (ecoepidemiological) studies conducted with IMI. Considering all lines of evidence, the quality of the studies included in this analysis was variable, but the results of the studies were consistent and point to the same conclusion – that IMI had no adverse effects on viability of the honeybee colony. Thus, the overall conclusion is that IMI, as currently used as a seed treatment and with good agricultural practices, does not present a significant risk to honeybees at the level of the colony.
Introduction and problem formulation
This paper is the second in a series of quantitative weight of evidence (QWoE) analyses on the effects and risks of the use of neonicotinoids as seed treatments on honeybees. The methodology used for the QWoE analysis has been described in the first paper in this series (Solomon and Stephenson Citation2017) and this paper reports on a QWoE of the effects and risks of the neonicotinoid imidacloprid (IMI). IMI was first registered in 1992 for use on cotton, rice, cereals, peanuts, potatoes, vegetables, pome fruits, pecans, and turf-grass (Fairbrother et al. Citation2014). It is effective against sucking and soil-dwelling insects, whiteflies, termites, turf insects, and Colorado potato beetle (Fairbrother et al. Citation2014). Although in a state of regulatory flux, the major current use of IMI in areas without restrictions (i.e., the EU) is as a seed treatment. Here, the product might be mixed with other pesticides, such as fungicides, and coated on the seed with binders and other materials that provide a hard coating that does not abrade and reduces release of the coating as dust during handling and, more importantly, pneumatic seeding (drilling). The use of IMI, at least in the United Kingdom, had declined from a high of about 40,000 kg in 2005 to about 8,000 kg in 2011, largely because of the greater use of other neonicotinoids (Goulson Citation2013). Data on changes in global use were not available.
Physical, chemical, and toxicological properties of IMI
IMI, CAS RN [138261–41-3], IUPAC name 1-(6-chloro-3-pyridylmethyl)-N-nitroimidazolidin-2-ylideneamine (), is a solid at room temperature and has a low vapor pressure of 4 x10−7 mPa (20°C); 9 x10−7 mPa (25°C), and its Hc is 2 x 10–10 Pa m3 mol−1 (20°C, calc.) (BCPC Citation2015). Because the vapor pressure is low and Henry’s constant small, IMI is unlikely to be present in air at concentrations sufficiently large to be of toxicological concern. Solubility in water is 610 mg/L, the log KOW is 0.57 (21°C), and the KOC is 249–336 (BCPC Citation2015), so it is relatively mobile in soil pore water and can be readily taken up by the roots of plants. It is stable to hydrolysis at environmentally relevant pH (5–11), which means that it is sufficiently stable to be efficacious as a soil-active insecticide. Generally, for a period after planting, IMI is readily available for uptake by plants. Once in the plant, it can be translocated from the roots to other parts of the plant. IMI is metabolized in plants to two active metabolites, IMI-5-hydroxy (IMI-OH) and IMI-olefin (IMI=), which are structurally similar () and have similar toxic potency (EFSA Citation2013). The parent material and these two metabolites are referred to by their abbreviations in this paper.
Toxicity
As for all neonicotinoid insecticides, IMI acts by binding to the insect nicotinic acetylcholine receptor (nAChR) and acts as an agonist (Jeschke et al. Citation2011). The companion paper (Solomon and Stephenson Citation2017) presents a generic conceptual model for exposure of honeybees to neonicotinoids. This assessment of IMI was based on that conceptual model.
The oral toxicity of IMI in first-tier laboratory studies was summarized by EFSA and the acute oral LD50 was reported to range from 3.7 to >70.3 ng/bee (EFSA Citation2008). In a more recent conclusion report (EFSA Citation2013), the first-tier acute oral toxicity value of the active substance in the honeybee selected by EFSA was 3.7 ng/bee and the no-observed-adverse effect dose (NOAED) for acute toxicity was 1.2 ng/bee. The NOAED for foraging behavior reported by EFSA (Citation2013) was 1.5 ng/bee and the chronic NOAED also selected by EFSA was based on survivorship and was 0.13 ng/bee. These toxicity values are appropriate for use as an initial screening value for characterizing risk from oral exposures, but are less useful for assessing responses in higher-tier field studies that better measure apical endpoints directly. Contact toxicity of IMI is unlikely to be relevant; the acute contact toxicity of IMI to bees was reported as 81 ng/bee (EFSA Citation2013), much greater than the oral toxicity value.
The focus of this QWoE analysis was on responses of honeybees to IMI under more realistic semi-field and field conditions. For this reason, toxicity values derived from higher-tier studies that assessed effects on the colony were chosen as the most appropriate measures of effect. Toxicity data for higher-tier assessment were provided in a robust whole-hive feeding-exposure study conducted over a period of 42 d (Bayer CropScience Citation2014h). This study exposed bees via sugar syrup and, in addition to toxicity measures in individual bees, additional colony-relevant responses for survival and productivity were measured (see supplemental information (SI)). The concentrations in the syrup in this study can be used to estimate doses in bees that are based on the default assumptions of intake of food used by the USEPA (Citation2014), see below. The above study reported a no-observed-adverse effect concentration (NOAEC) of 25 µg IMI/kg of syrup, equivalent to an oral no-observed-adverse effect-dose (NOAED; 7.3 ng/bee/d) for all responses measured (). The lowest-observed-adverse-effect-concentration (LOAEC) was 50 µg IMI/L, equivalent to an oral lowest observed-adverse effect-dose (LOAED) of 14.6 ng/bee/d. These toxicity values were used in this paper for assessing the exposures of bees to IMI measured in higher-tier field studies and are expressed in doses per bee to allow normalization from various sources of exposure.
Table 1. Colony-level effect measures for IMI from Bayer CropScience (Citation2014h).
In a whole-colony 2-y study (Dively et al. Citation2015), where honeybees were fed a supplemental diet of pollen containing IMI over multiple brood cycles, the authors reported no effects in the first year (2009) on foraging activity or colony performance metrics during and shortly after 12 weeks of exposure to concentrations up to 100 μg IMI/kg pollen. Overwintering performance was also unaffected in the first year of the study. In the second year of the study (2010), the hives in the control treatment had experienced some queen losses, with a subsequent temporary decrease in brood production in midsummer. There was no significant effect on overwintering performance (p = 0.21). The loss of queens in 2010 resulted in lower numbers of capped brood cells in the control, but there was no concentration response in the IMI-exposed bees (authors’ ); the NOAEC for brood development was >100 μg IMI/kg pollen. It should be noted that the authors concluded that their study demonstrated that exposure of honeybees to conservative estimates of dietary concentrations (≤10 µg IMI/kg pollen) would result in no adverse effects on honeybee colony health. This study had several weaknesses (see SI) and was judged to be unsuitable for a definitive characterization of risks in this WoE, but the results do suggest that exposures to IMI via pollen are small in relation to the estimated toxicity values. In a more recent study (Wu-Smart and Spivak Citation2016), the effects of IMI on several colony-level endpoints were reported. The study had several weaknesses (two major) and the QoM was 0.65 (see SI). The authors used colonies of 1500, 3000, and 7000 bees and conducted the work over three years. Overall, the authors reported that colony size affected the severity of the responses observed in the study. The major weaknesses rendered the study unsuitable for the characterization of toxicity values at the level of the colony. The duration of the observations (3 weeks) was too short in relation to the lifespan of worker bees (5–6 weeks), so effects might have been missed. In addition, the exposures were compromised because the bees were not provided with sufficient syrup to satisfy their needs. Thus, the exposures from the treated syrup were likely diluted by nectar collected outside the hive and dose per bee cannot be calculated.
Figure 2. Strengths and relevance of exposure values from controlled field studies with IMI-treated seeds and mixed treatments (where application methods were not defined). Number of responses assessed = 86. Symbols may obscure others, see SI for all responses. There were no data points obscured by the legend.
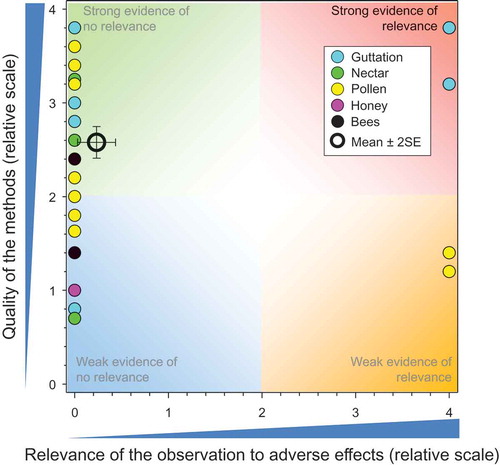
Figure 3. Strength and relevance of effects in honeybees exposed in controlled field studies via crops treated with IMI as seed treatments. Number of responses assessed = 85. Symbols may obscure others, see SI for all responses. There were no data points obscured by the legend.
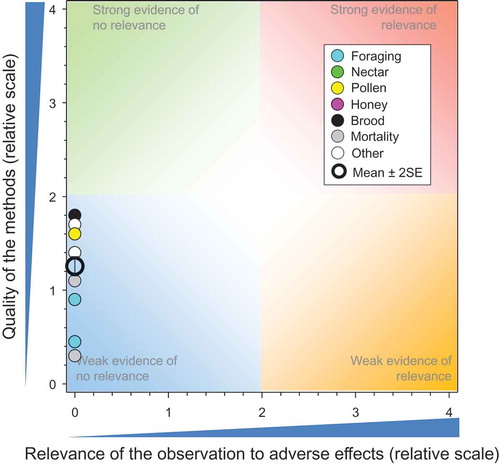
Figure 4. Strengths and relevance of effects observed in honeybees exposed in controlled field studies to IMI via dust and carryover of residues. Number of responses assessed = 14. Symbols may obscure others, see SI for all responses. There were no data points obscured by the legend.
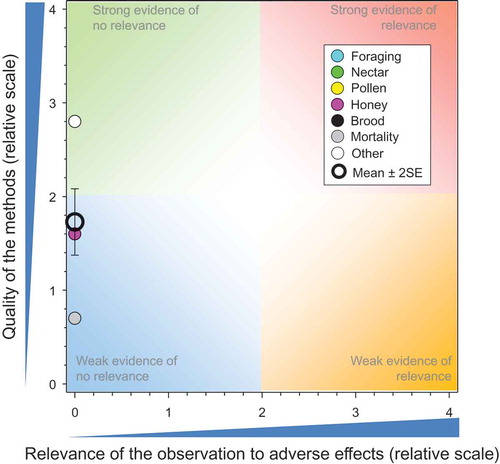
Figure 5. Strengths and relevance of ecoepidemiology, higher-tier observational studies conducted on IMI. Number of responses assessed = 32. Symbols may obscure others, see SI for all responses. There were no data points obscured by the legend.
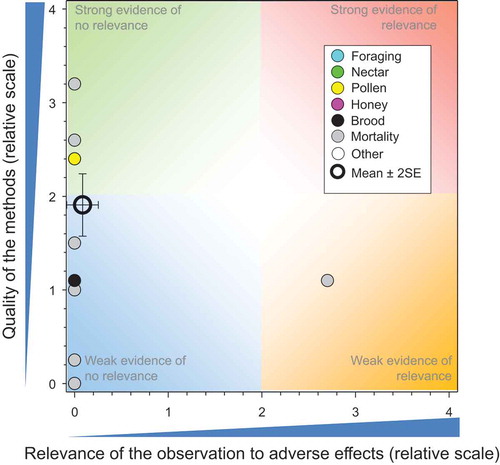
EFSA concluded that the biologically active metabolites of IMI, IMI-OH, and IMI= are of similar toxicity to the parent IMI. For the purposes of the WoE assessment, where concentrations of active metabolites were measured, they were added to those of the IMI and the concentration was expressed as ΣIMI. It was assumed that larvae were as sensitive to IMI as the adults. However, this is a conservative assumption; physiological responses such as behavior in the developing larva would likely be less relevant to apical endpoints than in adults. Degradation of IMI via hydrolysis in water is slow; thus, IMI present in pollen, nectar and/or honeydew, and water carried to the hive is not expected to degrade rapidly. Thus, concentrations in these matrices are expected to be representative of what bees are exposed to except for guttation fluid collected as “water”.
The concentrations in the food in these studies can be used to estimate doses in honeybees that are based on the assumptions of intake of food used by the USEPA (Citation2014), see .
Table 2. Threshold concentration in food and water for toxicity values for IMI in honeybees.
The main null hypothesis being tested is that treatment of seeds with IMI has no negative impacts on colony viability and no adverse effects on survival of the colony. There are several sub-hypotheses addressed below:
Concentrations of IMI and toxic metabolites in bee-relevant matrices from seed-treated crops in the field do not exceed toxicity values derived from higher-tier toxicity studies on colonies of honeybees.
Colonies of honeybees exposed under experimental conditions in the field to IMI from treated seeds do not show adverse effects.
Exposure of colonies of honeybees to measured concentrations of IMI resulting from use as a seed treatment in open agricultural fields does not cause adverse effects.
Methods
Sources of information used in this QWoE assessment
The source of studies, papers, and reports used in this QWoE analysis and the methods used to assess quality and relevance of the data have been described in the first paper in the series (Solomon and Stephenson Citation2017). Reviews and papers lacking sufficient information to allow for assessment of quality and relevance were not included in the analysis (see discussion in Solomon and Stephenson Citation2017). Other than these, we included all papers and reports on higher-tier studies conducted at the level of the colony and under realistic field conditions. Papers and reports were not excluded based on quality.
The methods used to score the papers and reports for quality and relevance and how these scores were used to produce the QWoE diagrams in this paper are also provided in the text and SI in Solomon and Stephenson (Citation2017). These methods are common to this and two other companion papers and are not discussed in detail here.
Characterization of exposures via surface water
There were several reports of concentrations of IMI measured in surface waters. These studies were monitoring studies or surveys, the results of which were not coupled to higher-tier assessment of effects. These studies were from the open literature and were not specifically included in the QWoE analysis; however, the results are summarized here. The risks of the reported concentrations were assessed by comparison to the exposures that might be expected from the collection of water or guttation fluids (see below). Where centiles of exposure were reported, or could be calculated from the raw data, 90th and other upper centiles were compared to the toxicity values.
In a study of an urban watershed, the maximum concentration of IMI detected in the Kisco River (NY, USA) in 2000–2001 was 0.13 μg/L (n = 10; level of quantitation (LOQ) = 0.106 µg/L) (Phillips and Bode Citation2004). Concentrations in surface waters draining agricultural watersheds were greater; in 2010–2011 in California, the maximum measured concentration of IMI was 3.29 μg/L (n = 75; LOQ = 0.01 µg/L) (Starner and Goh Citation2012). Maximum concentrations of IMI measured between 2008 and 2011 in surface waters in urban areas of California were ≤0.16 µg/L (n = 45; LOQ = 0.05 µg/L) (Ensminger et al. Citation2013).
An analysis of the measured concentrations of IMI in surface waters in the Netherlands (NL) for 2009–2011 showed that the 95th centile concentrations were <1 μg/L; however, the maximum measured value was ≈ 20 μg/L (from in Vijver and Van Den Brink Citation2014).
In a survey, concentrations of IMI in Prairie potholes and meltwater samples were measured in samples taken four times between 2012 and 2013 in Saskatchewan. The maxim concentration was 0.256 µg/L (Main et al. Citation2014). The frequency of detection ranged from 8 to 0% (LOQ = 0.0011 µg/L). In a second study (Main et al. Citation2016), IMI was analyzed for but detections were not reported (LOQ = 0.0023 µg/L); they were all presumed to be non-detects. In a third study (Main et al. Citation2017), the effects of macrophytes on concentrations of neonicotinoids in Prairie potholes were investigated. The maximum concentration of IMI detected was 0.007 µg/L and the LOQ was 0.003 µg/L. Raw data were not reported for any of the studies, so it was not possible to estimate centiles.
In a study conducted in Ontario (Struger et al. Citation2017), IMI was detected in 13 of 15 drains, creeks, and rivers at frequencies of almost 100%. Of the total of 440 samples, the median value from individual sites ranged from 0.585 to <0.0013 µg/L (the latter was assumed to be the MDL; recovery was not reported). The maximum value measured was 10.4 µg/L. Samples were collected every two weeks through the growing season (May to September), with monthly sampling in April, October, November, and December. Analysis was by tandem mass spectrometer using an electrospray ionization (ESI) source in positive ion mode. Isotopically labeled internal standard of IMI was added after the concentration of the residues on an SPE cartridge, so the actual extraction efficiency of each sample could not be determined. Field blanks were all less than the MDL. Of the total of 440 samples, the median values from individual sites ranged from 0.585 to <0.0013 µg/L. The median across all sites was 0.006 µg/L and the maximum value measured was 10.4 µg/L. In another study in S. Ontario, sources of water in or close to maize fields (puddles, ditches, and drains ≤100 m from the edge of the field) that might be attractive to nearby beehives were surveyed, sampled weekly from 29 April to 28 June 2013, and analyzed for the presence of neonicotinoids (Schaafsma et al. Citation2015). Samples were analyzed using the QuEChERS (quick, easy, cheap, effective, rugged and safe) methodology and quantified with liquid chromatography tandem mass spectroscopy (LC-MS/MS) using isotopically labeled internal standards (>99% pure). For IMI in water, the LOQ was 0.05 μg/L, mean recovery was 79%, but relative standard deviation (RSD) was not reported in the paper. Raw data were provided and centiles could be calculated. Median and 90th centile concentration of IMI in surface water potentially attractive to bees were <0.05 μg/L μg/L (data from Schaafsma et al. Citation2015, SI Table 2, n = 120). The Ontario Ministry of Environment and Climate Change (OMECC) measured concentrations of IMI in surface waters in the major use-areas in S. Ontario (OMECC Citation2017). Samples were collected at five sites (Big Creek, Garvey Glenn, Little Ausable Creek, North Creek, and White Ash Creek) in 2015, either during normal flow or during precipitation events. Analyses were conducted at the OMECC laboratories and the MDL was 0.005 µg/L. The median for all sites (n = 149) was ≤MDL and the maximum measured concentration was 2.3 µg/L, sampled during a precipitation event.
A paper reported on the concentrations of neonicotinoids in surface waters in the United States (Hladik and Kolpin Citation2016). The report was based on one-time samples collected in 38 streams across 24 US states and Puerto Rico between November 2012 and June 2014. Analysis followed the USGS protocols with appropriate blanks and control samples and surrogate standards. IMI was detected above the MDL (0.004–0.006 µg/L) in 37% of the samples, the 90th centile was 0.014 µg/L, and the maximum concentration was 0.042 µg/L. Temporal studies in the selected streams showed peak concentrations in the spring and summer months, but the maximum concentrations observed were less than the overall maximum.
Concentrations of IMI measured in surface waters were reported by the National Water Quality Monitoring Council (NWQMC) of the United States (NWQMC Citation2016). This database was queried for concentrations of IMI in surface water and the data were downloaded. The data were processed to exclude duplicates, preliminary results, analyses where storage temperatures were not met, where holding times were exceeded, and where calibration and QA/QC criteria were not met. Total and dissolved concentrations were treated as equivalent. Only the data where the MDL was 0.02–0.1 μg/L were included and all non-detects were assigned a dummy value of zero. For samples of surface waters collected between March 9, 1999 and November 12, 2015, the median and the 90th centiles were <MDL, and the 99th centile was 0.25 μg/L. Data from winter samples were not excluded as bees might be active in the southern US in winter.
Results and discussion
Risks from exposures via surface waters
To assess risks, dose per honeybee was estimated as described for guttation fluid (below and SI). In all cases, the estimated dose of IMI was less than the chronic NOAED and no acute risks were identified ().
Table 3. Estimated doses of IMI in honeybees collecting surface water from various sources.
Risks to honeybees from exposures resulting from uptake and translocation of IMI from seed treatments
There were 31 studies (Bargańska, Ślebioda, and Namieśnik Citation2013; Bayer Corporation Citation2002; Bayer CropScience Citation1999b; Citation1999c, Citation1999d, Citation1999e, Citation1999a, Bayer CropScience AG Citation2010; Bayer CropScience Citation2011a; Citation2011b, Citation2012a; Citation2012b, Citation2014i; Citation2014j, Citation2014k, Bayer CropScience AG Citation2014; Bayer CropScience Citation2014a; Citation2014b, Bayer CropScience AG Citation2015a; Bayer CropScience Citation2015a; Citation2015b, Bernal et al. Citation2010; Botías et al. Citation2015, Chauzat et al. Citation2006, Citation2011; Kasiotis et al. Citation2014; Laurent and Rathahao Citation2003; Mullin et al. Citation2010; Pohorecka et al. Citation2012; Reetz et al. Citation2011; Stewart et al. Citation2014) that measured potential exposures of honeybees to IMI resulting from use as a seed treatment and these resulted in 86 responses (see SI). The results of the comparisons of exposures from bee-relevant matrices measured in controlled field studies with IMI-treated seeds and mixed treatments (where application methods were not defined or known) to toxicity values are shown in . There are multiple observations that have the same scores for quality and relevance; these are separated for easier identification in the SI.
All but five of the observations showed concentrations less than the NOAED for honeybees of 7.3 ng IMI/bee/d. One of these was a measurement from pollen from a study by Mullin et al. (Citation2010). This was the maximum value from 350 samples, 10 of which had concentrations above the LOD. This sample also contained large concentrations of the metabolites IMI-OH and IMI=. The score for quality of the methods (QoM) for this paper was 1.2 and it is not known whether this is an outlier or not or the result of contamination. Another value for pollen was from a survey of residues in pollen and honey from hives where deaths of honeybees had been reported in Greece (Kasiotis et al. Citation2014). Two of 14 samples of pollen had concentrations >NOAED. Both were from the same region (Attica) but were sampled in different years. Three values that exceeded the NOAED were from guttation fluid. One was the 90th centile concentration in guttation fluid collected in autumn from a strong study (QoM = 3.8) on plants grown from IMI-treated seeds of winter barley (Bayer CropScience Citation2014d). Concentrations in guttation fluid collected in the following spring were less than the toxicity value and had no relevance to adverse effects. Another was a study with winter barley (Bayer CropScience Citation2012b) where the 90th centile concentration in guttation fluids exceeded the NOAED in autumn but not in spring. The third exceedance of NOAED in guttation fluid also was in a study with winter barley and this was observed only in autumn.
Some studies were of better quality than others and the overall mean for QoM was 2.58 ± SE of 0.08. The mean and SE for relevance were 0.23 ± 0.10, suggesting that there was a consistent lack of relevant effects in studies that were generally strong. The overall weight of evidence suggests that there is little or no risk to honeybees from exposure to concentrations of IMI in bee-relevant matrices resulting from its use as a seed treatment.
Risks to honeybees resulting from exposure to dusts containing IMI
Three higher-tier studies addressed dispersion of and exposure to dust generated during the planting of seeds treated with IMI. A WoE diagram for these studies was not generated. Two studies by the same group in Italy (Girolami et al. Citation2013, Citation2012) measured concentrations in and on honeybees at various distances from a pneumatic seed drill used for sowing treated seeds of corn. It was not clear whether the formulation used (Gaucho 350FS® Bayer CropScience AG) was a legacy product or a newer one developed to reduce drift of active substance (a.s.) on dust during seeding. Honeybees in cages were used as deposition collectors and the total amount of IMI on and in the honeybees was measured. These studies had major weaknesses related to lack of proper storage of the samples and that external residues were not separated from the actual body dose in the honeybee for analysis (QoM = 0.45 and 0.25, respectively). Some of the exposures were large (up to 3500 ng/bee) but cannot be compared to a toxicity value as no data were available for the combined oral and topical route of exposure.
The third study (Bayer CropScience Citation2014a) was more robust (QoM = 1.9) and based on analysis of a large number of samples (1,323) collected on petri dishes placed around the test sites. Of the 1,323 usable samples, 95.0% contained no quantifiable residues. Of the 66 samples containing quantifiable residues of IMI, 65 were taken at the time of sowing and one during the 24-h post-sowing period. Of these samples, 63 were associated with pneumatic sowing and three with mechanical sowing. The maximum measured residue deposited was equivalent to 0.111 g a.s./ha, collected in one sample 1 m from the edge of the field during sowing. The deposition amounts cannot be directly compared to oral toxicity values for IMI; however, the maximum measured deposition rate was 0.11 g IMI/ha, about 5000-fold less than the foliar rate of application (390–400 g a.s./ha) to cotton that resulted in median concentrations in nectar and pollen that did not exceed the NOAED (Bayer CropScience Citation2014a). This indicates that dust from currently used seed coatings presents a de minimis risk to honeybees via uptake in plants that are a source of pollen or nectar for honeybees.
Effects of IMI on honeybees from use as a seed treatment
The studies for which effects of IMI on honeybees were reported were from field experiments where seeds were treated with IMI and potential exposure of honeybees occurred while honeybees were foraging for pollen and nectar or guttation fluid from plants with systemic residues (Bayer CropScience Citation1998; Citation1999b; Citation1999c, Citation1999d, Citation1999e, Citation1999a, Citation2002, Citation2007, Citation2012c; Citation2012b, Citation2014f; Citation2014b, Citation2014c, Citation2014d, Citation2014e).
A total of 85 responses were included in the effects assessment; data from 15 studies were used to assess the relationship between quality and relevance of potential effects of IMI to honeybees exposed to systemic residues potentially in plants growing from seeds treated with the chemical. Most of the studies were field studies conducted under good laboratory practice (GLP) guidelines. The quality of these was diminished by insufficient replication, failure to measure any detectable or quantifiable concentrations in field-collected matrices and to adequately characterize exposures below 10 µg/kg, or failure to apply statistical procedures to the data, presumably because there were no adverse effects observed. The QoM of the seed-treated studies was 1.21 ± SE of 0.06 and the values ranged from 0.3 to 1.8 (). The relevance of responses was similar regardless of whether the study was weak or moderately strong because, essentially, all studies indicated that exposure of honeybees to plants that had been sown with seed treated with IMI resulted in no adverse effects on a multitude of measurement endpoints that included mortality (adults, workers, drones, pupae, larvae, queen); colony strength (hive weights, number of workers, total number of adult honeybees, overwintering performance, food consumption rates, etc.); colony development (queen development, brood development, numbers of eggs, larvae, capped cells, pupae, honey, nectar, pollen stores, etc.); colony health (infestation with Varroa mite, viruses, or disease); foraging intensity and activity; flight dynamics (intensity and activity); behavior (trembling, agitation, immobilization, incoordination, hyper- or hypo-responsiveness, etc.); and productivity of the hive (hive weights, honey production, etc.). Combinations of these metrics were generally measured over time (repeated measures) with multiple colony condition assessments (CCA) conducted periodically (four to nine per study) for either one-season or multi-seasonal studies.
Most of the earlier studies included in the WoE were designed to assess exposure and honeybees were used as a tool to collect some of the exposure data. The effects data associated with these earlier studies were generally qualitative observations collected over relatively short periods of time. Since the observational metrics of the honeybees were incidental to the study rather than the direct focus, it is not surprising that these studies indicated that IMI did not present a risk to honeybees. Results of the more recent field and semi-field studies that have focused on effects on honeybees indicate that the exposure concentrations in nectar, pollen, and guttation fluid were either insufficient to cause effects on apical endpoints or minimal exposure of honeybees was occurring. For the most part, residues of IMI in honeybees were not detected. The absence of effects and preponderance of no-effects were attributed, in part, to resilience that has evolved in the social system of honeybees where the colony functions as an “organism” with highly conserved traits. The WoE clearly demonstrates that environmentally realistic concentrations of IMI result in no adverse effects to honeybees at the colony level of biological organization when used appropriately.
The major limitations of the field studies we assessed were the lack of statistical rigor and power in the experimental designs. This was because of insufficient independent replication and the prevalence of pseudo-replication. Statistical procedures are available that can address complex issues that arise when dealing with multiple measures collected over time from multiple sites (independent) or plots. There currently are no standard methods for assessing potential impacts in studies at higher tiers.
Effects of IMI on honeybees resulting from exposures to dust and carryover residues
Field or semi-field studies whereby honeybees were exposed to a combination of seed treatment and dust drift (Bayer CropScience Citation2015c; Schnier et al. Citation2003) or studies with potential soil residues from historical use patterns (Chauzat et al. Citation2009) were evaluated. Fourteen responses were assessed in this QWoE ().
Two studies examined the potential impacts or effects on honeybees exposed to residues from drift of dust during and immediately following sowing of the treated seed. The impact of residues from dust on foraging and flying honeybees in adjacent fields with flowering plants (Bayer CropScience Citation2015c) indicated that there was minimal impact to honeybees (). The second study was weak but showed no mortality (Schnier et al. Citation2003). One study used honeybee colonies to examine the potential effects of “carry-over” residues in soils from historical uses (Chauzat et al. Citation2009). The QoM was 1.73 ± SE of 0.18 and the relevance was zero, which was similar to that of the seed-treatment application.
Ecoepidemiology studies on the effects of IMI on honeybees
Compared to the above sections, fewer higher-tier observational studies were conducted with IMI in honeybees. There were 10 studies with 32 responses (Bacandritsos et al. Citation2010; Bayer Citation2000; Bayer Corporation Citation2001; Chauzat et al. Citation2010; CRA INA Citation2006; CRA ISZVe Citation2005, Citation2006; Garrido-Bailón et al. Citation2010; Nguyen et al. Citation2009, Pohorecka et al. 2012). Only one study (CRA ISZVe Citation2005), which had a QoM of 1.1, identified an adverse response for mortality in hives associated with detections of IMI in dead honeybees (see SI). This response was only observed in 2005 and not in the same region in 2006 (CRA ISZVe Citation2006) (although not all sites were assessed in both years).
As observed in the above sections, some studies were of better quality than the others (); the overall mean for QoM was 1.82 ± SE of 0.20. The mean and SE for relevance were 0.08 ± 0.08. In general, weaknesses were related to lack of full consideration of potential confounders, e.g., exposures to other pesticides, weather, and/or diseases. Weather is unpredictable and might influence the forage crops and/or the honeybees. This is the bane of field studies, but if there are many studies, the weight of evidence will indicate the general trend.
A compilation of several ecoepidemiology studies conducted on the potential effects of IMI on honeybees when used as seed treatment on sunflower in France (INRA Citation1999) did not provide sufficient detail to allow WoE analysis. However, summary conclusions offer corroborative evidence that there were no major differences between treated and control sites in terms of frequency of detection (5% and 1.5%) of IMI in plant tissues, significant differences in production of honey, ability of honeybees to return to the hive, short-term mortality, and activity and development of colonies.
Overall, the weight of evidence does not support a causal relationship between exposure to IMI and adverse effects in honeybees. The better-quality studies did not identify adverse effects and the results of all but one of the weaker studies were consistent. The lack of effects in these studies is likely due primarily to a combination of low exposures and label directions to minimize exposures to honeybees.
Conclusions
The overlay of the mean and 2xSE values from the above five major groupings of studies () provides an overall summary of the weights of evidence of all the studies included in this assessment. These results indicate that IMI, as currently used in good agricultural practices as a seed treatment, does not present a significant risk to honeybees at the level of the colony.
Figure 6. Overview of the conclusions of the WoE for the effects of IMI on honeybees at the level of the colony. Total number of responses = 299. There were no data points obscured by the legend.
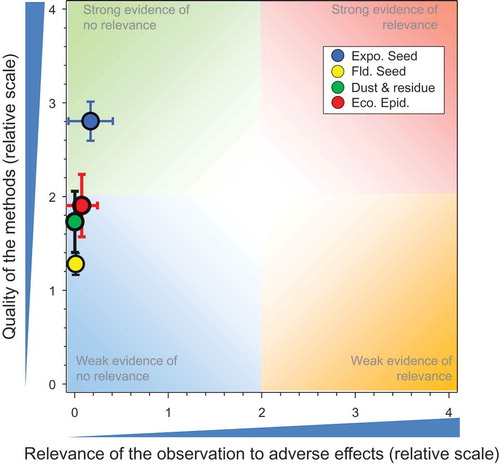
As pointed out above, the effects estimated from exposure of honeybees to IMI via nectar, guttation fluid, and pollen are based on a conservative assumption that honeybees are exposed exclusively to these sources and obtain all food via these routes. The field studies where honeybees are exposed under more realistic conditions, whether from treated seed or other routes of exposure, all show very little relevance for adverse effects on honeybees. The lack of effects in all but one of the ecoepidemiology studies is a further line of evidence of the lack of effects under realistic use conditions where good agricultural practices further mitigate against adverse effects.
Quality of the studies included in this analysis was variable, but the results of the studies are consistent and point to the same conclusion. The overall weight of evidence based on many studies, which included 299 responses, does not falsify the main null hypothesis being tested, i.e., that IMI has no negative impacts on viability and survival of the colony.
Declaration of interest
No potential conflicts of interest were reported by the authors.
Paper-2-IMI-WOE-SUPPLEMENTARY_file.pdf
Download PDF (2.7 MB)Acknowledgments
The authors wish to thank Ms. Jennifer Miller (Miller Environmental Science Inc.) for QA services and Mr. Spencer West for assistance with literature searches and obtaining the references and associated SI. Dr. EJ Marshall and Prof. K Walters are thanked for their detailed and constructive comments on the MS. The authors wish to thank Bayer Crop Science for providing access to the unpublished reports and for funding the study. The decision to publish this assessment was the authors’ and they were solely responsible for the content and the opinions herein.
Supplemental data
Supplemental data for this article can be access on the publisher’s website.
References
- [NWQMC] National Water Quality Monitoring Council. 2016. Water quality data. Accessed January, 2016. NWQMC website http://waterqualitydata.us/portal/.
- [OMECC] Ontario Ministry of Environment and Climate Change. 2017. Accessed January 25, 2017. Stream Neonicotinoid Monitoring Study OMECC website https://www.ontario.ca/search/data-catalogue?sort=asc&query=Neonicotinoid
- Bacandritsos, N., A. Granato, G. Budge, I. Papanastasiou, E. Roinioti, M. Caldon, C. Falcaro, A. Gallina, and F. Mutinelli. 2010. Sudden deaths and colony population decline in Greek honey bee colonies. Journal of Invertebrate Pathology 105:335–40. doi:10.1016/j.jip.2010.08.004.
- Bargańska, Ż., M. Ślebioda, and J. Namieśnik. 2013. Pesticide residues levels in honey from apiaries located of Northern Poland. Food Control 31:196–201. doi:10.1016/j.foodcont.2012.09.049.
- Bayer Corporation. 2001. The impact of GAUCHO® and TI-435 seed-treated canola on honey bees Apis mellifera L., Stilwell, Kansas: Bayer Corporation, (Unpublished Report). Report M-084721-01-1.
- Bayer Corporation. 2002. Imidacloprid (Admire®) residue levels following in-furrow application in potato fields in Prince Edward Island and New Brunswick. Stilwell, Kansas: Bayer Corporation, (Unpublished Report). Report M-061850-01-1.
- Bayer CropScience. 1998. The impact of Gaucho 70 WS seed treated sunflower seeds on honey bees. Leverkusen, Germany: Bayer CropScience (Unpublished Report). Report M-006826-01-2.
- Bayer CropScience. 1999a. Residues of imidacloprid and imidacloprid metabolites in nectar, blossoms, pollen and honey bees sampled from a British summer rape field and effects of these residues on foraging honeybees. Leverkusen, Germany: Bayer CropScience (Unpublished Report). Report M-040023-01-1.
- Bayer CropScience. 1999b. Residues of imidacloprid and imidacloprid metabolites in nectar, blossoms, pollen and honey bees sampled from a summer rape field in Sweden and effects of these residues on foraging honeybees. Leverkusen, Germany: Bayer CropScience (Unpublished Report). Report M-006811-01-1.
- Bayer CropScience. 1999c. Residues of imidacloprid and imidacloprid metabolites in nectar, blossoms, pollen and honey bees sampled from a French summer rape field and effects of these residues on foraging honeybees. Leverkusen, Germany: Bayer CropScience (Unpublished Report). Report M-006815-01-1.
- Bayer CropScience. 1999d. Residue levels of imidacloprid and imidacloprid metabolites in nectar, blossoms and pollen of sunflowers cultivated on soils with different imidacloprid residue levels and effects of these residues on foraging honeybees: Test Location: farmland “Hofchen” - 1999. Leverkusen, Germany: Bayer CropScience (Unpublished Report). Report M-016820-01-1.
- Bayer CropScience. 1999e. Residue levels of imidacloprid and imidacloprid metabolites in nectar, blossoms and pollen of sunflowers cultivated on soils with different imidacloprid residue levels and effects of these residues on foraging honeybees: Test Location: farmland “Laacher Hof’ - 1999. Leverkusen, Germany: Bayer CropScience (Unpublished Report). Report M-016827-01-1.
- Bayer CropScience. 2002. Field test: Side effects of oil-seed rape grown from seeds dressed with imidacloprid and beta-cyfluthrin FS 500 on the Honey Bee (Apis mellifera L.). Leverkusen, Germany: Bayer CropScience (Unpublished Report). Report M-066846-01-1.
- Bayer CropScience. 2007. Residue levels of imidacloprid and imidacloprid metabolites in nectar, blossoms and pollen of summer rape cultivated on soils with different imidacloprid residue levels and effects of these residues on foraging honeybees: Test Location: Farmland “Laacher Hof’ - 1999. Report Amendment No. 1 13 April 2007. Leverkusen, Germany: Bayer CropScience (Unpublished Report). Report M-016828-02-1.
- Bayer CropScience. 2009. Monitoring of dust drift deposits during and after the drilling of winter barley, dressed with MANTA PLUS® In Germany. Monheim, Germany: Bayer CropScience (Unpublished Report). Report M-342603-01-1.
- Bayer CropScience. 2011a. Determination of residue levels of imidacloprid, imidacloprid-monohydroxy and imidacloprid-olefine in bee-relevant matrices of winter rape in a cereal succeeding crop scenario at bayer cropscience ag experimental farm “Laacher Hof”, Germany. Monheim, Germany: Bayer CropScience (Unpublished Report). Report M-406075-01-1.
- Bayer CropScience. 2011b. Determination of residue levels of imidacloprid, imidacloprid-monohydroxy and imidacloprid-olefine in bee-relevant matrices of winter rape in a cereal succeeding crop scenario at bayer cropscience ag experimental farm “Höfchen”, Germany. Monheim, Germany: Bayer CropScience (Unpublished Report). Report M-406083-01-1.
- Bayer CropScience. 2012a. Imidacloprid - determination of residues of imidacloprid in pollen, extrafloral nectar fluids and nectar of cotton plants grown from imidacloprid-treated seeds in two cotton growing areas in Greece 2011. Monheim, Germany: Bayer CropScience (Unpublished Report). Report M-424399-01-1.
- Bayer CropScience. 2012b. Final Report (Non-GLP) field study to monitor potential effects on honey bees from exposure to guttation fluid of winter barley (W-BAR), seed-treated either with an imidacloprid or a clothianidin combi-product. Monheim, Germany: Bayer CropScience (Unpublished Report). Report M-498922-01-1.
- Bayer CropScience. 2012c. Assessment of exposure of honey bees (Apis mellifera) to imidacloprid in controlled feeding study, interim Report. Research Triangle Park, NC: Bayer CropScience (Unpublished Report). Report M-442872-01-1.
- Bayer CropScience. 2013. Pilot study: Honey bee brood and colony level effects following imidacloprid intake via treated artificial diet in a field study in North Carolina. Research Triangle Park, NC: Bayer CropScience (Unpublished Report). Report M-442868-02-1.
- Bayer CropScience. 2014a. Determination of the residues of imidacloprid and its metabolites 5-hydroxy imidacloprid and imidacloprid olefin in bee relevant matrices collected from treated cotton during two successive years and in white clover planted after treated cotton. Research Triangle Park, NC: Bayer CropScience (Unpublished Report). Report M-501306-01-1.
- Bayer CropScience. 2014b. A long-term field study to monitor potential effects on the honeybee (Apis mellifera L.) from exposure to guttation fluid of sugar beets, seed-treated with the insecticides clothianidin + imidacloprid + beta-cyfluthrin in Southern Germany in 2013 and 2014. Monheim, Germany: Bayer CropScience (Unpublished Report). Report M-500734-01-1.
- Bayer CropScience. 2014c. A long-term field study to monitor potential effects on the honeybee (Apis mellifera L.) from exposure to guttation fluid of sugar beets, seed-treated with the insecticides clothianidin + imidacloprid + beta-cyfluthrin in Southern Germany in 2013 and 2014. Monheim, Germany: Bayer CropScience (Unpublished Report). Report M-500724-01-1.
- Bayer CropScience. 2014d. Field study to monitor potential effects on honey bees from exposure to guttation fluid of winter Barley (W-BAR), seed-treated with the insecticidal seed-treatment product clothianidin + imidacloprid FS 100 + 175 G in Germany in 2011/2012. Monheim, Germany: Bayer CropScience (Unpublished Report). Report M-501261-01-1.
- Bayer CropScience. 2014e. Final report assessment of potential impacts on honeybee colony development, their hibernation performance and concurrent monitoring of aerial dust drift during the sowing operation of poncho beta plus - treated sugar beet pills with typical commercial vacuum-pneumatic sowing technology, directly adjacent to full-flowering Phacelia tanacetifolia in Germany. Monheim, Germany: Bayer CropScience (Unpublished Report). Report M-504065-01-1.
- Bayer CropScience. 2014f. Final report (Non-GLP) field study to monitor potential effects on honey bees from exposure to guttation fluid of winter wheat (W-WHT), seed-treated either with an imidacloprid or a clothianidin combi-product. Monheim, Germany: Bayer CropScience (Unpublished Report). Report M-498939-01-1.
- Bayer CropScience. 2014g. Honey bee colony feeding study, evaluating the effects of imidacloprid-fortified artificial nectar diet on long term colony health in a field study in North Carolina: Colony condition assessment data & statistic. Research Triangle Park, NC: Bayer CropScience (Unpublished Report). Report M-478404-02-1
- Bayer CropScience. 2014h. Honey bee brood and colony level effects following imidacloprid intake via treated artificial diet in a field study in North Carolina. Research Triangle Park, NC: Bayer CropScience (Unpublished Report). Report M-501299-01-1.
- Bayer CropScience. 2014i. Determination of the residues of imidacloprid and its metabolites imidacloprid-5-hydroxy and imidacloprid-olefinin bee relevant matrices collected in a succeeding crop scenario with natural aged residues of imidacloprid. Field phase conducted with winter oil seed rape in Northern France. Monheim, Germany: Bayer CropScience (Unpublished Report). Report M-504810-01-1.
- Bayer CropScience. 2014j. Determination of the residues of imidacloprid and its metabolites imidacloprid-5-hydroxy and imidacloprid-olefin in bee relevant matrices collected in a succeeding crop scenario with natural aged residues of imidacloprid. Field phase conducted with Phacelia and Maize in Northern France. Monheim, Germany: Bayer CropScience (Unpublished Report). Report M-504836-01-1.
- Bayer CropScience. 2014k. Determination of the residues of imidacloprid in bee relevant matrices collected from succeeding crops following application of imidacloprid FS 600E G via soil incorporation to plateau concentration and sowing of imidacloprid-treated winter barley seeds. Field phase conducted in Southern France. Monheim, Germany: Bayer CropScience (Unpublished Report). Report M-504842-01-1.
- Bayer CropScience. 2015a. Determination of residues of imidacloprid and clothianidin in flowers, leaves, soil, nectar and pollen of cotton after seed treatment with Gaucho® FS (Imidacloprid 600 FS) or Poncho® (Clothianidin 600 FS), or Foliar Application with Provado® 200 SC (Imidacloprid 200 SC) in a Semi-Field Study in Brazil. Monheim, Germany: Bayer CropScience (Unpublished Report). Report M-525745-01-1.
- Bayer CropScience. 2015c. Assessment of potential impacts on honeybee colony development, their hibernation performance and concurrent monitoring of aerial dust drift during the sowing operation of imidacloprid FS 350A G - treated winter barley with typical commercial pneumatic sowing technology, directly adjacent to full-flowering Phacelia tanacetifolia in United Kingdom. Monheim, Germany: Bayer CropScience (Unpublished Report). Report M-504522-02-1.
- Bayer CropScience AG. 2010. Determination of Residues of Clothianidin and Imidacloprid and their Metabolites in Melon Following an Application of Clothianidin & Imidacloprid WS 56.25 + 18.75 as seed treatment. Niefern-Öschelbronn, Germany: Bayer CropScience AG (Unpublished Report). Report M-361798-01-1.
- Bayer CropScience AG. 2014. Field study to monitor potential effects on honey bees from exposure to guttation fluid of winter wheat (W-WHT), seed-treated either with an imidacloprid or a clothianidin combi-product. Monheim, Germany: Bayer CropScience AG (Unpublished Report). Report M-498939-01-1.
- Bayer CropScience AG. 2015a. Determination of residues of imidacloprid and clothianidin in flowers, leaves, soil, nectar and pollen of cotton after seed treatment with Gaucho® FS (Imidacloprid 600 FS) or Poncho® (Clothianidin 600 FS), or foliar application with Provado® 200 SC (Imidacloprid 200 SC) in a semi-field study in Brazil. Niefern-Oeschelbronn, Germany: Bayer CropScience AG (Unpublished Report). Report M-512861-01-1.
- Bayer CropScience. 2015b. Determination of residues of imidacloprid and clothianidin in flowers, leaves, soil, nectar and pollen of soybean after seed treatment with Gaucho® FS (Imidacloprid 600 FS) or Poncho® (Clothianidin 600 FS), or foliar application with Connect® (Imidacloprid & Beta-Cyfluthrin 112.5 SC) in a semi-field study in Brazil. Niefern-Oeschelbronn, Germany: Bayer CropScience AG (Unpublished Report). Report M-515291-01-1.
- Bayer CropScience AG. 2015c. Determination of residues of imidacloprid and clothianidin in flowers, leaves, soil, nectar and pollen of cotton after seed treatment with Gaucho® FS (Imidacloprid 600 FS) or Poncho® (Clothianidin 600 FS), or foliar application with Provado® 200 SC (Imidacloprid 200 SC) in a semi-field study in Brazil. Niefern-Oeschelbronn, Germany: Bayer CropScience AG (Unpublished Report). Report M-519517-01-1.
- Bayer CropScience. 2015d. Determination of residues of imidacloprid and clothianidin in flowers, leaves, soil, nectar and pollen of cotton after seed treatment with Gaucho® FS (Imidacloprid 600 FS) or Poncho® (Clothianidin 600 FS), or foliar application with Provado® 200 SC (Imidacloprid 200 SC) in a semi-field study in Brazil. Monheim, Germany: Bayer CropScience (Unpublished Report). Report M-525732-01-1.
- Bayer, S. A. 2000. Field evaluation in Argentina of possible risk for honey bees from the product ‘Gaucho’ on Sunflowers. Buenos Aires, Argentina: Bayer SA, (Unpublished Report). Report M-090720-02-1.
- BCPC. 2015. The Pesticide Manual (12th ed.), ed. Tomlin, C. D. S. Alton, UK: British Crop Protection Council. In editor C. D. S. Tomlin. Alton, Hampshire, UK: British Crop Protection Council.
- Bernal, J., E. Garrido-Bailón, M. J. Del Nozal, A. V. González-Porto, R. Martín-Hernández, J. C. Diego, J. J. Jiménez, J. L. Bernal, and M. Higes. 2010. Overview of pesticide residues in stored pollen and their potential effect on bee colony (Apis mellifera) losses in Spain. Journal of Economic Entomology 103:1964–71. doi:10.1603/EC10235.
- Botías, C., A. David, J. Horwood, A. Abdul-Sada, E. Nicholls, E. Hill, and D. Goulson. 2015. Neonicotinoid residues in wildflowers, a potential route of chronic exposure for bees. Environmental Science & Technology 49:12731–40. doi:10.1021/acs.est.5b03459.
- Chauzat, M.-P., P. Carpentier, A.-C. Martel, S. Bougeard, N. Cougoule, P. Porta, J. Lachaize, F. Madec, M. Aubert, and J.-P. Faucon. 2009. Influence of pesticide residues on honey bee (Hymenoptera: Apidae) colony health in France. Environmental Entomology 38:514–23. doi:10.1603/022.038.0302.
- Chauzat, M.-P., J.-P. Faucon, A.-C. Martel, J. Lachaize, N. Cougoule, and M. Aubert. 2006. A survey of pesticide residues in pollen loads collected by honey bees in France. Journal of Economic Entomology 99:253–62. doi:10.1093/jee/99.2.253.
- Chauzat, M.-P., A.-C. Martel, P. Blanchard, M.-C. Clément, F. Schurr, C. Lair, M. Ribière, K. Wallner, P. Rosenkranz, and J.-P. Faucon. 2010. A case report of a honey bee colony poisoning incident in France. Journal of Apicultural Research 49:113–15. doi:10.3896/IBRA.1.49.1.22.
- Chauzat, M.-P., A.-C. Martel, N. Cougoule, P. Porta, J. Lachaize, S. Zeggane, M. Aubert, P. Carpentier, and J.-P. Faucon. 2011. An assessment of honeybee colony matrices, Apis mellifera (Hymenoptera: Apidae) to monitor pesticide presence in continental France. Environmental Toxicology and Chemistry 30:103–11. doi:10.1002/etc.361.
- CRA INA. 2006. depopulation and mortality events of bees in beehives with different agricultural destinations in the region Emilia Romagna Final Report 2006. Milan, Italy: CRA - Istituto Nazionale di Apicoltura. Report M-428630-01-2.
- CRA ISZVe. 2005. Monitoring of depopulation and mortality events of bees in beehives with different agricultural destinations in the region Veneto report 2005. Legnaro, Italy: Centro Regionale per l’Apicoltura, Istituto Zooprofilattico Sperimentale delle Venezie. Report M-428632-01-2.
- CRA ISZVe. 2006. Monitoring of depopulation and mortality events of bees in beehives with different agricultural destinations in the region Veneto report 2006. Legnaro, Italy: Centro Regionale per l’Apicoltura, Istituto Zooprofilattico Sperimentale delle Venezie. Report M-428631-01-2.
- Dively, G. P., M. S. Embrey, A. Kamel, D. J. Hawthorne, and J. S. Pettis. 2015. Assessment of chronic sublethal effects of imidacloprid on honey bee colony health. PLoS ONE 10:e0118748. doi:10.1371/journal.pone.0118748.
- EFSA. 2008. Conclusion regarding the peer review of the pesticide risk assessment of the active substance imidacloprid. EFSA Journal 2008:1–120.
- EFSA. 2013. Conclusion on the peer review of the pesticide risk assessment for bees for the active substance imidacloprid. EFSA Journal 11–3068:55.
- Ensminger, M., R. Budd, K. Kelley, and K. Goh. 2013. Pesticide occurrence and aquatic benchmark exceedances in urban surface waters and sediments in three urban areas of California, USA, 2008–2011. Environmental Monitoring and Assessment 185:3697–710. doi:10.1007/s10661-012-2821-8.
- Fairbrother, A., J. Purdy, T. Anderson, and R. Fell. 2014. Risks of neonicotinoid insecticides to honeybees. Environmental Toxicology and Chemistry 33:719–31. doi:10.1002/etc.v33.4.
- Garrido-Bailón, E., R. Martín-Hernández, J. Bernal, J. Bernal, A. Martínez-Salvador, L. Barrios, A. Meana, and M. Higes. 2010. Short communication. The detection of Israeli Acute Paralysis virus (IAPV), fipronil and imidacloprid in professional apiaries are not related with massive honey bee colony loss in Spain. Spanish Journal of Agricultural Research 8:658–61. doi:10.5424/sjar/2010083-1262.
- Girolami, V., M. Marzaro, L. Vivan, L. Mazzon, C. Giorio, D. Marton, and A. Tapparo. 2013. Aerial powdering of bees inside mobile cages and the extent of neonicotinoid cloud surrounding corn drillers. Journal of Applied Entomology 137:35–44. doi:10.1111/jen.2012.137.issue-1-2.
- Girolami, V., M. Marzaro, L. Vivan, L. Mazzon, M. Greatti, C. Giorio, D. Marton, and A. Tapparo. 2012. Fatal powdering of bees in flight with particulates of neonicotinoids seed coating and humidity implication. Journal of Applied Entomology 136:17–26. doi:10.1111/jen.2011.136.issue-1-2.
- Goulson, D. 2013. Review: An overview of the environmental risks posed by neonicotinoid insecticides. The Journal of Applied Ecology 50:977–87. doi:10.1111/1365-2664.12111.
- Hladik, M. L., and D. W. Kolpin. 2016. First national-scale reconnaissance of neonicotinoid insecticides in streams across the USA. Environmental Chemistry 13:12–20. doi:10.1071/EN15061.
- INRA. 1999. Effects of crop protection products on bees effects of gaucho seed dressing on losses of foraging bees. Bures-sur-Yvette, France: Laboratoire de Neurobiologie Comparee des Invertebre, INRA. Report M-110240-02-1.
- Jeschke, P., R. Nauen, M. Schindler, and A. Elbert. 2011. Overview of the status and global strategy for neonicotinoids. Journal of Agricultural and Food Chemistry 59:2897–908. doi:10.1021/jf101303g.
- Kasiotis, K. M., C. Anagnostopoulos, P. Anastasiadou, and K. Machera. 2014. Pesticide residues in honeybees, honey and bee pollen by LC-MS/MS screening: Reported death incidents in honeybees. Science of the Total Environment 485–486:633–42. doi:10.1016/j.scitotenv.2014.03.042.
- Laurent, F. M., and E. Rathahao. 2003. Distribution of [14C] imidacloprid in sunflowers (Helianthus annuus L.) following seed treatment. Journal of Agricultural and Food Chemistry 51:8005–10. doi:10.1021/jf034310n.
- Main, A. R., J. Fehr, K. Liber, J. V. Headley, K. M. Peru, and C. A. Morrissey. 2017. Reduction of neonicotinoid insecticide residues in Prairie wetlands by common wetland plants. Science of the Total Environment 579:1193–202. doi:10.1016/j.scitotenv.2016.11.102.
- Main, A. R., J. V. Headley, K. M. Peru, N. L. Michel, A. J. Cessna, and C. A. Morrissey. 2014. Widespread use and frequent detection of neonicotinoid insecticides in wetlands of Canada’s Prairie Pothole Region. PLoS ONE 9:e92821. doi:10.1371/journal.pone.0092821.
- Main, A. R., N. L. Michel, M. C. Cavallaro, J. V. Headley, K. M. Peru, and C. A. Morrissey. 2016. Snowmelt transport of neonicotinoid insecticides to Canadian Prairie wetlands. Agriculture, Ecosystems & Environment 215:76–84. doi:10.1016/j.agee.2015.09.011.
- Mullin, C. A., M. Frazier, J. L. Frazier, S. Ashcraft, R. Simonds, D. Vanengelsdorp, and J. S. Pettis. 2010. High levels of miticides and agrochemicals in North American apiaries: Implications for honey bee health. PLoS ONE 5:e9754. doi:10.1371/journal.pone.0009754.
- Nguyen, B. K., C. Saegerman, C. Pirard, J. Mignon, J. Widart, B. Thirionet, F. Verheggen, D. Berkvens, E. De Pauw, and E. Haubruge. 2009. Does imidacloprid seed-treated maize have an impact on honey bee mortality? Journal of Economic Entomology 102:616–23. doi:10.1603/029.102.0220.
- Phillips, P. J., and R. W. Bode. 2004. Pesticides in surface water runoff in south-eastern New York State, USA: Seasonal and stormflow effects on concentrations. Pest Management Science 60:531–43. doi:10.1002/(ISSN)1526-4998.
- Pohorecka, K., P. Skubida, A. Miszczak, P. Semkiw, P. Sikorski, K. Zagibajło, D. Teper, Z. Kołtowski, M. Skubida, D. Zdańska, and A. Bober. 2012. Residues of neonicotinoid insecticides in bee collected plant materials from oilseed rape crops and their effect on bee colonies. Journal Apicultural Sciences 56:115–34. doi:10.2478/v10289-012-0029-3.
- Reetz, J. E., S. Zühlke, M. Spiteller, and K. Wallner. 2011. Neonicotinoid insecticides translocated in guttated droplets of seed-treated maize and wheat: A threat to honeybees? Apidologie 42:596–606. doi:10.1007/s13592-011-0049-1.
- Schaafsma, A., V. Limay-Rios, T. Baute, J. Smith, and Y. Xue. 2015. Neonicotinoid insecticide residues in surface water and soil associated with commercial maize (corn) fields in Southwestern Ontario. PLoS ONE 10:e0118139. doi:10.1371/journal.pone.0118139.
- Schnier, H. F., G. Wenig, F. Laubert, V. Simon, and R. Schmuck. 2003. Honey bee safety of imidacloprid corn seed treatment. Bulletin of Insectology 56:73–76.
- Solomon, K. R., and G. L. Stephenson. 2017. Quantitative weight of evidence assessment of higher tier studies on the toxicity and risks of neonicotinoid insecticides in honeybees 1: Methods. Journal of Toxicology and Environmental Health B.
- Starner, K., and K. S. Goh. 2012. Detections of the neonicotinoid insecticide imidacloprid in surface waters of three agricultural regions of California, USA, 2010–2011. Bulletin of Environmental Contamination and Toxicology 88:316–21. doi:10.1007/s00128-011-0515-5.
- Stewart, S. D., G. M. Lorenz, A. L. Catchot, J. Gore, D. Cook, J. Skinner, T. C. Mueller, D. R. Johnson, J. Zawislak, and J. Barber. 2014. Potential exposure of pollinators to neonicotinoid insecticides from the use of insecticide seed treatments in the Mid-Southern United States. Environment Science & Technology 48:9762–69. doi:10.1021/es501657w.
- Struger, J., J. Grabuski, S. Cagampan, E. Sverko, D. McGoldrick, and C. H. Marvin. 2017. Factors influencing the occurrence and distribution of neonicotinoid insecticides in surface waters of southern Ontario, Canada. Chemosphere 169:516–23. doi:10.1016/j.chemosphere.2016.11.036.
- USEPA. 2014. Guidance for Assessing Pesticide Risks to Bees. Washington, DC: Office of Chemical Safety and Pollution Prevention, Office of Pesticide Programs. United States Environmental Protection Agency. Report
- Vijver, M. G., and P. J. Van Den Brink. 2014. Macro-invertebrate decline in surface water polluted with imidacloprid: A rebuttal and some new analyses. PLoS ONE 9:e89837. doi:10.1371/journal.pone.0089837.
- Wu-Smart, J., and M. Spivak. 2016. Sub-lethal effects of dietary neonicotinoid insecticide exposure on honey bee queen fecundity and colony development. Scientific Reports 6:32108. doi:10.1038/srep32108.