Abstract
Particulate matter (PM) has long been recognized as an air pollutant due to its adverse health and environmental impacts. As emission of PM from agricultural operations is an emerging air quality issue, the Agricultural Particulate Matter Emissions Indicator (APMEI) has been developed to estimate the primary PM contribution to the atmosphere from agricultural operations on Census years and to assess the impact of practices adopted to mitigate these emissions at the soil landscape polygon scale as part of the agri-environmental indicator report series produced by Agriculture and Agri-Food Canada. In the APMEI, PM emissions from animal feeding operations, wind erosion, land preparation, crop harvest, fertilizer and chemical application, grain handling, and pollen were calculated and compared for the Census years of 1981–2006. In this study, we present the results for PM10 and PM2.5, which exclude chemical application and pollen sources as they only contribute to total suspended particles. In 2006, PM emissions from agricultural operations were estimated to be 652.6 kt for PM10 and 158.1 kt for PM2.5. PM emissions from wind erosion and land preparation account for most of PM emissions from agricultural operations in Canada, contributing 82% of PM10 and 76% of PM2.5 in 2006. Results from the APMEI show a strong reduction in PM emissions from agricultural operations between 1981 and 2006, with a decrease of 40% (442.8 kt) for PM10 and 47% (137.7 kt) for PM2.5. This emission reduction is mainly attributed to the adoption of conservation tillage and no-till practices and the reduction in the area of summerfallow land.
Increasing sustainability in agriculture often means adapting management practices to have a beneficial impact on the environment while maintaining or increasing production and economic benefits. We developed an inventory of primary PM emissions from agriculture in Canada to better quantify the apportionment, spatial distribution, and trends for Census years 1981–2006. We found major reductions of 40% in PM10 and 47% in PM2.5 emissions over the 25-yr period as a co-benefit of increasing carbon sequestration in agricultural soils. Indeed, farmers adopted conservation tillage/no-till practices, increased usage of cover crops, and reduced summerfallow, in order to increase soil organic matter and reduce carbon dioxide emissions, which also reduced primary PM emissions, although the agricultural production increased over the period.
Supplemental materials are available for this article. Go to the publisher's online edition of the Journal of the Air & Waste Management Association for primary PM emissions at the soil landscape polygon scale from Canadian agricultural sources in 2006 (adapted from CitationPattey et al., 2010) and for previous Census years between 1981 and 2001.
Introduction
Particulate matter (PM) has been recognized as an air pollutant due to its adverse impacts on environmental and mammalian health. Increase in PM, especially PM2.5 concentration, is associated with adverse health effects, such as increased respiratory diseases and premature death, as reported by numerous epidemiological studies (e.g., Donham and Thelin, 2006; CitationSamet and Krewski, 2007; CitationU.S. EPA, 2004). Ambient PM contributes to visibility impairment, smog, acid rain, and stratospheric ozone depletion and also influences climate by changing surface energy balance (CitationRamanathan and Feng, 2009). Using AURAMS, an atmospheric chemistry model, to simulate continental concentrations of air pollutants, CitationBouchet et al. (2009) predicted an increase in annual primary PM2.5 emissions in Canada between 2002 and 2015, although a reduction in ambient ozone concentration was projected following air-quality mitigation measures adopted in the United States and Canada. The emission of PM from agricultural operations is an emerging air quality issue, especially at the boundaries between rural and urban environments. Agricultural operations have been recognized as a significant source of PM emitted into the atmosphere (CitationSaxton, 1996). The main agricultural sources of primary PM include dust from soil and biogenic material, droplets and particles from agrochemicals, bacteria, endotoxins, and spores. Organic exposures may affect the airways and, depending on the antigenicity of the material and host susceptibility, may result in asthma, asthma-like symptoms, or chronic obstructive pulmonary disease (CitationAmerican Thoracic Society, 1998). Inhalation of fungal spores leads primarily to parenchymal dysfunction such as hypersensitivity pneumonitis. Endotoxins found in moldy hay, grain, and similar organic materials may create a complex of constitutional symptoms known as the organic dust toxic syndrome. Agricultural worker exposure to PM has been associated with mild to chronic respiratory allergic reactions, commonly known as farmer's lung (CitationCanadian Centre for Occupational Health and Safety, 1999).
Canada is the fifth world exporter of agriculture and agri-food products, and the agriculture sector accounts for 8% of Canada's gross domestic production. In 2008, 7.4% of total Canadian landmass area was in farmland (i.e., 0.68 million km2). The climatic zones of the agroecosystem range from subhumid in the Prairies (Alberta, Saskatchewan, Manitoba) to temperate in Ontario and Quebec and maritime in New Brunswick, Nova Scotia and Prince Edward Island. British Columbia belongs to a separate ecozone—the Pacific Maritime, which is Canada's warmest and wettest climate.
The different eco-regions of Canada are represented by different agriculture and agricultural practices. Crop production within the Prairie Provinces consists largely of grain and oilseed cultivation, mainly wheat and canola. Animal production in this region consists primarily of beef feedlot operations. In contrast, the majority of agriculture in Eastern Canada is comprised of smaller scale dairy farms with hay, corn, soybean, and cereal crop rotation systems intended to provide feed for animal production. Swine and poultry production is largely concentrated in Manitoba, Ontario, and Quebec.
Despite these regional differences, agricultural practices in Canada have exhibited major transitional shifts over a 25-year period (1981–2006): from small family-operated farms to larger, more industrialized operations, in response to cost efficiency, food demand, and technology development (CitationEilers et al., 2010). A set of agri-environmental indicators was developed in Canada to report and assess the trends in environmental sustainability of agricultural activities on Census years. Until 2005, only the greenhouse gas budget was reported under the air quality sub-category. Ammonia and particulate matter were made available in report number 3 (CitationEilers et al., 2010) as they contribute to the reduction of air quality. In 2006, PM emissions from Canadian agriculture represented approximately 18% of total PM10 open sources (CitationEnvironment Canada, 2008). As many potentially significant sources were excluded in the existing PM inventories (CitationEnvironment Canada, 2008), the Agricultural Particulate Matter Emissions Indicator (APMEI ; CitationPattey et al., 2010) was created to assess in greater detail primary PM emissions (PM2.5, PM10, and TSP) from Canadian agriculture at the soil landscape polygon scale. In this study, we introduce the APMEI and present the results of PM10 and PM2.5 emissions for the Census years of 1981–2006.
Methodology
The APMEI was designed to quantify annual primary PM emissions from agricultural operations within Canada on the Census years. In total, eight agricultural operations (categories) were included in the indicator between 1981 and 2006 and two additional categories (crop residue burning and animal cremation) were computed in 2001 and 2006. The categories included in the emissions inventory are wind erosion, land preparation, crop harvest, grain handling, pollen emission, fertilizer application, chemical application, animal feeding operations, crop residue burning, and animal cremation. Chemical application and pollen sources only contribute to coarse particles (>10 μm) and are not presented here, as we report the results of PM10 and PM2.5 emissions. Emissions estimates were conducted for nine of 10 provinces in Canada. The three northern territories and Newfoundland were not included in this emissions inventory due to a limited data set and the extremely small land areas that are represented by agriculture. The emissions estimates were calculated in a similar manner to other emissions inventories, where the PM emissions are based upon an activity level and an emission factor:
where ERi,j is the emission rate of soil landscape polygon j for activity i, APi,j is the intensity of activity i at polygon j, and EFi is the emission factor for activity i.
To adequately assess the spatial representation of the annual PM emissions throughout Canada, this inventory uses the Soil Landscape of Canada (SLC v3.0), a geographic information system (GIS) framework developed by Agriculture and Agri-Food Canada. The activity level is based primarily on the Interpolated Census of Agriculture (ICOA), where the Census of Agriculture data provided by Statistics Canada are interpolated into the SLC polygon level. The use of the SLC polygons is beneficial for calculating PM emissions, given the strong linkage between soil type and PM emissions for a majority of field activities such as land preparation and wind erosion. Information on management practices were derived from Farm Environmental Management Survey (FEMS; CitationStatistics Canada, 2006). The 30-yr climatic normals were used on Ecodistrict basis. Other activity data were found in the quarterly reports on grain yield from the Canada grains council, the annual reports on fertilizer consumption, shipment and trade of the Canadian Fertilizer Institute, and in Grain trade of Canada of Statistics Canada. Emission factors for each agricultural sector were derived from previous empirical studies (). The primary resources for emission factors were the U.S. Environmental Protection Agency (EPA) AP-42, the California Air Resources Board, and peer-reviewed scientific papers. Given the vast area of agricultural land within Canada, which spans numerous different landscapes and climatic zones, the use of an emission inventory provides the most feasible method of determining primary PM emissions from agricultural sources in Canada.
Table 1. Summary of emission factor sources per activity
Animal Feeding Operations
Primary PM emissions from livestock production consist of feather fragments, fecal material, skin debris or dander, feed particles, animal wastes, mold spores, bacteria, fungus, litter fragments, and others. Factors that affect the PM release from livestock production are animal activity, feeding patterns, temperature, relative humidity, ventilation rate, building design, litter, and manure collection system (CitationLim et al., 2003; CitationTakai et al., 1998). Measurement results from Europe showed that PM concentrations in animal confinement buildings are greatest in poultry houses, followed by swine buildings and then cattle buildings (CitationTakai et al., 1998). CitationSeedorf et al. (1998) attributed the greater PM concentrations in both swine and poultry facilities to a lower relative humidity compared to cattle barns. In addition, poultry and swine are typically indoor feeding operations in Canada, while cattle are hosted outdoors, especially for the warm seasons, or year round in feedlots. Ventilation systems are required for livestock buildings to facilitate adequate air exchange. Thus, a portion of the PM generated from confined livestock buildings will be emitted into the atmosphere via the ventilation system. This proportion will vary according to the ventilation system type (i.e., mechanical or natural), the air renewal rate, and filtering capacity. For animal feeding operations, the EF in kg head−1 yr−1 for each animal type () was calculated as:
Table 2. PM emission factors () for livestock production expressed on animal unit basis (AU)
where BMi is the average body mass (kg) for animal type i, EFAU(i) is the emission factor (g AU−1 d−1 with AU: animal unit; ), and CFi is the annual confinement or production time (d) for animal type i (CitationBoadi et al., 2004; CitationLeeson, 2005).
PM emissions are calculated by multiplying the emission rate per animal by animal population based on the emission factor approach. To account for the impacts of animal types on the PM emissions, livestock is divided into four animal categories and a total of 18 subcategories according to the data available from Statistics Canada and the level of detail needed for the calculations. Additionally, the animals are compared on an AU basis of 500 kg in order to accurately compare emissions between the different animal categories ().
Wind Erosion
Wind erosion commonly occurs as wind blows across exposed agricultural land and results in PM emissions from the entrained particles. Wind erosion is a concern in western regions of Canada, especially in Saskatchewan and Alberta. The introduction of wind breaks and beneficial management practices to protect the field surface has reduced wind erosion. Unlike persistently vacant land, windblown dust emissions from agricultural land varies with the change in canopy and soil cover due to land management, planting, crop canopy development, harvesting, and snow cover. In order to improve wind erosion estimates from agricultural land, several adjustment factors were included to provide temporal canopy and soil cover over an annual cycle. These factors included: (1) crop canopy cover, (2) postharvest vegetative cover (i.e., residue), and (3) snow cover during the winter.
Windblown dust emissions from agricultural lands are calculated by multiplying the cultivated cropland area by a PM emission factor calculated using the Wind Erosion Equation (WEQ; CitationWoodruff and Siddoway, 1965), for which the impact of soil cover change on PM emissions is considered (CitationCARB, 1997). The windblown PM emission factor from agricultural lands is calculated as (CitationCARB, 1997; CitationU.S. EPA, 1985):
where A is the portion of total wind erosion losses (A = 0.0125 for PM10, and 0.0025 for PM2.5), Ij is the soil erodibility (kg ha−1 yr−1) at SLC polygon j, Ki is the dimensionless surface roughness factor for crop type i, Cj is a dimensionless climatic factor at SLC polygon j, L'i,j is a dimensionless unsheltered field width factor, and SLRi,j is the soil loss rate defined as the ratio of the soil loss for a soil under a given canopy cover (or soil cover) divided by the soil loss from bare soil.
Land Preparation
Agricultural tillage is the common method for Canadian farmers to prepare the land for seeding and weed control. PM emissions are generated from airborne soil particles during tillage due to the mechanical disturbance of the soil surface.
PM emissions from agricultural tilling are proportional to the area tilled and the number of tillage events per year (CitationU.S. EPA, 1985), and are also dependent on soil texture and machinery type (e.g., plow, disk, and cultivator). However, it is difficult to collect information on equipment type used for each tillage event at the SLC level. In this study, PM emissions from agricultural tilling for each SLC polygon were estimated as:
where Ei,j is the emission rate for PM10 or PM2.5 from agricultural tilling for crop type i at SLC j (kg yr−1), EFj is the emission factor for PM10 or PM2.5 at SLC polygon j (kg ha−1), CAi is the agricultural tilling area for crop type i (ha), NPi,k is the number of tilling events per year for crop type i and tillage practice type k (conventional tillage, conservation tillage or no tillage), and TPk is the percentage of tillage practice type k.
The number of tillage events per year depends on tillage practices. For example, conservation tillage has fewer tilling events per year compared to conventional tillage.
Based on data available from Statistics Canada, the emission factor from agricultural tilling was calculated as (CitationU.S. EPA, 1985):
where k is the particle size multiplier, k = 0.21 for PM10 and 0.042 for PM2.5, sij is the silt content of surface soil (%) for component i at SLC polygon j defined as the mass fraction of particles <75 μm diameter found in the soil to a depth of 10 cm, and pij is the percentage of component i in polygon j. Silt content of surface soil was determined according to the soil texture class at SLC polygons based on - (CitationU.S. EPA, 1998). Information on the number of tilling events per year for crop type and tillage practice type was taken from a soil cover indicator (CitationHuffman and Coote, 2010).
Table 3. Summary of assumed crop emission factors from harvest
Table 4. Emission factors selected for crop residue burning in the indicator
Harvest
PM generated from agricultural harvesting, commonly referred to as grain dust, includes grain and dry plant particles, molds, pollen and spores, silica, bacteria, fungi, insects, and possibly pesticide residues. These emissions are due to mechanical disturbances in the field caused by combines processing plant material and vehicles traveling over the field.
PM emissions from agricultural harvest operations are computed by multiplying an emission factor and an activity factor using the following equation:
where Ei,j is the emission rate of either PM10 or PM2.5 (kg yr−1) for SLC polygon j and crop type i (kg yr−1), EFi is the emission factor (kg ha−1) of crop type i, and AHi,j is the harvested crop area for crop type i at a SLC polygon j (ha yr−1).
The emission factors of PM10 proposed by California Air Research Board (2003) were adopted and used to calculate PM emissions from agricultural harvest (). Where the specific emission factors for some crops were not available from CARB, the emission factors for these crops were based on an approximation from the closest representation (CitationCARB, 2003). The emission factors for PM2.5 were calculated from the PM10 values using the soil size speciation value for agricultural tilling. The scaling factor for PM2.5 is 0.2 (CitationHouck et al., 1989).
Fertilizer Application
Fertilizer application supplies essential nutrients to crops and ultimately improves crop productivity and yield. PM emissions are generated from fertilizer application through fertilizer particles entrained by the wind and/or soil disturbance by the tractors. In addition, secondary PM is also generated through ammonium due to fertilizer application. PM emissions at SLC polygons (ERj kg yr−1) were calculated as follows (CitationMetroVancouver, 2010):
where AFj is the amount of fertilizer applied at the SLC polygon j (t ha−1). EF is the PM emission factor from fertilizer application, which has a value of 1.09 kg t−1 for PM10, and 0.31 kg t−1 for PM2.5. Data on the amount of fertilizer applied at SLC polygons were derived from the fertilizer application rate and area for each province, assuming a uniform rate of application for all the SLC polygons within a province.
Agricultural Burning
Historically, burning crop residues was the most common practice for clearing harvested lands in Canada and worldwide. However, burning crop residues can emit substantial amounts of PM and other pollutants into the atmosphere. These emissions released from burning residues may have adverse impacts on human health and environment.
PM emissions from agricultural burning are dependent on the type of crop burned and the manner of combustion. The emissions (ERij kg yr−1) can be estimated based on emission factors (EFi for crop type i, kg t−1) () as:
where FLi is the fuel loading for crop type i (t ha−1) and Aij is the area of residue burned per year at SLC polygon j and crop type i (ha yr−1).
For the majority of all reported studies and emission inventories, the fuel loading values for field crops are taken from AP-42 (CitationU.S. EPA, 2003) because fuel loading values are not readily available. However, the mass of crop residue is dependent on the crop growth situation. Therefore, fuel loading has temporal and spatial variations. Ideally, fuel loading should be estimated and recorded at the time of each burn. However, these data are not available, especially for regional and national emission inventories.
To account for the temporal and spatial variations of fuel loadings, the fuel loadings are estimated using the following approach:
where CYi is the yield for crop i (kg ha−1), and RAGi is the ratio of above-ground residue dry matter to yield for crop i. The values of RAG are taken from Appendix 1 in CitationJanzen et al. (2003).
Crop yields are reported by Statistics Canada at the provincial level. If the yield for a crop is not reported, then the national average yield for that specific crop was used to substitute the missing value.
The area of residue burned per year was estimated from the data collected from FEMS. The magnitude of residue burning is significantly dependent on the type of residue. Flax has the highest burning ratio among all crops, since flax residue is fibrous and difficult to handle with current field equipment (CitationChen et al., 2005). However, FEMS only provided a value of burning ratio for each province. Since flax only represents a small ratio of field crops except for Manitoba, we assumed that the burning ratio was the same for all the crops, and burning ratio from FEMS were applied to all the crops and SLC polygons in that province.
Animal Cremation
Disposal of livestock through incineration is one of the three commonly used practices to process dead animals. In Canada, cremation should be done using regulated incinerators. The installation and operation of any incinerator must be in compliance with provincial environment regulations. Animal cremation generates PM containing organic and other toxic materials that are harmful to human health and animal welfare if the cremation is not done using proper incinerators. PM emissions from animal cremation depend on the type of incineration process and quantity of cremated animals. Currently, there is very little information regarding PM emissions from animal cremation.
The emissions in this indicator are estimated based on an emission factor approach as:
where ERi,j is the emission rate of PM10 or PM2.5 at SLC polygon j for animal type i (kg yr−1), EFi is the emission factor (kg head−1) for animal type i, ANij is the animal population for animal type i (head) in the SLC j, and POIi is the harvested percentage of incinerated animal type i.
There are few data on the PM emission factors from animal cremation. The emission factors used in this inventory are taken from two reports (AEA, 2002; CitationVan Heyst, 2005) and are summarized in . No PM10 and PM2.5 emission factors from animal cremation were available. Therefore, the emission factors for PM10 and PM2.5 were determined using particle size fractions for uncontrolled medical waste incineration (CitationU.S. EPA, 1985). The emission factors for poultry cremation used in this inventory come from a combination of these two reports (AEA, 2002; CitationVan Heyst, 2005). The emission factor for pig cremation (AEA, 2002) was assigned to pig and other types of animals. In addition, average animal mass was used to convert the emission factor from mg kg−1 to kg head−1 during calculations (CitationBoadi et al., 2004; CitationLeeson, 2005), The data from FEMS were used to get the proportion of dead animals that were incinerated.
Table 5. Summary of emission factors from animal cremation used in this indicator
Grain Handling
Grain handling refers to all the activities involved in the grain elevators, which includes shipping, receiving, storing, and processing of grain. Grain elevators can be classified as four groups based on their location and function: primary, process, transfer, and terminal elevators.
Significant particulates emitted in grain handling operations come from shipping and receiving, grain dryers, and cleaners. The amount of dust emitted during the various operations depends upon the type of transport mode (truck, railcar, or ship/barge), grain being handled, its quality or grade, its moisture content, the degree of enclosure at loading and unloading areas, and the extent and efficiency of dust containment systems used.
Emissions from grain handling operations are often controlled with cyclones or fabric filters. Recent studies showed that a PM reduction of between 60 and 80% can be achieved (CitationU.S. EPA, 2003). In this indicator, a 75% control efficiency was assumed and used for all the elevators.
Emissions from the four types of grain elevator were calculated using emission factors based on the mass of grain handled as
where ER is the emission rate of either PM10 or PM2.5 (kg yr−1), EF is the emission factor for grain handling (kg t−1), and M is the mass of grain handled (t yr−1).
All particulate emission factors were taken from the AP-42 (CitationUS EPA, 2003) and applied for all the elevators. summarizes the emission factors used in this indicator along with the control assumptions.
Table 6a. Uncontrolled PM emissions factors and assumed control efficiencies or primary elevators
Table 6b. Uncontrolled PM emissions factors and assumed control efficiencies for process elevators
Table 6c. Uncontrolled PM emissions factors and assumed control efficiencies for transfer elevators
Table 6d. Uncontrolled PM emissions factors and assumed control efficiencies for terminal elevators
The base quantities required are grain production for four categories of elevator: primary, process, transfer, and terminal. These data can be obtained or extracted from Annual Grain Trade of Canada by Statistics Canada. Percentages of transportation modes using for grain receiving and shipping are obtained either from the estimates from an expert (M. Hemmes, personal communication) or from Grain Trade of Canada. The PM emissions from grain handling were calculated at a provincial scale because of data availability.
Results and Discussion
In 2006, PM emissions from the Canadian agricultural sector were approximately 652.6 kt for PM10 and 158.1 kt for PM2.5. The majority of PM emissions were from the Canadian Prairies—Saskatchewan and to a lesser extent Alberta, and Manitoba (; also supplemental information). These three provinces also represent approximately 82% of agricultural land area and activity in Canada.
Figure 1. National and provincial emissions of PM10, and PM2.5 over the 25-year period, 1981–2006. PM emissions from Nova Scotia, New Brunswick, and Prince Edward Island (PEI) are combined as Atlantic (Atl) Canada, given the relatively small contributions from these provinces.
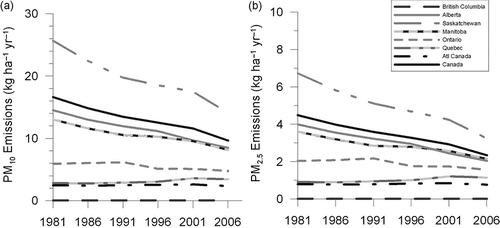
With the exception of Quebec, national and provincial PM emissions decreased for PM10 and PM2.5 over the 25-year period, 1981–2006 (; supplemental information). Nationally, PM emissions from agriculture decreased by 442.8 kt (40 %) for PM10 and 137.7 kt (47%) for PM2.5, between 1981 and 2006 (). The greatest decreases in PM emissions were primarily attributed to changes in land-use practices, which were primarily due to the conversion to reduced and no-till cropping systems, as well as decreases in the area of summerfallow. The most intense cases of wind erosion were reported in the semi-arid prairies, where the growing season is short and summerfallow was common. The introduction of windbreaks and beneficial management practices (e.g., retention of crop residues, use of perennial forages or cover crops) to protect the field surface contributed to reduced wind erosion. Indeed, the Prairie Shelterbelt Program has actively contributed to land conservation since 1901 by providing both technical services and adapted tree and shrub seedlings to farmers in the Prairie Provinces.
Table 7. Changes in national and provincial PM10, and PM2.5 annual emission rates between 1981 and 2006 for categories reported for all Census years (wind erosion, land preparation, crop harvest, grain handling, fertilizer application, animal feeding operations)
Between 1981 and 2006 tillage activity and area in the Prairie Provinces dramatically decreased. In 2006 approximately 50% of total land area was cultivated using no-till and 30% was cultivated using reduced tillage practices. Land preparation, crop harvesting, and wind erosion collectively represented approximately 95% of the total PM10 emissions in 2006 (). Crop residue burning contributed 11% of PM2.5 in addition to land preparation, crop harvesting, and wind erosion. The contribution of grain handling and animal feeding operation was quite minimal (<2.4%) for both PM10 and PM2.5 emissions.
The reductions in PM emissions from field operations are a secondary positive outcome provided from these improved land management practices. The initial intended purposes of no-till and reduced tillage practices were in response to cropland degradation including loss of soil carbon, extensive erosion, and plant mortality due to low soil moisture during drought periods. These practices are most commonly used in the Prairie Provinces, which frequently experience drought conditions due to the subhumid climate. Although PM emissions have decreased nationally between 1981 and 2006, Quebec demonstrated a net increase in PM emissions, although the provincial emissions were very low. This was primarily due to a 111% increase in the area of cropland: mainly grain corn, which contributed to a 24% and 20% increase in PM emissions from land preparation and crop harvesting activities, respectively, within the province. Additionally, a 5% increase in animal husbandry, particularly within the swine and poultry industries, contributed to an increase in PM emissions over this period. These increases in PM emissions have more impact in Quebec as reduced tillage practices were only mildly adopted, due to their marginal benefits under the temperate climate. In addition to increased PM emissions in Quebec, emissions from animal feeding operations increased by approximately 19% for PM2.5 and 22% for PM10 throughout Canada. However, these increases in PM emissions were offset by the much larger decreases in PM emissions associated with the more predominant field-based activities ().
This agro-environmental indicator suffers from a lack of experimentation, which would assist in building a common database of PM emission factors related to management practices (e.g., tillage, harvest). Indeed, having emission factors specific to machinery, for different soil texture and environmental conditions (soil moisture, relative humidity, wind speed, air temperature, etc.), would speed up the definition of beneficial management practices accounting for PM emissions. Similarly, for modeling the impact of wind erosion on PM emissions, field experiments would be very beneficial. The proportions of the different PM categories in the overall emissions need to be verified.
Although the indicator would tremendously benefit from updated emission factors based on recent experimentations, the actual results already provide us with key information. All the management practices that reduced soil erosion and restored soil organic matter were beneficial for reducing PM emissions, practices such as installing and maintaining wind breaks, reducing summerfallow, increasing cover crop area, employing no-till or reduced tillage methods in appropriate areas, and avoiding residue burning.
However, no direct inference on the trends in local air quality can be made using these large-scale and multi-decadal PM emissions trends. Temporal variability on less than annual time scales was beyond the scope of the current work, though this variability can have a substantial impact on emissions levels on these time scales. To assess the impact of agriculture intensification would require collecting higher resolution spatial allocation maps, then combining the results in emission processing systems such as SMOKE (CitationHouyoux et al., 2000) so that the gridded emissions could be used as input in a regional air-quality model such as AURAMS (Markar et al., 2008), to compute the concentrations.
Conclusions
A comprehensive APMEI was developed to estimate PM emissions from agriculture in Canada. A detailed emissions inventory pinpoints where extensive reductions have been made and where remediation needs to be addressed and prioritizes the research gaps. The general outcome from the results of the APMEI indicates that PM emissions decreased significantly over a 25-year period between 1981 and 2006. The strongest example of these changes in PM emissions is associated with land preparation and tillage practices adopted in the Prairie Provinces. Although this indicator is very useful in determining PM emissions on a large scale, the paucity of emission factors relevant to Canadian conditions somewhat limits the full utility of the PM emissions estimates. More research on agricultural PM emissions and emissions factors relevant to Canadian conditions could enhance the APMEI and contribute to model development of PM emissions.
uawm_a_672058_sup_25584920.pdf
Download PDF (866.8 KB)Acknowledgments
This work was funded by the National Agri-Environmental Health Analysis and Reporting Program of Agriculture and Agri-Food Canada. The authors extend thanks to those who provided invaluable help and advice: Ted Huffman, Brian McConkey, Warren Eilers, Devon Worth, Ron vanHarleem, Xavier Verge, Sonja Fransen, and Sébastien Blouin from Agriculture and Agri-Food Canada, John Ayres, Susan Charles, and Sanjay Girdhar from Environment Canada, Steve Francis from California Air Resources Board, and Mark Hemmes from Quorum Corporation.
References
- AEA Technology . 2002 . Atmospheric emissions from small carcass incinerators , Abingdon , , UK : AEA Technology Environment . Report produced for the Department for Environment, Food and Rural Affairs
- American Thoracic Society . 1998 . Respiratory health hazards in agriculture . Am. J. Respir. Crit. Care Med. , 158 : s2 – s76 . Environment Canada. Final Report.
- Boadi , D., K. H. , Ominski , D. L. , Fulawaka and Wittenberg , K. M. 2004 . Improving estimates of methane emissions associated with enteric fermentation of cattle in Canada by adopting an IPCC (Intergovernmental Panel on Climate Change) tier-2 methodology
- Bouchet , V. S. , Cousineau , S. , Crevier , L. P. , Duhamel , A. , Racine , J. , Sassi , M. and Moran , M. 2009 . “ Analysis of current and projected air quality levels in Canada with AURAMS ” . In Proceedings of the 89th American Meteorological Society Annual Meeting and 11th Conference of Atmospheric Chemistry , Phoenix , AZ : Paper 6.4 .
- Canadian Centre for Occupational Health and Safety. 1999. OSH answers: Farmer's lung http://www.ccohs.ca/oshanswers/diseases/farmers_lung.html (http://www.ccohs.ca/oshanswers/diseases/farmers_lung.html) (Accessed: 9 February 2012 ).
- Canadian Grain Commission. 2010. Grain elevator and storage capacity totals combined for all provinces and elevator types, 1962 to 2010 http://www.grainscanada.gc.ca/wa-aw/geic-sgc/summary-results-sommaire-resultats-eng.asp (http://www.grainscanada.gc.ca/wa-aw/geic-sgc/summary-results-sommaire-resultats-eng.asp) (Accessed: 9 February 2012 ).
- CARB . 1997 . Section 7.12: Windblown dust—Agricultural lands. Emission inventory procedural manual volume III: Methods for assessing area source emissions , Sacramento : California Air Resource Board .
- CARB . 2003 . Emission inventory procedural manual volume III: Methods for assessing area source emissions , Sacramento : California Air Resource Board .
- Chen , Y. , Tessier , S. , Cavers , C. , Xu , X. and Monero , F. 2005 . A survey of crop residue burning practices in Manitoba . Appl. Eng. Agric. , 21 : 317 – 323 .
- Donham , K. J. and Thelin , A. 2004 . Agricultural medicine: Occupational and environmental health for the health professions , Victoria : Blackwell Publishing Asia .
- Eilers , W. , MacKay , R. , Graham , L. and Lefebvre , A. 2010 . Environmental sustainability of Canadian agriculture: Agri-Environmental Indicator Report Series, Report 3 , Ottawa : Agriculture and Agri-Food Canada .
- Environment Canada. 2008. Criteria air contaminants—2006 Emission summaries http://www.ec.gc.ca/pdb/cac/Emissions1990-2015/emissions_e.cfm (http://www.ec.gc.ca/pdb/cac/Emissions1990-2015/emissions_e.cfm) (Accessed: 9 February 2012 ).
- Houck , J. E. , Chow , J. C. , Watson , J. G. , Simons , C. A. and Pritchett , L. C. 1989 . Determination of particle size distribution and chemical composition of particulate matter from selected sources in California, Final report , Reno , NV : Desert Research Institute & OMNI Environmental . Prepared for California Air Resources Board, Agreement no. A6–175–32
- Houyoux , M. R. , Vukovich , J. M. , Coats , C. J. Jr. and Wheeler , N. J. M. 2000 . Emission inventory development and processing for the seasonal model for the regional air quality (SMRAQ) project . J. Geophys. Res. , 105 : 9079 – 9090 .
- Huffman , T. and Coote , D. 2010 . “ Soil cover ” . In Environmental sustainability of canadian agriculture: Agri-Environmental Indicator Report Series—Report 3 , Edited by: Eilers , W. , MacKay , R. , Graham , L. and Lefebvre , A. Ottawa : Agriculture and Agri-Food Canada .
- Janzen , H. H. , Beauchemin , K. A. , Bruinsma , Y. , Campbell , C. A. , Desjardins , R. L. , Ellert , B. H. and Smith , E. G. 2003 . The fate of nitrogen in agroecosystems: An illustration using Canadian estimates . Nutr. Cycl. Agroecosyst. , 67 : 85 – 102 .
- Jenkins , B. 1996 . Atmospheric pollutant emission factors from open burning of agricultural and forest biomass by wind tunnel simulations , Davis : University of California, Davis . Tables 4.1.1 to 4.1.8
- Leeson , S. 2005 . PM Emissions from the Canadian poultry industry II: Activity factors , Environment Canada. Draft report 5 .
- Lim , T.-T. , Heber , A. J. , Ni , J.-Q. , Gallien , J. X. and Xin , H. October 12–15 2003 . “ Air quality measurements at a laying hen house: Particulate matter concentrations and emissions ” . In American Society of Agricultural Engineers Proceedings of the 3rd International Conference on Air Pollution from Agricultural Operations October 12–15 , Research Triangle Park , NC
- Makar , P. A. 2008 . “ High resolution nested runs of the AURAMS model with comparisons to PrAIRie2005 field study data ” . In Air pollution modeling and its application XIX , Edited by: Borrego , C. and Miranda , A.I. Dordrecht, The Netherlands: Springer Science .
- MetroVancouver. 2010. 2005 Lower Fraser Valley air emissions. Inventory & Forecast and BackCast. Detailed listing of results and methodology http://www.metrovancouver.org/about/publications/Publications/2005-LFV-Air_ Emissions_Inventory-Forecast-Backcast.pdf (http://www.metrovancouver.org/about/publications/Publications/2005-LFV-Air_ Emissions_Inventory-Forecast-Backcast.pdf) (Accessed: 9 February 2012 ).
- Pattey , E. , Qiu , G. and van Haarlam , R. 2010 . “ Particulate matter ” . In Environmental sustainability of canadian agriculture: Agri-Environmental Indicator Report Series—Report 3 , Edited by: Eilers , W. , MacKay , R. , Graham , L. and Lefebvre , A. Ottawa : Agriculture and Agri-Food Canada .
- Ramanathan , V. and Feng , Y. 2009 . Air pollution, greenhouse gases and climate change: Global and regional perspectives . Atmos. Environ. , 43 ( 1 ) : 37 – 50 .
- Roumeliotis , T. S. and Van Heyst , B. J. 2007 . Size fractionated particulate matter emissions from a broiler house in southern Ontario, Canada . Sci. Total Environ. , 383 : 174 – 182 .
- Samet , J. and Krewski , D. 2007 . Health effects associated with exposure to ambient air pollution . J. Toxicol. Environ. Health , 70 : 227 – 242 .
- Saxton , K. E. 1996 . Agricultural wind erosion and air quality impacts: a comprehensive research program . Am. J. Altern. Agric. , 11 ( 2–3 ) : 64 – 70 .
- Seedorf , J. 2004 . An emission inventory of livestock-related bioaerosols for lower Saxony, Germany . Atmos. Environ. , 38 : 6565 – 6581 .
- Seedorf , J. , Hartung , J. Schroeder , M. 1998 . Temperature and moisture conditions in livestock buildings in Northern Europe . J. Agric. Eng. Res. , 70 : 49 – 57 .
- Statistics Canada. 2006. Farm Environmental Management Survey (FEMS) http://www.statcan.gc.ca/cgi-bin/imdb/p2SV.pl?Function=getSurvey&SDDS=5044&lang=en&db=imdb&adm=8&dis=2 (http://www.statcan.gc.ca/cgi-bin/imdb/p2SV.pl?Function=getSurvey&SDDS=5044&lang=en&db=imdb&adm=8&dis=2)
- Takai , H. , Pedersen , S. Johnsen , J. O. 1998 . Concentrations and emissions of airborne dust in livestock buildings in Northern Europe . J. Agric. Eng. Res. , 70 : 59 – 77 .
- U.S. Environmental Protection Agency . 1985 . Compilation of air pollutant emission factors. Volume I. Stationary point and area sources. AP-42 , 4th , Research Triangle Park , NC : U.S. Environmental Protection Agency .
- U.S. Environmental Protection Agency . 1998 . National air pollutant emission trends, procedures document, 1990–1996 , Research Triangle Park , NC : U.S. Environmental Protection Agency . EPA-454/R-98-008
- U.S. Environmental Protection Agency. 2003. Compilation of air pollutant emission factors. Volume I. stationary point and area sources. AP-42, 5th ed. Washington, DC: U.S. EPA http://www.epa.gov/ttn/chief/ap42/ch09/index.html (http://www.epa.gov/ttn/chief/ap42/ch09/index.html) (Accessed: 9 February 2012 ).
- U.S. Environmental Protection Agency . 2004 . Air quality criteria for particulate matter (October 2004). EPA 600/P-99/002aF-bF , Washington , DC : U.S. Environmental Protection Agency .
- Van Heyst , B. 2005 . Evaluation of emission factors for the improvement of the estimation methodology for particulate matter from agricultural poultry industry , Canada , , Guelph : University of Guelph . Report prepared for Environment
- Woodruff , N. P. and Siddoway , F. H. 1965 . A wind erosion equation . Soil Sci. Soc. Am. Proc. , 29 : 602 – 608 .