Abstract
The acceleration of the composting process and the improvement of compost quality have been explored by evaluating the efficacy of various additives, inoculating with specific microorganisms and the application of various biosurfactants. The magnesium-aluminum silicate attapulgite is a low-cost potential composting additive, but its effects on aerobic composting are unknown. This study investigated the effects of attapulgite application on compost production and quality during the aerobic composting of chicken manure. Addition of attapulgite significantly increased the temperature (p < 0.05) while it reduced compost total organic carbon (TOC) and seed germination indices (GIs) throughout the process. Its addition enhanced nitrate concentrations, promoted organic matter degradation, increased seed germination indices, and accelerated the composting process. Interestingly, attapulgite addition did not increase the population of ammonia-oxidizing bacteria. These results suggest that attapulgite is a good additive for the composting industry.
We investigated the addition of two forms of attapulgite during aerobic composting of chicken manure to determine their effects under strict composting environmental parameter control. Our results provides primary evidence that attapulgite may have potential for application in the composting industry.
All treatments showed no increase within the first 15 days. However, emissions increased for all treatments within 15–45 days, reaching approximately 6300, 2000, and 4000 mg/m2 from the control, artifactitious attapulgite, and raw attapulgite treatments, respectively.
A considerable amount of organic waste is produced in China each year. The annual amount of generated animal manure alone is estimated to be between 2500 and 3000 million wet metric tonnes (CitationZhang et al., 2011). However, less than 20% of the total animal manure is properly disposed of, and the rest remains untreated, posing serious threats to the environment (Zhang et al., 2008).
Composting is the biological degradation and transformation of organic materials under controlled conditions to promote aerobic decomposition (CitationGu et al., 2011). In recent years, composting has been presented as an environmental friendly and sustainable alternative to manage and recycle organic solid wastes, with the aim of obtaining a quality product to be used as an organic amendment in agriculture (CitationGu et al., 2011). However, its concomitant generation of odorous gases has become a serious threat to local environments.
Composting is one of the few natural processes capable of stabilizing organic wastes (CitationBarrington et al., 2002). However, nitrogen losses occurring during composting are a key issue. Nitrogen (N) volatilization reduces the fertilizer value of the waste, and constitutes an important economic loss. During the aerobic composting of poultry waste/straw stalks, sludge/straw stalks, and poultry waste/sawdust, the total N lost was 16–76% (CitationBarrington et al., 2002).
To determine effective ways to accelerate composting and improve compost quality, numerous methods to control and reduce nitrogen loss during composting have been explored. Currently two methods are widely used. The first involves adjusting the technology and conditions of composting, for example, ventilation, the carbon-to-nitrogen ratio (C/N), the humidity, and temperature (CitationDiaz et al., 2007; CitationParé et al., 1998; CitationYang et al., 2005). The second method involves adding amendments during composting, including physical amendments (such as turf, zeolite, and basalt) and chemical amendments (such as ferric chloride, superphosphate, phosphoric acid, and magnesium hydroxide) (CitationBishop and Godfrey, 1983; CitationMartins, 1992; CitationRen et al., 2008). For example, ammonia volatilization can be reduced by covering the compost with zeolite and coconut shell (CitationKithome et al., 1999; CitationMahimairaja et al., 1994). It has been shown that addition of magnesium chloride, calcium chloride, and magnesium sulfate in poultry manure can reduce ammonia volatilization (CitationWitter and Kirchmann, 1989), and nitrogen can be reserved by adding ferric chloride and calcium superphosphate (CitationLin et al., 2008; CitationRaviv et al., 2002). In addition, many studies have shown that addition of microbial agents in composting can reduce nitrogen loss (CitationKuroda et al., 2004; CitationLi et al., 2003). However, the methods just mentioned also have some disadvantages, such as instability, high cost, poor quality, and safety of the resulting compost.
Attapulgite is a crystalloid hydrous magnesium-aluminum silicate mineral with a special laminated chain structure in which crystalline lattice displacement exists. Thus, the crystals contain varying quantities of Na+, Ca2+, Fe3+, and Al3+, and are present as needles, fibers, or fibrous clusters. Attapulgite has very good colloidal properties with specific features including dispersion, high temperature endurance, salt and alkali resistance, and also high adsorbing and decoloring capabilities. Attapulgite also has certain plastic and adhesive characteristics.
Although attapulgite is a relatively low-cost composting amendment, ranging in cost between $150 and $800 per tonne, its effects on aerobic composting are unknown. Our study showed adding attapulgite, zeolite, or another adsorbent could reduce ammonia loss by about 50% in animal manure aerobic composting process by reducing ammonia volatilization. (CitationLefcourt and Meisinger, 2001; CitationYang et al., 2011). In the present study, we investigated the addition of two forms of attapulgite during aerobic composting of chicken manure to determine their effects under strict composting environmental control. Therefore, the modified attapulgite has been used to reduce the loss of nitrogen in the process of aerobically composted chicken manure. It has much stronger adsorptivity features (the surface area has increased by 300 times more than common attapulgite) and very good promoted digestion. (Due to the 300 times increased surface area, the modified attapulgite has much stronger absorbability and can better promote digestion.)
Materials and Methods
Attapulgite
Raw attapulgite was obtained from Mingguang city, Anhui Province of China. Raw attapulgite and hydrochloric acid (1 M) were mixed in a ratio of 1:1 w/v at 1000 rev/min for 20 min. The resulting product was flushed by pure water up to pH 7, vacuum-dried at 80°C for 24 hr, finely ground, and finally calcined at 600°C for 2 hr to obtain the modified attapulgite. Selected characteristics of the raw and modified attapulgites are shown in .
Table 1. Physicochemical properties of artifactitious attapulgite and raw attapulgite (dry weight basis determined on triplicate samples)
Composting materials
Chicken manure and mushroom residues were obtained from a chicken farm at the Institute of Animal Science, Guangdong Academy of Agricultural Sciences, and from a Xinghe edible fungus factory in Dongguang city, Guangdong province, respectively. Rice husks and rice bran were obtained from the Institute of Rice, Guangdong Academy of Agricultural Sciences. Selected characteristics of the materials are shown in .
Table 2. Physicochemical characteristics of the composting raw materials (dry weight basis determined on triplicate samples)
Composting device
The reactor size was 0.8 m × 2 m × 1.5 m (), and the working volume was 2.4 m3. For gas exchange, a ventilator was installed at the upper right corner of the reactor. In order to maintain the appropriate oxygen content for the composting (CitationHogland et al., 2003; CitationKeener et al., 2005), forced ventilation was performed at a rate of 3000 L/min at 17 min after every 0.5 hr. Stirring was conducted every 6 hr by upending the reactor vessel. The composting temperature was automatically monitored using a platinum electrode (PT-100).
Experimental design
The fresh chicken manure, rice husks, rice bran, and mushroom residues were mixed in a ratio of 5:1:1:3, v/v/v/v. To mix compost materials evenly, attapulgite and rice bran were mixed at various ratios. After that, rice husks, mushroom residues, and chicken manure were added successively. The resulting product was used as composting feedstock and had a moisture content of 55% (CitationJiminez and Garcia, 1992). Three treatments were applied to 1000 kg of compost material in each reactor: (i) modified attapulgite sprinkled onto the compost at a final rate of 2 g/kg on a fresh weight basis (T1); (ii) raw attapulgite sprinkled onto the compost at a final rate of 2 g/kg on a fresh weight basis (T2); and (3) a nonamended control (CK). Our study has shown that adding attapulgite at 2, 5, or 10 g/kg all resulted in 90% ammonia fixation. To minimize compost cost, 2g/kg was selected for further test. Each treatment was repeated in three separate reactors.
Compost Sampling and Analysis
Samples were taken at 0, 1, 3, 5, 12, 15, 25, 30, and 45 days from different reactor depths, mixed as a composite sample, and then divided into two subsamples. One subsample set was immediately extracted and centrifuged with the supernatant, then used to determine pH, electrical conductivity (EC), ammonium nitrogen, nitrate nitrogen, seed germination indices (GIs), and the ammonia-oxidizing bacterial population. The other subsample set was air-dried and ground to pass through a 1-mm sieve, and was used to measure total organic carbon (TOC) and total nitrogen (TN) contents.
A fresh subsample was extracted with distilled water or 1 M KCl at a ratio of 1:10 for 1 hr and then centrifuged. After centrifugation, the water-based supernatant was used to measure pH (PB-10 Sartorius) and EC (DDS-11A, Shanghai Hongyi). The KCl-based supernatant was analyzed for ammonium nitrogen and nitrate nitrogen using a flow injection analyzer (AMS France Futura).
The number of amoA copies present was determined by quantitative polymerase chain reaction (qPCR) based on an ABI PRISM7500 real-time PCR system (ABI, Applied Biosystems, USA). Some templates were diluted 10- or 100-fold. Each 25 μL qPCR contained 2 μL template, 12.5 μL SYBR Premix Ex Taq (Takara Bio Inc., Shiga, Japan), 0.5 μL ROX Reference Dye II (50×), 1 μL of both Arch-amoAR (5′-GCGGCCATCCATCTGTATGT) and Arch-amoAF (5′-ST AATGGTCTGGCTTAGACG) (CitationFrancis et al., 2007), and 2 μL ddH2O. The amplification regime comprised a predenaturation step (95°C/30 s), followed by 40 cycles of 94°C/30 s, 58°C/45 s, 72°C/45 s. A melting-curve analysis was performed over the temperature range 65–98°C (0.2°C per read, 6 s hold), and the fluorescence was determined during each cycle at 83°C. A standard curve based on known numbers of amoA copies was prepared as described by CitationHe et al. (2007). The R 2 of the standard curve was 0.99 with a slope of –3.5, and was linear over eight orders of magnitude.
Seed GIs were determined as follows. Distilled water (50 mL) was added to 5 g of fresh compost, which was mechanically shaken for 1 hr and then filtered. Compost extract (5 mL) was added to culture dishes (9 cm diameter) containing two pieces of filter paper. In total, 20 radish seeds were evenly distributed on the surface of the filter paper and then incubated in the dark at 30°C for 48 hr. The control for the GI index test was water. The procedures were replicated three times for each sample. Root lengths and germination rates were measured and GI was calculated as described previously (CitationGu et al., 2011).
A 0.1000-g air-dried sample was digested in an H2SO4–H2O2 mixture with the digest used to determine total N concentrations according to organic fertilizer technology standards (NY525–2002). Another 0.1000-g air-dried sample was used to measure TOC concentrations, again in accordance with the standards (NY525–2002).
Gas Measurements
Nitrous oxide (N2O) was sampled during composting using a static chamber technique (CitationLi et al., 2002), while ammonia was sampled using the air collector–acid absorption method (GB/T 14668-93, China). Gas samples for N2O measurement were taken from the collection trap eight times during the experiment (on days 1, 3, 5, 12, 15, 25, 30, and 45). Gas chromatography (HP-Chemistation 5890; Hewlett-Packard, USA) and electron capture detector (GC/ECD; Hewlett-Packard) were combined to achieve a quantitative analysis of nitrous oxide discharge. The gas discharge flux was calculated as the mass of gas discharged per minute per unit area, based on the relationship:
where f represented the gas flux [μg/(m2 h)], Δm the N mass in gaseous nitrous oxide (μg), A the cross-sectional area (m2), h the static mass height (m), the change in the gas concentration per minute (×1 0-9/min), M = 0.64 g/mol, 273 /
, and T1
and T2
the temperature (°C) in the mass at the time of collection of, respectively, the first and last samples. The ammonia trapped in boric acid was titrated using sulfuric acid (H2SO4).
Data Analysis
The mean and standard error (S.E.) of pH, TOC, C/N, EC, GI, ammonium nitrogen, nitrate nitrogen, ammonium emissions, and size of ammonia-oxidizing bacterial population at various sampling times for the CK, T1 and T2 treatments were calculated. A one-way analysis of variance (ANOVA) was used to test the effect varying the treatment. Experimental data were statistically analyzed using Microsoft Excel 2007 (Microsoft, USA) and SPSS v13.0 (IBM, USA).
Results and Discussion
Temperature of compost
Temperature variations of the composting materials with time in the different treatments are shown in Temperatures in the T1, T2, and CK treatments rapidly increased during the initial stage of composting (first 6 days), which is attributed to strong heat release during microbial activity (CitationGu et al., 2011; CitationHaug, 1993).
The highest temperature (74ºC) from T1 and T2 treatments occurred at day 3, while for CK treatment the highest temperature (70ºC) appeared at day 4. A temperature of 60°C or higher was maintained for 11 consecutive days for all three groups. However, a temperature of 55°C or higher was maintained for 16 consecutive days in the T1 treatment, but for only 14 and 12 consecutive days for the T2 and the CK treatments, respectively.
Overall, higher temperatures were observed from the modified attapulgite amended treatment over the 45-day composting period.
pH of compost
The T1 and T2 treatments exhibited rapid increases in pH of approximately 1.1 and 0.9 pH units, respectively (from the initial 7.6), within the first 12 days, and a decline from day 12 to day 30, with a relatively stable pH (7.5) throughout the remaining 15 days (). However, the CK treatment exhibited a rapid increase in pH of approximately 0.6 pH units (from the initial 7.6) within the first 5 days, and a decline from day 5 to day 15, with a relatively stable pH (7.0) throughout the remaining 30 days. These results suggest that attapulgite addition did not substantially affect compost pH throughout the process when compared with the untreated control. However, Lefcourt reported chemical changes in the slurry in response to the addition of amendments at 0.4, 1.0, 2.5, and 6.25% by weight. Alum treatment retained ammonia by reducing the slurry pH to 5 or less (CitationLefcourt and Meisinger, 2001).
TOC content of the compost
All treatments resulted in continued declines in total C concentrations within the first 15 days, with relatively stable values throughout the remaining 30 days (). These declines are ascribed to increased microorganism activity, which would have gradually consumed degradable carbon sources during the composting process (CitationChefetz et al., 1996; CitationDomeizel et al., 2004; CitationGao et al., 2005).
Figure 4. Influence of attapulgite on the evolution of the total organic carbon (TOC) during aerobic composting. The error bars represent the standard deviation.
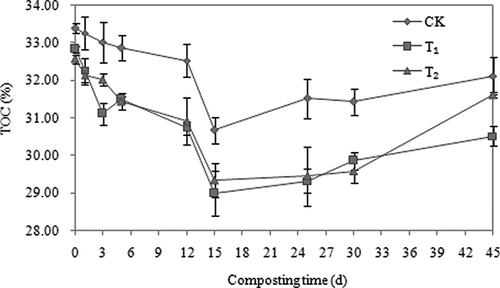
On the same sampling dates, reduced total organic C concentrations were observed in the samples with added modified attapulgite compared with those from the control treatment (). Total organic C concentrations in the samples to which modified attapulgite had been added were reduced by approximately 14% after 15 days of composting, whereas those in the control treatment decreased by approximately 7% (), indicating that modified attapulgite addition enhanced organic C degradation and consequently accelerated the composting process. Significant differences (p < 0.05) in TOC were observed between T1 and the CK and T2 attapulgite treatments, while the CK and T2 attapulgite treatments did not significantly differ from one another with respect to TOC (p > 0.05).
C/N of the composting materials
Similarly to changes in total organic C concentrations, continued declines in C/N were observed in all treatments within the first 15 days, with a relative stabilization throughout the remaining 30 days. C/N declined faster in the treatments with added attapulgite compared with the control (). At a later stage in composting, N loss was higher in CK than in either of the treatments, resulting in a higher C/N developing in CK than in either T1 or T2. The two treatments differed significantly from one another (p < 0.05) with respect to C/N.
EC of the compost
EC is an indicator of soluble salt concentrations in soils or organic wastes. Soil salinity and biotoxicity problems may arise from land application of organic wastes such as composts with high EC levels (CitationBao, 2000). In general, all treatments resulted in rapid increases in EC during the first 6 days of composting, followed by a subsequent decline in the following 7–45 days (). No significant differences (p > 0.05) for EC were observed between the treatments. Slightly higher EC values were observed in the T1 group throughout the composting process. These results suggest that the application of low levels of compost added with attapulgite would not cause problems.
Seed germination index
GI is an important parameter. The compost is considered nonmature with a possible biotoxicity to plants when the GI is less than 50% (CitationZucconi and de Bartholdi, 1987). It is considered fully mature and nontoxic to plants when the GI is greater than 85% (CitationRiffaldi et al., 1996).
Compared with the CK treatment, the modified attapulgite treatment resulted in a slightly higher GI at the same sampling dates. GI exceeded 50% for all treatments after 15 days of composting. After 45 days, GI values exceeded 100% and 90% from the T1 group and CK group, respectively (). Significant differences (p < 0.05) in GI were observed between the treatments, but the two attapulgite addition treatments did not differ significantly from one another (p > 0.05).These results suggest that the addition of attapulgite can improve composting conditions and compost quality.
Ammonium nitrogen and nitrate nitrogen contents of the compost
All treatments resulted in rapid increases in ammonium-N concentrations within the first 12 days of composting, followed by declines throughout the remaining 33 days (). The peak concentrations were 6.35, 5.98, and 4.82 g/kg at day 12 in the T1, T2, and CK treatments, respectively.
Figure 8. Influence of attapulgite on the evolution of the ammonium nitrogen during aerobic composting. The error bars represent the standard deviation.
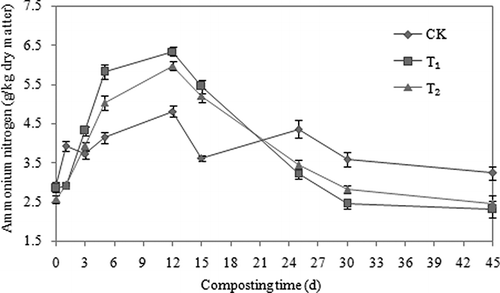
In general, nitrate-N concentrations showed an increasing trend for the two attapulgite treatments within the first 15 days of composting (), followed by declines throughout the remaining 30 days. However, they showed a declining trend for the control treatment throughout the composting process. The peak values of 3.01 and 2.76 g/kg occurred at day 15 of composting in the T1 and T2 treatments, respectively.
Ammonia and nitrogen dioxide emissions
Ammonia emissions are shown in Ammonia emissions increased for the control treatment throughout the composting process, reaching 900 mg/m2 at 45 days. However, emissions increased for the two attapulgite addition within 12 days, followed by relatively stable levels throughout the remaining 30 days. The concentrations were 150 and 190 mg/m2 at day 12 from the modified attapulgite amended and raw attapulgite addition treatments, respectively. CitationLefcourt and Meisinger (2001) chose zeolite, a cation exchanger, to act as an absorber of ammonium from diary slurry, and showed that it was most effective when placed on top of the top of the compost pile. In contrast, a layer of mature sewage–sludge–straw compost was quite ineffective. The absorption of ammonia increased the nitrogen content of the zeolite by up to 2.5%, while a similar experiment using clay soil doubled the nitrogen content of the absorbent material (CitationWitter and Lopez-Real, 1988).
Figure 10. Influence of attapulgite on the evolution of the ammonium emissions during aerobic composting.
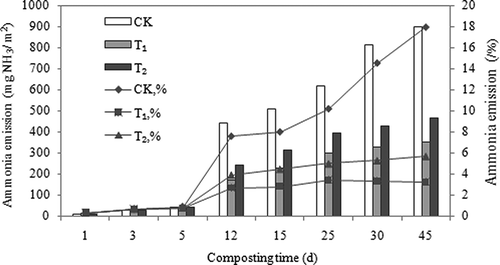
Nitrogen dioxide emissions are shown in All treatments showed no increase within the first 15 days. However, emissions increased for all treatments within 15–45 days, reaching approximately 6300, 2000, and 4000 g/m2 from the control, modified attapulgite, and raw attapulgite treatments, respectively. One possible explanation for this may be the higher temperatures in the modified attapulgite amended groups, which is an important control on the activity of nitrite oxidoreductase, a key enzyme responsible for the oxidation of NO2 - to NO3 - (CitationFukumoto et al., 2006).
Figure 11. Influence of attapulgite on the evolution of the nitrogen dioxide emissions during aerobic composting.
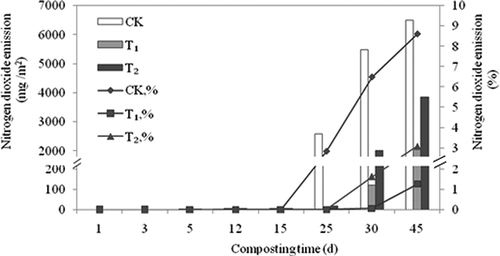
Taken together, these data indicated that attapulgite can reduce ammonia and nitrogen dioxide emissions during the composting process and that modified attapulgite may have a greater effect than raw attapulgite.
Ammonia-oxidizing bacteria population during composting
Ammonia-oxidizing bacteria are responsible for the rate-limiting step of the nitrification process. To investigate their populations during the composting process, a real-time PCR system was used (CitationHe et al., 2007). In all three treatments, populations tended to increase during the first 30 days and then declined from 30 to 45 days (). Peak values were approximately 1.00E+08, 1.00E+08, and 8.00E+06 for the CK, T2, and T1 treatments, respectively (). The size of the ammonia-oxidizing bacteria population was significantly reduced by the T1 treatment at the 15-, 25-, and 30-day sampling times, presumably because of the effectiveness of the modified attapulgite as an absorption medium. Hassen and his coworkers found that the tested clay substrate appeared as a relatively moderate phage adsorbent since the quantity of adsorbed phage averaged 98.2 ± 0.88%. He also reported that the retention capacity of purified clay Kbl2 appeared to be 60% for Pseudomonas aeruginosa (CitationHassen et al., 2003).
Conclusions
The addition of attapulgite increased composting temperature, organic matter degradation, and nitrate nitrogen content, enhanced organic matter decomposition, accelerated the composting process, and improved the compost quality to a certain extent over the entire 45-day composting period. The addition of attapulgite did not increase the ammonia-oxidizing bacteria population during composting. Compared with the untreated control, attapulgite addition did significantly affect (p < 0.05) compost TOC and GI throughout the composting process. The attapulgite treatment also increased GI by 10%. The results of this study suggest that attapulgite may have potential for application in the composting industry, and modified attapulgite may be better than raw attapulgite owing to its better performance during composting.
Acknowledgments
This project was partially supported by the Central Foundation of Agricultural Science and Technology Production (2010E00079) and Key Scientific Research Base of Guangdong Province (2009A060800026). The authors thank Ruiping Jiang and Ping Li for their assistance with field sampling and chemical analysis.
References
- Bao , S.D. 2000 . Soil and Agro-Chemistry Analysis , Beijing : China Agricultural Press .
- Barrington , S. , Choiniere , D. , Trigui , M. and Knight , W. 2002 . Effect of carbon source on compost nitrogen and carbon losses . Bioresource Technol. , 83 ( 3 ) : 189 – 194 . doi: 10.1016/S0960-8524(01)00229-2
- Bishop , P.L. and Godfrey , C. 1983 . Nitrogen transformations during sludge composting . Biocycle , 24 ( 5 ) : 34 – 39 .
- Chefetz , B. , Hatcher , P.G. , Hadar , Y. and Chen , Y. 1996 . Chemical and biological characterization of organic matter during composting of municipal solid waste . J. Environ. Qual. , 25 ( 4 ) : 776 – 785 . doi: 10.2134/jeq1996.0047242500 2500040018x
- Diaz , L.F. , De Bertoldi , M. and Bidlingmaier , W. 2007 . Compost Science and Technology , 25 – 48 . Dordrecht , , The Netherlands : Elsevier .
- Domeizel , M. , Khalil , A. and Prudent , P. 2004 . UV spectroscopy: A tool for monitoring humification and for proposing an index of the maturity of compost . Bioresource Technol. , 94 ( 2 ) : 77 – 184 . doi: 10.1016/j.biortech.2003.11.026
- Francis , C.A. , Beman , J.M. and Kuypers , M.M. 2007 . New processes and players in the nitrogen cycle: the microbial ecology of anaerobic and archaeal ammonia oxidation . ISME J. , 1 ( 1 ) : 19 – 27 . doi: 10.1038/ismej.2007.8
- Fukumoto , Y. , Suzuki , K. , Osada , T. , Uroda , K. , Hanajima , D. , Yasuda , T. and Haga , K. 2006 . Reduction of nitrous oxide emission from pig manure composting by addition of nitrite-oxidizing bacteria . Environ. Sci. Technol. , 40 ( 21 ) : 6787 – 6791 . doi: 10.1021/es0611801
- Gao , W. , Zheng , G.D. , Gao , D. , Chen , T.B. , Han , X.R. and Zhang , Y.A. 2005 . Transformation of organic matter during thermophilic composting of pig manure . Environ. Sci. , 5 ( 7 ) : 986 – 990 .
- Gu , W.J. , Zhang , F.B. , Xu , P.Z. , Tang , S.H. , Xie , K.Z. , Huang , X. and Huang , Q.Y. 2011 . Effects of sulphur and Thiobacillus thioparus on cow manure aerobic composting . Bioresource Technol. , 102 : 6529 – 6535 . doi: 10.1016/j.biortech.2011.03.049
- Hassen , A. , Jamoussi , F. , Saidi , N. , Mabrouki , Z. and Fakhfakh , E. 2003 . Microbial and copper adsorption by smectitic clay--an experimental study . Environ. Technol. , 24 ( 9 ) : 1117 – 1127 . doi: 10.1080/09593330309385652
- Haug , R.T. 1993 . The Practical Handbook of Compost Engineering , Boca Raton, FL : Lewis .
- He , J.Z. , Shen , J.P. , Zhang , L.M. , Zhu , Y.G. , Heng , Z.Y.M. , Xu , M.G. and Di , H.J. 2007 . Quantitative analyses of the abundance and composition of ammonia-oxidizing bacteria and ammonia-oxidizing archaea of a Chinese upland red soil under long-term fertilization practices . Environ. Microbiol. , 9 : 3152 – 3152 . doi: 10.1111/j.1462-2920.2007.01481.x
- Hogland , W. , Bramryd , T. , Marques , M. and Nimmermark , S. 2003 . Physical, chemical and biological processes for optimizing decentralized composting . Comp. Sci. , 11 ( 4 ) : 330 – 336 .
- Jiminez , E.I., and Garcia , V.P. 1992 . Determination of maturity indexes for city refuse composts. . Agr. Ecosyst. Environ. , 38 : 331 – 343 .
- Keener , H.M. , Ekinci , K.F. and Michel , C. 2005 . Composting process optimization using on/off controls . Comp. Sci. Util. , 13 ( 4 ) : 288 – 299 .
- Kithome , M. , Paul , J.W. and Boke , A. 1999 . Reducing nitrogen losses during simulated composting of poultry manure using absorbents or chemical amendments . J. Environ. Qual. , 28 : 194 – 201 . doi: 10.2134/jeq1999.0047242 5002800010023x
- Kuroda , K. , Hanajima , D. , Fukumoto , Y. , Suzuki , K. , Kawamoto , S. , Shima , J. and Haga , K. 2004 . Isolation of thermophilic ammonium-tolerant bacterium and its application to reduce ammonia emission during composting of animal wastes . Biosci. Biotechnol. Biochem. , 68 : 286 – 290 . doi: 10.1271/bbb.68.286
- Lefcourt , A.M. and Meisinger , J.J. 2001 . Effect of adding alum or zeolite to dairy slurry on ammonia volatilization and chemical composition . J. Dairy Sci. , 84 : 1814 – 1821 . doi: 10.3168/jds.S0022-03020174620-6
- Li , G.X. , Li , Y.C. and Li , Y.F. 2003 . Advance on composting of solid waste and utilization of additives . J. Agro-Environ. Sci. , 22 : 252 – 256 .
- Li , Z.A. , Zou , B. , Cao , Y.S. , Liu , J. and Wen , D.Z. 2002 . Technique of static chamber in determining nitrous oxide emission from land surface . Soil Environ. Sci. , 11 ( 4 ) : 413 – 416 .
- Lin , X.F. , Li , G.X. and Ren , L.M. 2008 . Effect of FeCl3 and Ca(H2PO4)2 as amendments on reducing nitrogen loss during composting . J. Agro-Environ. Sci. , 27 : 1662 – 1666 .
- Mahimairaja , S. , Bolan , N.S. and Hedley , M.J. 1994 . Losses and transformation of nitrogen during composting of poultry manure with different amendments: An inoculation experiment . Bioresource Technol. , 47 : 265 – 273 . doi: 10.1016/0960-85249490190-2
- Martins , O. 1992 . Less of nitrogenous compounds during composting of animal wasters . Bioresource Technol. , 42 : 103 – 111 .
- Paré , T. , Dinel , H. and Schnitzer , M. 1998 . Transformations of carbon and nitrogen during composting of animal manure and shredded paper . Biol. Fertil. Soils , 26 : 173 – 178 . doi: 10.1007/s003740050364
- Raviv , M. , Medina , S. and Karasnovsky , A. 2002 . Conserving nitrogen during composting . Biocycle , 43 : 48 – 51 .
- Ren , L.M. , He , Q. and Li , G.X. 2008 . Effect of Mg(OH)2 and H3PO4 amendments on nitrogen conservation during simulated aerobic composting and its benefit analyses . Trans. CSAE , 24 : 225 – 228 .
- Riffaldi , R. , Levi-Minzi , R. , Pera , A. and de Bertoidi , M. 1996 . Evaluation of compost maturity by means of chemical and microbial analyses . Waste Manage. Res. , 4 : 387 – 396 . doi: 10.1177/0734242X8600400157
- Witter , E. and Kirchmann , H. 1989 . Effects of addition of calcium and magnesium salts on ammonia volatilization during manure decomposition . Plant Soil , 115 : 53 – 58 . doi: 10.1007/BF02220694
- Witter , E. and Lopez-Real , J. 1988 . Nitrogen losses during the composting of sewage sludge . Biol. Wastes , 23 : 279 – 294 . doi: 10.1016/0269-7483(88)90016-X
- Yang , Y.M. , Liu , H.L. and Yang , Z. 2005 . Methods and techniques in the control of nitrogen loss during the composting—A review . Nat. Sci. (Beijing Normal Univ.) , 41 : 213 – 216 .
- Yang , H. , Ning , H.L. and Pei , L. 2011 . Properties study on ammonia nitrogen adsorption of attapulgite . Chin. J. Environ. Eng. , 5 ( 2 ) : 343 – 346 .
- Zhang , F.B. , Gu , W.J. , Xu , P.Z. , Tang , S.H. , Xie , K.Z. , Huang , X. and Huang , Q.Y. 2011 . Effects of alkyl polyglycoside (APG) on composting of agricultural wastes . Waste Manage. , 31 : 1333 – 1338 . doi: 10.1016/j.wasman.2011.02.002
- Zucconi , F. 1987 . “ Compost specifications for the production and characterization of compost from municipal solid waste ” . In Compost: Production, Quality and Use , Edited by: De Bartholdi , M. , De Bartholdi , M. , Ferranti , P. , Hermite , P.L. and Zucconi , F. 30 – 50 . Essex : Elsevier Applied Science .