Abstract
Microwave (MW) is applied to enhance perchloroethylene (PCE) or pentachlorophenol (PCP) removal using zero-valent iron (ZVI; Fe0) as the dielectric medium. ZVI has a much higher dielectric loss factor (39.5) than other media; it is capable of absorbing MW radiation rapidly to speed up the release of electrons, leading to rises of the ZVI particle surface temperature. If the MW power is continued, excessive electricity will accumulated inside ZVI particles, resulting in sparks. The results show that during the initial 5 sec (700 W), the linear aliphatic PCE has a faster decomposing rate than the ringed PCP (82.0% vs. 4.8%) because less energy is required for decomposing the linear-chlorine bond (90 kcal mol−1) than ring-chlorine bonds (95 kcal mol−1). Later, the removal rate for either PCE or PCP remains the same when the exposure time is between 5 and 60 sec. Without MW irradiation, linear PCE molecules have larger surface area to contact ZVI, and hence they have better removal efficiencies than PCP molecules. Using Fe0 as a microwave dielectric medium to treat PCE or PCP is a new and worthwhile treatment technology; it is environmentally friendly, and its use will eliminate the secondary pollution.
Nanoscale iron particles are characterized by high surface-area-to-volume ratios, high specific surface area, and high surface reactivity. With a much higher dielectric loss factor, it is capable of absorbing MW radiation rapidly to speed up the release of electrons, leading to rise in temperature. The time needed to achieve a satisfactory treatment is also reduced, leading to significant saving of energy consumption to make this method cost-effective and also environmentally friendly for the industry to pursuit sustainable development.
Introduction
Perchloroethylene (PCE), which has been extensively used in commercial processes such as machine producing, metal degreasing, and dry cleaning, is extremely transportable in the environment to cause serious problems in surface water bodies and groundwater (CitationFukami et al., 2004; CitationHincapié et al., 2005). Conventional physicochemical methods to remove PCE contamination use expensive chemicals or consume excessive energy, and hence, these methods are not practical for field applications. Biological processes may be effective; the conditions must be well controlled to enhance adequate microbial growth (CitationLin et al., 2005). Advanced oxidation processes, such as titanium oxide/ultraviolet (TiO2/UV), photo-Fenton, and ozone, are effective and inexpensive but may produce toxic by-products (CitationTorrades et al., 2004; CitationMalato et al., 2002; CitationAguedach et al., 2005).
Zero-valent iron (ZVI or Fe0) particles have been successfully applied to remove halide-containing organic substances, for example, chlorobenzene, from wastewater or groundwater (CitationLee and Jou, 2011, Citation2012), The metal is inexpensive and nontoxic (CitationLin et al., 2004; CitationLin and Lo, 2005) but may have several disadvantages: (1) after a short period of reaction time, Fe0 particles will form a thin film of oxides on the surface to lower the activity of the iron; (2) the high surface activity of Fe0 particles is difficult to maintain; and (3) Fe0 particles from various sources may have obvious different capabilities in oxidizing halides (CitationLin and Lo, 2005; CitationZhang et al., 1998). These disadvantages may be alleviated by prewashing the ZVI particles with acids to remove the oxidized layer that may accumulate on the surface (CitationClark et al., 2003; CitationLien et al., 2001).
Microwave (MW) heating is made possible by exposing an appropriate dielectric material to MW irradiation to convert the absorbed MW energy into heat. The average energy absorbed per unit mass of the substance can be expressed as
EquationEquation 1 shows that plays an important role in the MW energy absorption equation. The temperature rise of the medium absorbing MW energy can be expressed by the following equation (CitationClark et al., 2000; CitationZhang et al., 2006):
EquationEquation 3 is derived by combining Equationeqs 1 and Equation2:
Using an appropriate medium to generate heat from MW energy is of great importance to develop the MW irradiation into an effective thermal waste treatment method. For example, the MW radiation may also be combined with the adsorption capacity of granular activated carbon to treat pentachlorophenol (PCP) and phenol (Jou and Wu, 2006; CitationTai and Jou, 1999), improve the efficiency of TiO2 photocatalyst (CitationJou et al., 2008b; CitationLiao et al., 2010) with demonstrated energy savings efficiency improvement (CitationLiu et al., 2004), and treat heavy-oil-contaminated sand (CitationChang et al., 2011). In this study, the characteristics of MW heating such as low reaction time to achieve rapid and selective heating are combined with the superb properties of ZVI particles as MW dielectric medium, including high specific surface area, surface reactivity, and permittivity loss factor, to decompose refractory linear aliphatic PCE and ringed PCP. The influence of reaction time and MW power on ZVI temperature, the average MW absorbed, and the comparative efficiencies of removing PCE and PCP are studied.
Materials and Methods
Preparation of ZVI
The ZVI particles used in this research were prepared by mixing 0.045 M FeCl3·6H2O (97%; Showa, Alfa Aesar, USA) and 0.25 M NaBH4 (98%; Lancaster, Showa Chemicals, Japan). The following chemical equation delineates the production of Fe0 from Fe3+ (CitationChen et al., 2001):
The resulting Fe0 particles have diameters between 60 and 80 nm with 33.36 m2 g −1 specific surface area as determined using the Brunauer-Emmett-Teller (BET) method (SA-300; Beckman, USA).
Experimental setup
shows the schematic diagram of the laboratory setup for conducting the microwave-energy-enhanced degradation of PCE or PCP. A household microwave oven (frequency 2.45 GHz; Sampo, Taiwan) with maximum power output of 750 W was utilized for generating the microwave energy. It was slightly modified by installing a programmable PID (proportional integral derivative) controller. The MW energy was controlled by programming the PID (proportional-integral-derivative) controller to adjust the PID feedback signal for maintaining a constant preselected MW energy level. The 30-mL column bottle reactor that was made of low-energy-loss and heat-resistant (up to 700°C) boron-silica amber glass with Teflon-lined screw cap was used to hold the sample in the decomposition studies. After being filled with samples, the column bottle reactor was covered with 1 g fresh granular activated carbon (GAC) at the top in order to capture all tail gas during the decomposition treatment. For the MW-induced decomposition study, the column bottle reactor was placed in the microwave oven to carry out the experiment.
Batch tests
The test sample was prepared by dissolving pure 40.58 mg PCE (99.98%; Tedia Co., Tedia, USA), or 49.45 mg solid PCP (90%; Lancaster) in 25-mL analytical acetone solution in the column reactor. ZVI was then added to the reactor containing the prepared sample to carry out the decomposition studies. The top portion of the vertical reactor was filled with fresh activated carbon for absorbing any PCE or PCP that may escape with the tail gas during the treatment.
Three sets of reactors each containing 0.1, 0.3, and 0.5 g ZVI, respectively, were subject to MW treatment. The decomposition studies were repeated by exposing the samples to an array of 350, 490, and 700 W MW power for various durations, that is, 5, 10, 20, 30, 40, 50 and 60 sec. On the other hand, after the MW treatment, the GAC was recovered. It was added with 25 mL acetone and the mixture was shaken in an ultrasonic wave shaker for 5 min; the acetone extract was used to determine Cl − concentration and pH value.
Analysis methods
The ZVI real permeability and loss factor permeability measured using an impedance analyzer (Agilent, 4291B; USA) operated at 1.8 GHz and 25°C are 19.92 and 39.43 F m −1, respectively. Qualitative analyses of PCE and other related chemicals were conducted with an HP 6890 gas chromatography (GC) coupled with an HP 5973 mass selective detector (MSD) (xx, xx). An HP capillary column (HP-5MS; 30 m × 0.25 mm × 0.25 μm) was used for identifying intermediates and degradation products, with the carrier gas (He) flow rate kept constant at 1.5 mL min −1. For analyzing PCE, the oven temperature was programmed to vary from 35°C (maintain for 1 min) to 130°C at a ramp rate of 5°C min −1, whereas for PCP analyses, the oven temperature was programmed from 70 to 260°C at a ramp rate of 30°C min −1 and held at 260°C for 5 min. The real permittivity and permittivity loss factors of ZVI, analyzed using an impedance analyzer (Agilent, 4291B) operated at 1.8 GHz and 25°C are 20.6 and 39.5 F m−1, respectively.
The material structure of nanoscale ZVI particles was analyzed using X-ray diffraction (Philips XRD 3100 diffractometer; xx, xx) at 45 kV and 30 mA. The X-ray with a wavelength of 1.54060 Å was generated using Cu Kα radiation and a graphite monochromator. Samples were placed in a glass holder and were scanned from 10 to 80° at 2.0° min−1. This scanning range covered all major species of iron and iron oxides. X-ray diffraction (XRD) was used to investigate the material structure synthesis of nanoscale iron particles. The XRD pattern shown in reveals main peaks of iron corresponding to the diffraction angles (2θ) of 44.9°. Images produced by using a scanning electron microscope (SEM) (Hitachi, S-2500; Japan) were used to evaluate the consumption of surface material, and infrared (IR) (Raytek, Raynger 3i) was used to measure temperature variations of the medium during the reaction process.
Results and Discussion
Decomposition of PCE and PCP treated with zero-valent iron only
Zero-valent iron (ZVI or Fe0) serves as an electron donor (reducing agent) to reduce organic substances (CitationHou et al., 2007); the rate can be approximated by a first-order reaction (CitationLin et al., 2004; CitationLin and Lo, 2005; CitationJanda et al., 2004; CitationLien and Zhang, 2005):
Because the chemical reduction occurs at the ZVI particle surface, k can be replaced by k sa and ρa as (CitationLin et al., 2004; CitationLin and Lo, 2005; CitationLiu et al., 2004):
shows that after 120 min, 0.5 g Fe0 particles will remove 3.0% PCP at a reaction rate (k) of 3.0 × 10−4 min−1 and a surface-area-normalized reaction rate constant (k sa) of 4.5 × 10−7 L min −1 m−2, whereas 5.3% PCE is removed at 5.0 × 10−4 min−1 (k) and 7.5 × 10−7 L min −1 m−2 (k sa). The linear PCE molecule has a larger contact surface with Fe0 particles than the ringed PCP molecule, and hence a better removal efficiency than the latter.
Influence of MW induction on ZVI temperature and absorbed power
Both the dielectric property of a medium and the MW power level affect the temperature of the medium when exposed to MW irradiation. If the MW output power is maintained at 700 W for 10 sec, the ZVI particle surface temperature rises form 28 to 490°C, causing Fe0 to be oxidized into Fe+2, and the release of more electrons. The rapid movement of iron electrons produces more friction heat, and hence the rate of temperature rise is proportional to the number of electrons released. The oxidation of each Fe0 atom releases 2 electrons but oxidation of ferrous atom (Fe+2) releases only 1 electron. After all Fe0 atoms are converted to Fe+2, fewer electrons are released, causing the rate of heat increase to decrease. Afterward, the rate of temperature rise becomes moderate and the temperature reaches 540°C at 60 sec.
When the MW output power is maintained at 700 W for 10 sec, the calculated MW energy absorbed by ZVI is 77.8 W cm −3 based on Equationeq 3. If the exposure time is extended to 60 sec, the calculated average absorbed energy increases to 86.2 W cm −3.
Sparks are observed when the exposure time exceeds 30 sec because the Fe+2 formed from ZVI has a lower capacity than ZVI in absorbing the MW output power. If the same level of MW power is continued, excessive electricity will accumulate inside ZVI particles, resulting in sparks. Changes of ZVI surface structure before and after the MW treatment can be shown using SEM photographs. shows the protuberating rods on the original iron particles prior to MW irradiation. The MW irradiation causes Fe0 atom to change to Fe+2 by releasing 2 electrons; the oxidized ions (i.e., Fe+2 and Fe+3) cover the ZVI particle surface and the thin film of oxides. shows the disappearance of these protuberating rods and a smoother surface for ZVI particles after MW irradiation. On the other hand, comparisons of the X-ray diffraction analyses for ZVI particles before and after MW treatment can be seen in and b. The peak corresponding to amorphous-phase iron exists in the large-scale wave range. In , ZVI particles without MW irradiation have obvious peak at 2θ value of 44.9°, indicating the existence of ZVI. In , for ZVI after MW irradiation, the peak at 2θ value of 44.9° obviously decreases with the accompanied appearance of weak peaks at 35.6°, 43.4°, and 63.2° so that the existence of oxidized iron is confirmed.
Influence of MW energy levels on PCE and PCP removal
Because of its nonlocality, the valent electron of a metal moves in a waveform. When the metal is placed in the MW electrical field, the electron will move toward the anode and gain more energy to accelerate the motion. In contrast, electrons may move toward the cathode to reduce their energy and speed (CitationVlack, 1989). Concurrent with the conversion of high-frequency electrical field, the metal placed in the electrical field will absorb MW energy to cause differences in electropotential attraction, leading to more rapid spinning of the electron to result in more heat generation due to friction.
shows that using 0.5 g ZVI as dielectric medium, the efficiency of PCE or PCP removal increases with higher MW output power for the same reaction time. If the MW output power is raised from 350 to 700 W (20-sec exposure times), the PCE removal efficiency increases from 26.0% to 98.0%, reaction rate (k) increases from 0.04 to 0.14 sec−1, and surface-area-normalized reaction rate constant (k sa) increases from 5.9 × 10−5 to 2.2 × 10−4 L sec −1 m−2; whereas the PCP removal efficiency increases from 13.3% to 91.3%, reaction rate (k) increases from 3.7 × 10−2 to 7.8 × 10−3 sec−1, and surface-area-normalized reaction rate constant (k sa) increases from 5.6 × 10−5 to 1.2 × 10−5 L sec −1 m−2. The energy needed to crack the chlorine bond in PCE molecule is 90 kcal mol −1, which is less than the 95 kcal mol −1 for cracking the chlorine bond in PCP molecule. Hence, during the initial treatment period (exposure time from 5 to 20 sec), PCE is removed at a faster rate than PCP. Later, the difference between the removal rates becomes smaller.
Figure 5. PCE and PCP removal efficiencies versus exposure reaction time with 0.5 g ZVI particles subject to 700 and 350 W MW irradiation.
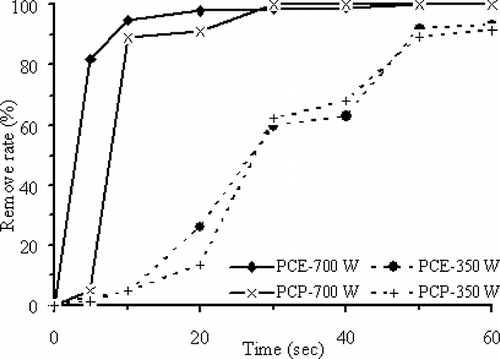
ZVI particles have rather high activity; they may self-oxidize to release heat at room temperature. When exposed to MW irradiation, the oxidation rate will be accelerated by the absorbed MW energy to enhance the decomposition of organic substances such as PCE and PCP in addition to raising temperature. The PCE or PCP removal efficiencies in the presence of ZVI particles as dielectric medium with and without MW irradiation ( and , respectively) are compared. The results show that PCE and PCP subject to the MW treatment (i.e., 700 W MW irradiation for 20 sec) have higher decomposition efficiencies (98.0% for PCE and 91.3% for PCP) than PEC and PCP without MW treatment for 120 min (5.3% for PCE and 3.0% for PCP).
Influence of ZVI dosages on the treatment efficiency
Higher total surface areas will enable the dielectric substance to absorb more MW energy. demonstrates that with 700 W MW output power for 10 sec, 41.0% PCE is removed with 0.1 g ZVI particles. When the ZVI dosage is increased to 0.3 and 0.5 g, the PCE removal efficiency is raised to 88.0% and 95.0%, respectively. Similarly, when the ZVI dosage is increased to 0.1, 0.3, and 0.5 g, the PCP removal efficiency is raised to 47.0%, 53.0%, and 89.1%, respectively. In the MW electrical field, increase of ZVI dosages increases the total ZVI particle surface area as well as the contact between ZVI particles and PCE or PCP in addition to more surface to absorb MW energy. On the other hand, the accelerated absorption of MW energy by ZVI particles increases the oxidation of Fe0 atom to release electrons, and enhances the PCE or PCP removal rate. This results in more heat for better removal of PCE or PCP. Additionally, PCE molecules have pointed protuberances on the surface; hence, they have larger surface area that can absorb more MW than PCP molecules. This may account for the observed better removal efficiency for PCE than PCP under similar conditions.
Analyses of the tail gas from the reactor
Qualitative analyses of the tail gas from the reactor were carried out by injecting 0.2 mL of the reactor tail gas into the GC/MSD and the acetone extraction to determine Cl − concentration and pH value. Results of GC analyses performed on PCE show that at an exposure time of 10 sec, the tail gas is found to contain CO2 and trace CO; whereas concentration of Cl− in the solvent extraction increases from 1.17 to 12.3 mg L−1, the pH drops from 7.6 to 3.6. Because ZVI has a higher permittivity loss factor of 39.5, it absorbs the MW energy when exposed to the MW electrical field. With increasing exposure time, the release of electrons from ZVI speeds up with accompanying increase of ZVI surface temperature. The heat produced by MW-enhanced Fe0 oxidation reactions will raise the Fe0 temperature high enough (520–540°C) to complete the thermo-cracking of PCE molecules. Hence, the C=C double bond and C–Cl bond Mare cracked to form CO2 and HCl. According to the results of previously studies, the MW-induced decomposition of PCE and PCP molecules can be expressed by:
Conclusion
In this study, MW energy is used to induce the Fe0 oxidation rate to achieve high temperature for decomposing PCE and PCP. During the initial treatment period (700 MW, exposure time 5 sec), PCE has a faster decomposing rate than PCP (82.0% vs. 4.8%). The linear-chlorine PCE bond (90 kcal mol −1) requires less energy than ring-chlorine PCP bonds (95 kcal mol −1) to be decomposed during the initial reaction period. For longer MW exposure time (i.e., 30 sec), the removal rates for PCE and PCP remain the same.
Additionally, the linear PCE molecules have more contacts with ZVI particles than the ringed PCP molecules. Therefore, in the absence of MW energy, ZVI particles have better removal efficiency for PCE than PCP. Under the optimal operating conditions of applying 700 W MW energy for 20 sec, the removal efficiency is not affected by the molecular structures of PCE and PCP, and high removal efficiencies can be achieved within a short period of treatment time. Therefore, using ZVI as a MW dielectric medium to treat PCE and PCP is a new and worthwhile waste treatment technology.
References
- Aguedach , A. , Brosillon , S. , Morvan , J. and Lhadi , E.K. 2005 . Photocatalytic degradation of azo-dyes reactive black 5 and reactive yellow 145 in water over a newly deposited titanium dioxide . Appl. Catal. B Environ , 57 : 55 – 62 . doi: 10.1016/j.apcatb.2004.10.009
- Chang , H.J. , Jou , C.J.G. and Lee , C.L. 2011 . Treat heavy oil contaminated sand by microwave energy . Environ. Eng. Sci , 28 : 863 – 872 . doi: 10.1089/ees.2010.0328
- Chen , J.L. , Al-Abed , S.R. , Ryan , J.A. and Li , Z. 2001 . Effects of pH on dechlorination of trichloroethylene by zero-valent iron . J. Hazard. Mater , 83 : 243 – 254 . doi: 10.1016/S0304-3894(01)00193-5
- Clark , C.J. II , Rao , P.S.C. and Annable , M.D. 2003 . Degradation of perchloroethylene in cosolvent solutions by zero-valent iron . J. Hazard. Mater , 96 : 65 – 78 . doi: 10.1016/S0304-3894(02)00162-0
- Clark , D.E. , Folz , D.C. and West , J.K. 2000 . Processing materials with microwave energy . Mater. Sci. Eng , 287 : 153 – 158 . doi: 10.1016/S0921-5093(00)00768-1
- Fukami , N. , Yosida , M. , Lee , B.D. , Taku , K. and Hosomi , M. 2004 . Photocatalytic degradation of gaseous perchloroethylene: Products and pathway . Chemosphere , 42 : 345 – 350 . doi: 10.1016/S0045-6535(00)00140-5
- Hincapié , M. , Maldonado , M.I. , Oller , I. , Gernjak , W. , Sánchez-Pérez , J.A. , Ballesteros , M.M. and Malato , S. 2005 . Solar photocatalytic degradation and detoxification of EU priority substances . Catal. Today , 101 : 203 – 210 . doi: 10.1016/j.cattod.2005.03.004
- Hou , M. , Li , F. , Liu , X. , Wang , X. and Wan , H. 2007 . The effect of substituent groups on the reductive degradation of azo dyes by zerovalent iron . J. Hazard. Mater , 145 : 305 – 314 . doi: 10.1016/j.jhazmat.2006.11.019
- Janda , V. , Vasek , P. , Bizova , J. and Belohlav , Z. 2004 . Kinetic models for volatile chlorinated hydrocarbons removal by zero-valent iron . Chemosphere , 54 : 917 – 925 . doi: 10.1016/j.chemosphere.2003.08.033
- Jou , C.J., and Wu , C.R. 2008a . Granular activated carbon coupled with microwave energy for treating pentachlorophenol-containing wastewater . Environ. Prog , 27 : 111 – 116 . doi: 10.1002/ep.10245
- Jou , C.J.G. , Lee , C.L. , Tsai , C.H. and Wang , H.P. 2008b . Microwave energy enhanced photocatalyses with titanium dioxide (TiO2) for Degradation of trichloroethylene . Environ. Eng. Sci , 25 : 975 – 980 . doi: 10.1089/ees.2007.0155
- Lee , C.L. and Jou , C.J.G. 2011 . Reduced degradation of chlorobenzene in co-solvent solution using nano-scale zero-valent iron with microwave irradiation . Environ. Eng. Sci , 28 : 191 – 195 . doi: 10.1089/ees.2010.0152
- Lee , C.L. and Jou , C.J.G. 2012 . Degradation of chlorobenzene with microwave-aided zero-valent iron particles . Environ. Eng. Sci , 29 : 432 – 435 . doi: 10.1089/ees.2010.0329
- Liao , W. , Zheng , T. , Wang , P. , Tu , S. and Pan , W. 2010 . Microwave-assisted photocatalytic degradation of dimethyl phthalate over a novel ZrOx catalyst . Environ. Eng. Sci , 27 : 1001 – 1007 . doi: 10.1089/ees.2010.0159
- Lien , H.L. and Zhang , W.X. 2005 . Hydrodechlorination of chlorinated ethanes by nanoscale Pd/Fe bimetallic particles . J. Environ. Eng , 131 : 4 – 10 . doi: 10.1061/(ASCE)0733-9372(2005)131:1(4)
- Lin , C.J. and Lo , S.L. 2005 . Effects of iron surface pretreatment on sorption and reduction kinetics of trichloroethylene in a closed batch system . Water Res , 39 : 1037 – 1046 . doi: 10.1016/j.watres.2004.06.035
- Lin , C.J. , Lo , S.L. and Liou , Y.H. 2004 . Dechlorination of trichloroethylene in aqueous solution by noble metal-modified iron . J. Hazard. Mater , 116 : 219 – 228 . doi: 10.1016/j.jhazmat.2004.09.005
- Lin , C.J. , Lo , S.L and Liou , Y.H. 2005 . Degradation of aqueous carbon tetrachloride by nanoscale zerovalent copper on a cation resin . Chemosphere , 59 : 1299 – 1307 . doi: 10.1016/j.chemosphere.2004.11.064
- Lien , H.L. and Zhang , W.X. 2001 . Nanoscale iron particles for complete reduction of chlorinated ethenes . Colloid. Surface , 191 : 97 – 105 . doi: 10.1016/S0927-7757(01)00767-1
- Liu , X. , Quan , X. , Bo , L. , Chen , S. and Zhao , Y. 2004 . Simultaneous pentachlorophenol decomposition and granular activated carbon regeneration assisted by microwave irradiation . Carbon , 42 : 415 – 422 . doi: 10.1016/j.carbon.2003.12.032
- Malato , S. , Blanco , J. , Vidal , A. and Richter , C. 2002 . Photocatalysis with solar energy at a pilot-plant scale: An overview . Appl. Catal. B Environ , 37 : 1 – 15 . doi: 10.1016/S0926-3373(01)00315-0
- Tai , H.S. and Jou , C.J.G. 1999 . Application of granular activated carbon packed-bed reactor in microwave radiation field to treat phenol . Chemosphere , 38 : 267 – 275 . doi: 10.1016/S0045-6535(98)00432-9
- Torrades , F. , García-Montańo , J. , García-Hortal , J.A. , Domènech , X. and Peral , J. 2004 . Decolorization and mineralization of commercial reactive dyes under solar light assisted photo-fenton conditions . Solar Energy , 77 : 573 – 581 . doi: 10.1016/j.solener.2004.05.004
- Vlack , L.H.V. 1989 . Element of Materials Science and Engineering , Boston , MA : Addison-Wesley .
- Zhang , W.X. , Wang , C.B. and Lien , H.L. 1998 . Treatment of chlorinated organic contaminants with nanoscale bimetallic particles . Catal. Today , 40 : 387 – 395 . doi: 10.1016/S0920-5861(98)00067-4
- Zhang , X. and Hayward , D.O. 2006 . Applications of microwave dielectric heating in environment-related heterogeneous gas-phase catalytic systems . Inorg. Chim. Acta , 359 : 3421 – 3433 . doi: 10.1016/j.ica.2006.01.037