Abstract
The U.S. Environmental Protection Agency (EPA) has proposed a new secondary standard based on visibility in urban areas. The proposed standard will be based on light extinction, calculated from 24-hr averaged measurements. It would be desirable to base the standard on a shorter averaging time to better represent human perception of visibility. This could be accomplished by either an estimation of extinction from semicontinuous particulate matter (PM) data or direct measurement of scattering and absorption. To this end we have compared 1-hr measurements of fine plus coarse particulate scattering using a nephelometer, along with an estimate of absorption from aethalometer measurements. The study took place in Lindon, UT, during February and March 2012. The nephelometer measurements were corrected for coarse particle scattering and compared to the Filter Dynamic Measurement System (FDMS) tapered element oscillating microbalance monitor (TEOM) PM2.5 measurements. The two measurements agreed with a mass scattering coefficient of 3.3 ± 0.3 m2/g at relative humidity below 80%. However, at higher humidity, the nephelometer gave higher scattering results due to water absorbed by ammonium nitrate and ammonium sulfate in the particles. This particle-associated water is not measured by the FDMS TEOM. The FDMS TEOM data could be corrected for this difference using appropriate IMPROVE protocols if the particle composition is known. However, a better approach may be to use a particle measurement system that allows for semicontinuous measurements but also measures particle bound water. Data are presented from a 2003 study in Rubidoux, CA, showing how this could be accomplished using a Grimm model 1100 aerosol spectrometer or comparable instrument.
Visibility is currently based on 24-hr averaged PM mass and composition. A metric that captures diurnal changes would better represent human perception. Furthermore, if the PM measurement included aerosol bound water, this would negate the need to know particulate composition and relative humidity (RH), which is currently used to estimate visibility. Methods are outlined that could accomplish both of these objectives based on use of a PM monitor that includes aerosol-bound water. It is recommended that these techniques, coupled with appropriate measurements of light scattering and absorption by aerosols, be evaluated for potential use in the visibility based secondary standard.
Introduction
In June 2012 the U.S. Environmental Protection Agency (EPA) proposed to revise the Particulate Matter National Ambient Air Quality Standard (PM NAAQS) (EPA, 2012). Included in this proposal is a new secondary PM standard, which addresses the effects of visibility on the public. Currently visibility is regulated in Class I federal areas such as national parks and wilderness areas by the Regional Haze Program. Under this program, visibility is characterized and monitored using a visibility index, which is calculated in part from speciated PM2.5 mass concentrations. Similar to the existing Regional Haze Program, the proposed secondary PM NAAQS uses speciated PM2.5 mass concentrations to calculate a visibility index for urban areas. All concentrations are averaged over 24 hr and combined to calculate a light extinction coefficient using the IMPROVE algorithm. The five PM2.5 species that are used in the algorithm are sulfate, nitrate, organic carbonaceous mass, elemental carbon, and fine soil/crustal material, along with relative humidity. Sulfate, nitrate, and relative humidity are combined in the moist light extinction efficiency (MLEE) term, which accounts for the changes in light extinction at high relative humidity due to an increase in water bound to particles. The dry light extinction efficiency (DLEE) term accounts for light extinction at low relative humidity. The basic format of the algorithm used in the proposed standard is represented by the following (CitationPitchford, 2010; EPA, 2011):
The speciated data for sulfate and nitrate are often 24-hr samples, and RH levels are recorded hourly. It is important to note that because 24-hr averaged concentrations are used, diurnal cycles are not properly accounted for.
In preparation for this proposed standard, the EPA referred to many studies on human perception of visibility. (EPA, 2011) These studies verify that we perceive visibility on a short time scale as reported in Class I areas (CitationHyslop, 2009; EPA, 2011). Therefore, our visibility perception is better represented by hourly averaged data than by the proposed 24-hr standard. The EPA noted that hourly averaged measurements would better represent human perception as a light extinction indicator; however, the EPA felt that short-term variability of current instruments (for 1-hr averaged data) may cause data quality issues that are minimized by averaging over 4-24 hr (EPA, 2011). As a result, a 24-hr averaging time was chosen. These limitations prevent the proposed standard from reflecting the current understanding of human visibility perception.
The EPA also considered using directly measured PM2.5 light extinction as a visibility indicator, as opposed to the proposed calculated mass-based indicator. The EPA discussed numerous advantages of directly measuring light extinction, including the fact that it more directly relates to human perception of visibility. However, the EPA concluded that the development of a federal reference method (FRM) for direct light extinction measurements would take several years and has not yet begun development for such a method. Therefore, the EPA proposed to rely upon the existing network of instrumentation for mass-based concentrations to calculate PM2.5 visibility index values (EPA, 2011; EPA, 2012).
Before the next review of this proposed standard, it is necessary to conduct more scientific studies in urban areas to evaluate the adequacy of the proposed secondary PM NAAQS and the proposed FRM. Included is the need to evaluate a standard based upon hourly averaged concentrations and to further evaluate the instrumentation used. This study is a beginning toward this objective.
Experimental
The results of two different field studies are used in this paper.
Lindon, Utah, 2012 field study
A field study was conducted in Lindon, UT, at a Brigham Young University (BYU) air sampling site, which is located adjacent to the State of Utah PM monitoring site (40.338841 N, 111.7141 W). It was conducted from February to March 2012. During this time the daily high and low temperatures ranged between a low of –5°C to a high of 16°C in February and a low of –4°C to 21°C in March. Lindon, UT, located in Utah Valley, often suffers from wintertime inversions. A number of inversions occurred during the sampling campaign, including on February 4–11 and March 8–12. The onset of an inversion is evidenced by sharp increases in PM2.5 concentrations. All inversions were relatively weak because there was no persistent snow cover on the ground during the study. Hourly measurements of PM2.5 mass were made with an Filter Dynamic Measurement System tapered element oscillating microbalance monitor (FDMS TEOM) and a conventional TEOM heated to 30°C. PM10 mass was also measured with a conventional TEOM at 30°C. Coarse particulate mass was estimated as the difference between the two conventional TEOM measurements. PM2.5 carbonaceous material was measured with a dual oven (DO) semicontinuous OC–EC field analyzer (CitationGrover et al., 2009) from Sunset Laboratory, giving nonvolatile carbonaceous material (CM), semivolatile CM, and black carbon (CitationGrover et al., 2008). Black carbon and UV-absorbing carbon were measured with a McGee scientific aethalometer, model AE-22. Light scattering was measured with a Radiance Research integrating nephelometer, model M903. Concentrations of fine particulate sulfate and nitrate were estimated based on the FDMS TEOM, dual oven, and aethalometer data (CitationGrover et al., 2008). Hourly averaged data were obtained continuously with some gaps/exceptions as indicated in Calibration of the instruments was performed according to manufacturers’ specifications.
Rubidoux, California, 2003 field study
A study was conducted during July 2003 at the SCAQMD sampling site in Rubidoux, CA, as has been previously reported (CitationObeidi et al., 2002). Instruments were compared for semicontinuous measurement of fine particulate mass, including the semivolatile fraction. Both FDMS and differential TEOM monitors were used, and results were compared with the following: 1-hr RAMS, real-time ambient mass sampler (CitationObeidi et al., 2002) measurements; 1-hr averaged R&P particulate nitrate measurements; 1-hr averaged nonvolatile and semivolatile CM measurements using a DO OC-EC analyzer; and 3-hr integrated results from the PC-BOSS, Particle Concentrator—BYU Organic Sampling System (CitationDing et al., 2002) sampler results. During this study, fine particulate mass, including water content (CitationGrimm and Eatough, 2009), was measured using a Grimm model 1.107 monitor; however, the Grimm results were not included in the previous publication. The interpretation of these results is included in this paper.
Results and Discussion
Lindon Study: Correlation of FDMS and nephelometer measurements and the effect of RH
In this study, correlations between the FDMS TEOM and the nephelometer measurements indicate that the FDMS TEOM PM2.5 measurements will not adequately characterize light scattering at higher relative humidity (RH; >80%) when water is more readily absorbed by the aerosol. This conclusion is reached using the hourly averaged data obtained for PM2.5 mass and total light scattering using an FDMS TEOM and nephelometer (corrected for coarse particle scattering). These data, along with relative humidity, are shown in Time periods with very high Bp,scat values due to the presence of fog are marked with dashed lines.
The correlation between PM2.5 and corrected coarse particle scattering Bp,scat (assuming a mass scattering efficiency of 0.5 m2/g for coarse particles) is shown in This is limited to the time periods of February 4–7, February 10–11, February 15–16, and March 7–11, when nephelometer data were available and daily maximum hourly average PM2.5 concentrations exceeded 15 µg/m3. Time periods in which RH exceeded 80% (deliquescent point of ammonium sulfate) are indicated by solid black data points, and the time periods when the RH was between 65 and 80% (between the deliquescent points for ammonium nitrate and ammonium sulfate) are indicated by solid gray data points (excluding time periods with fog present). The deviation from linearity of the solid data points is due to the effect of particle-associated water, which is not measured by the FDMS TEOM. As expected, the deviations are greater for RH > 80% than for RH between 65 and 80%. At the lower range only ammonium nitrate will absorb water. Above 80% RH both ammonium sulfate and ammonium nitrate will absorb water and the rate of mass increase with increasing RH is greater. The relationship between the FDMS results and the effect of increasing RH can best be understood by considering that the FDMS TEOM first uses a cyclone cutoff, and then passes the sample through a Nafion dryer to remove the water (both gas-phase and particle-bound) (Meyer et al., 2002). Particle-bound water significantly increases above 80% humidity since this is the deliquescence point of (NH4)2SO4 and above the deliquescence point of NH4NO3(62%). (CitationFinlayson-Pitts and Pitts, 2000) Thus, the particles increase in mass above 65% RH and sharply increase in mass above 80% RH, and cause more light scattering, which is not indicated by the mass measurement since this water is not measured by the FDMS TEOM. This introduces a negative bias to the DLEE, and inclusion of these mass measurements (RH > 80%) would underestimate the light extinction coefficient. An appropriate IMPROVE algorithm could account for this, and the U.S. EPA has encouraged efforts to better calculate light extinction using the instruments currently deployed in the field (such as the FDMS TEOM) (EPA, 2011). However, in order to reliably calculate hourly averaged extinction, hourly concentrations of NH4NO3 and (NH4)2SO4 are necessary, and these concentrations are often not available. The difference between the measured mass and the true particle mass is even larger for instruments that do not reliable measure aerosol semivolatile mater (e.g., nitrate and semivolatile organic material). This would include a conventional TEOM and other filter-based PM2.5 FRM instruments.
Figure 2. Variation of nephelometer response (corrected for coarse particle scattering) with FDMS PM2.5 from the Lindon 2012 study. Linear regression of open data gives a mass scattering efficiency of 3.3 m2/g. The black closed data points are associated with ambient RH > 80%. The gray closed data points are associated with ambient RH from 65 to 80%. The increased scattering associated with this data can be attributed to particle-bound water that is not measured by the FDMS TEOM.
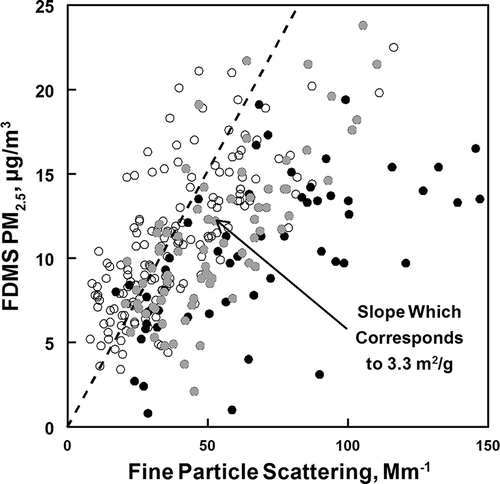
During the time periods of low humidity (less than 65%) when aerosol water content should be small or negligible (open data points in ), the linear relationship between measured PM2.5 mass and light scattering should give the mass scattering efficiency for the dry fine aerosol. Linear regression analysis of the open data points in gives a predicted average fine particulate mass scattering efficiency of 3.3 ± 0.3 m2/g (N = 149, r2 = 0.53) with an intercept of 1 ± 14 Mm−1. This value compares favorably to the average dry mass scattering efficiency obtained in a wintertime study in the Salt Lake Valley of 3.0 m2/g (CitationLong et al., 2005) and a value of 3.6 m2/g from the IMPROVE protocols. (Pitchford et al., 2007) The IMPROVE protocol value is calculated using the data in , which are the estimated average composition of fine particulate aerosols during February 9–10. This composition is similar to the composition seen in previous studies at Lindon (CitationHansen et al., 2010).
Figure 3. Average composition of FDMS measured PM2.5 during February 9–10 in the Lindon study. Indicated species are nonvolatile organic material (NVOM), semivolatile organic material (SVOM, estimated as the difference between the FDMS TEOM measurement and the other measured constituents), sulfate and nitrate (present as the ammonium salts), and black carbon (BC). Concentrations are µg/m3.
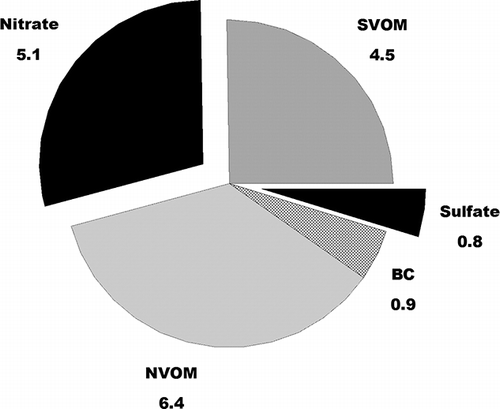
Further comparison of light scattering with other data obtained in Lindon reveals events in which correlation between primary emissions, formation of secondary aerosol, and light scattering can be examined. These relationships are best seen during the episode of high PM2.5 concentrations, between March 8 to 12, as shown in and indicates a weak inversion giving rise to increased pollution. Since nighttime RH did not exceed 80%, the impact of water uptake by the aerosol is negligible. A 2007 winter study in Lindon included a source apportion analysis (Hansen, Woolwine et al., 2010), which indicated that elevated daytime BC concentrations were associated with emission from mobile sources, and high BC concentrations in the late evening were due to emission from the home combustion of wood. Both daytime and nighttime formation of nitrate and organic secondary particulate material were also important. The diurnal trends in BC, RH, PM2.5, and Bp, scat (corrected for coarse particle scattering) are shown in The BC and scattering patterns in were also mirrored by CO concentrations taken 4 miles away from the Lindon sampling site (not shown here). The evening peaks between about 7 p.m. and 11 p.m. can be attributed to home combustion of wood (CitationHansen et al., 2010). While these BC peaks are also associated with increases in scattering, both the scattering and fine particulate mass continue to increase during the period after the end of the BC peak. The apportionment results from the 2007 study indicate that the increasing fine particle concentrations are associated with both organic and nitrate secondary material formed after the wood smoke emissions have been released (CitationHansen et al., 2010). Fine particle light scattering follows this same trend, shown in , indicating that secondary aerosol is an important, if not dominant, contributor to light scattering at the Lindon sampling site.
Figure 4. A comparison between light scattering, percent RH, PM2.5, and black carbon from March 8–11. The trends shown here indicate that secondary aerosol formation is likely the dominant contributor to light scattering, since scattering continues to increase after the end of the black carbon peaks.
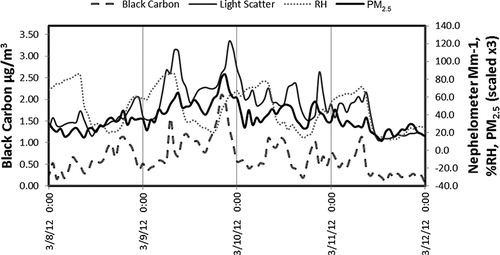
Rubidoux study: Measurement of fine particulate mass including the water content
One weakness of the mass-scattering correlation revealed from the Lindon 2012 data is the lack of measurement of particle-associated water using the FDMS TEOM. Such correlations could be improved and the prediction of human perception of visibility better estimated using PM measured mass that did include the particulate water in the measurement. Likewise, using a conventional TEOM will not measure this particle-bound water. In addition, because the sample collection filter is heated, the TEOM will not measure particulate semivolatile species such as ammonium nitrate or semivolatile organic material.
During the 2003 study at Rubidoux, CA (CitationGrover et al., 2005), PM2.5 mass was measured with both an FDMS TOEM and the RAMS (CitationObeidi et al., 2002), two instruments expected to measure total fine particulate mass except for the particle-associated water. In addition, PM2.5 and PM10-2.5 mass were measured with the Grimm model 1.107. The results of these measurements are shown in As indicated, the three measurements agree except for the times of high relative humidity when the Grimm gave higher results than the other two measurements.
Figure 5. Comparisons of PM2.5 data obtained using the Grimm model 1.107 with (A) FDMS TEOM PM2.5 mass and (B) the average of FDMS TEOM and RAMS measured PM2.5 mass. The difference between and Grimm and other mass measurements is shown, in addition to relative humidity. All results are hourly averaged measurements.
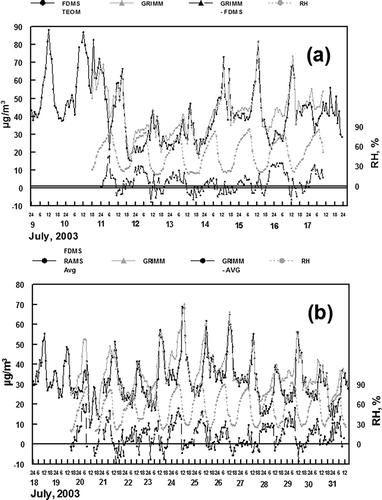
Peaks in the PM2.5 concentrations generally occurred during the midday period for each sampling day. This was a time of significant secondary ammonium nitrate and semivolatile organic material formation (CitationGrover et al., 2005). Relative humidity was generally low during this midday time period. The Grimm and comparison monitor results are in good agreement during these periods of low relative humidity, indicating that the protocols used to convert the Grimm monitor volume distribution data to a PM2.5 mass concentration are robust. In contrast, during the overnight periods of high relative humidity, the results obtained with the Grimm monitor are consistently higher than the results obtained with the comparison monitors. As described earlier, the comparison monitors do not measure fine particulate water content. It is probable that the higher concentrations measured during the high relative humidity time periods with the Grimm monitor may reflect the increased volume of aerosol associated with fine particulate water.
The uptake of water by the fine aerosol can be estimated during time periods when NH4SO4 and NH4NO3 chemical composition data were available (as shown in ). The particle uptake of water with increasing humidity increases significantly due to these two particle-bound species (Tang et al., 1981). The effect of relative humidity on water content was estimated from the data of Tang et al. using a previously described protocol to average the hysteresis effects on the data for pure ammonium sulfate (Sisler et al., 1992; CitationMalm et al., 1994). The effect of particle-bound ammonium nitrate is slightly less pronounced; however, we have used the same relative humidity curve to approximate the effect of both NH4SO4 and NH4NO3 (Tang et al., 1981). Both sulfate and nitrate are expected to exist as NH4SO4 and NH4NO3 due to the high amount of gas-phase NH3 in the Rubidoux area.
Figure 6. Relative humidity, NH4NO3, and (NH4)2SO4 data used to estimate the uptake of water by the fine aerosol being sampled. Data given reflect the day and hours when the sulfate and nitrate data were available. The hypothesis was that the positive differences between the Grimm monitor and comparison samplers was due to aerosol water.
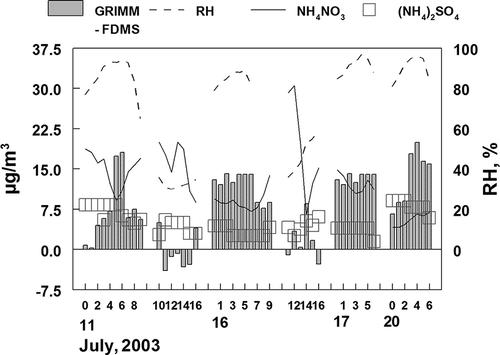
As seen in , sulfate concentrations did not vary significantly as a function of time of day or relative humidity. However, significant diurnal patterns in ammonium nitrate were seen, with the highest concentrations being present at midday. This was also a time of lower relative humidity. The diurnal pattern of the ammonium nitrate indicates the need for a shorter averaging time period than daily averages.
Since the highest concentrations of ammonium nitrate do not occur during periods of highest relative humidity, the calculation of water content using the data in provides a stringent test of the origin of the difference between the Grimm monitor and comparison sampler results. In , the PM2.5 mass difference between the Grimm monitor and the comparison sampler is compared to the calculated mass of water in the aerosol due to ammonium nitrate, ammonium sulfate, and relative humidity changes. Deviations from the slope of 1 are not any larger than the uncertainties in the assumptions used in the calculations and the data points do scatter uniformly around this line. The assumption that the higher mass measurements seen by the Grimm 1.107 monitor are due to water uptake is reasonable. In should be noted that the Grimm model 180 monitor with a Nafion dryer system does not measure particle-associated water (CitationLong et al., 2005).
Conclusion
The collection of hourly averaged data in the studies herein signifies the need for alternative instrumentation and the need for shorter averaging times if measured urban visibility is to be related to human perception. In areas where an FDMS TEOM is used to measure PM2.5, high humidity causes an underestimation of light extinction due to PM-bound water. This occurs because the particles-bound water has a sharp increase once the deliquescence point of NH4NO3 and (NH4)2SO4 is reached. In areas where hourly speciation data are not available, the calculation of light scattering will be skewed, especially if a 24-hr averaged value of NH4NO3 is used to calculate the MLEE, thus creating a bias for overestimating light scattering during times when RH is high and NH4NO3 levels are actually low. This is especially the case at nighttime. More significantly, the EPA proposed standard will not account for diurnal variation of visibility degradation, and therefore it will poorly relate to human visibility assessment. The correlations between particle mass and scattering reported here would be improved by the use of instruments akin to the Grimm model 1.107 monitor, which measures particle associated water. Furthermore, obtaining data using a nephelometer and a Grimm model 1.107 monitor together, would show the accuracy of scattering measurements based on reliable mass measurement systems (such as the Grimm). Such measurements are recommended.
Acknowledgment
This research was supported by Southern California Edison. Appreciation is expressed to CIRA, Fort Collins, CO, for the use of the nephelometer.
References
- Ding , Y.M. , Pang , Y.B. and Eatough , D.J. 2002 . High-volume diffusion denuder sampler for the routine monitoring of fine particulate matter: I. Design and optimization of the PC-Boss . Aerosol Sci. Technol. , 36 ( 4 ) doi: 10.1080/027868202753571205
- Finlayson-Pitts , B.J. and Pitts , J.N.J. 2000 . Chemistry of the Upper and Lower Atmosphere: Theory, Experiments, and Applications. , San Diego , CA : Academic Press .
- Grimm , H. and Eatough , D.J. 2009 . Aerosol measurement: The use of optical light scattering for the determination of particulate size distribution, and particulate mass, including the semi-volatile fraction . J. Air Waste Manage. Assoc. , 59 ( 1 ) doi: 10.3155/1047-3289.59.1.101
- Grover , B.D. , Eatough , N.L. , Woolwine , W.R. , Cannon , J.P. , Eatough , D.J. and Long , R.W. 2008 . Semi-continuous mass closure of the major components of fine particulate matter in Riverside, CA . Atmos. Environ. , 42 ( 2 ) doi: 10.1016/j.atmosenv.2007.09.037
- Grover , B.D. , Eatough , N.L. , Woolwine , W.R. , Eatough , D.J. and Cary , R.A. 2009 . Modifications to the Sunset Laboratory carbon aerosol monitor for the simultaneous measurement of PM2.5 nonvolatile and semi-volatile carbonaceous material . J. Air Waste Manage. Assoc. , 59 ( 8 ) doi: 10.3155/1047-3289.59.8.1007
- Grover , B.D. , Kleinman , M. , Eatough , N.L. , Eatough , D.J. , Hopke , P.K. , Long , R.W. , Wilson , W.E. , Meyer , M.B. and Ambs , J.L. 2005 . Measurement of total PM(2.)5 mass (nonvolatile plus semivolatile) with the filter dynamic measurement system tapered element oscillating microbalance monitor . J. Geophys. Res. Atmos. , 110 ( D7 ) doi: 10.1029/2004jd004995
- Hansen , J.C. , Woolwine , W.R. III , Bates , B.L. , Clark , J.M. , Kuprov , R.Y. , Mukherjee , P. , Murray , J.A. and al , et . 2010 . Semicontinuous PM2.5 and PM10 mass and composition measurements in Lindon, Utah, during winter 2007 . J. Air Waste Manage. Assoc. , 60 ( 3 ) doi: 10.3155/1047-3289.60.3.346
- Hyslop , N.P. 2009 . Impaired visibility: The air pollution people see . Atmos. Environ. , 43 ( 1 ) doi: 10.1016/j.atmosenv.2008.09.067
- Long , R.W. , Modey , W.K. , Smith , P.S. , Smith , R. , Merrill , C. , Pratt , J. , Stubbs , A. and al , et . 2005 . One- and three-hour PM2.5 characterization, speciation, and source apportionment using continuous and integrated samplers . Aerosol Sci. Technol. , 39 ( 3 )
- Malm , W.C. , Gebhart , K.A. , Molenar , J. , Cahill , T. , Eldred , R. and Huffman , D. 1994 . Examining the relationship between atmospheric aerosols and light extinction at Mount Rainier National Park and North Cascades National Park . Atmos. Environ. , 28 ( 2 ) doi: 10.1016/1352-2310(94)90110-4
- Obeidi , F. , Eatough , N.L. and Eatough , D.J. 2002 . Use of the RAMS to measure semivolatile fine particulate matter at Riverside and Bakersfield, California . Aerosol Sci. Technol. , 36 ( 2 ) doi: 10.1080/027868202753504065
- Pitchford, M. 2010. Assessment of the use of speciated PM2.5 mass-calculated light extinction as a secondary PM NAAQS indicator of visibility http://www.epa.gov/ttn/naaqs/standards/pm/s_pm_2007_td.html (http://www.epa.gov/ttn/naaqs/standards/pm/s_pm_2007_td.html)
- U.S. Environmental Protection Agency . 2011 . Policy Assessment for the Review of the Particulate Matter National Ambient Air Quality Standards. EPA 452/R-11-003 U.S. EPA http://www.epa.gov/ttnnaaqs/standards/pm/data/20110419pmpafinal.pdf
- U.S. Environmental Protection Agency . 2012 . National Ambient Air Quality Standards for Particulate Matter; Proposed rule . Fed. Reg. , 77 ( 126 ) : 38889 – 39055 .