Abstract
This study used pollution roses to assess sulfur dioxide (SO2) pollution in a township downwind of a large petrochemical complex based on data collected from a single air quality monitoring station. The pollution roses summarized hourly SO2 concentrations at the Taishi air quality monitoring station, located approximately 7.8–13.0 km south of the No. 6 Naphtha Cracking Complex in Taiwan, according to 36 sectors of wind direction during the preoperational period (1995–1999) and two postoperational periods (2000–2004 and 2005–2009). The 99th percentile of hourly SO2 concentrations 350˚ downwind from the complex increased from 28.9 ppb in the preoperational period to 86.2–324.2 ppb in the two postoperational periods. Downwind SO2 concentrations were particularly high during 2005–2009 at wind speeds of 6–8 m/sec. Hourly SO2 levels exceeded the U.S. Environmental Protection Agency (EPA) health-based standard of 75 ppb only in the postoperational periods, with 65 exceedances from 0–10˚ and 330–350˚ downwind directions during 2001–2009. This study concluded that pollution roses based on a single monitoring station can be used to investigate source contributions to air pollution surrounding industrial complexes, and that it is useful to combine such directional methods with analyses of how pollution varies between different wind speeds, times of day, and periods of industrial development.
The pollution roses summarize SO2 concentrations by wind direction and to investigate source contribution to air quality. Percentile statistics can catch pollution episodes occurring in a very short time at specific wind directions and speeds. The downwind areas have already exceeded regulated 1-hr SO2 standard since the operation of the complex.
Introduction
Petrochemical industrial complexes spanning hundreds of acres have been important manufacturing bases for growing the national economies of many developing countries in Asia, such as Taiwan and Korea, since the 1970s (CitationChen et al., 2007; CitationKim et al., 2002) and in China and many South Asian countries since the 1990s (CitationCharmondusit and Keartpakpraek, 2011; CitationZeng and Bathelt, 2011). Situated on the west coast of central Taiwan, the No. 6 Naphtha Cracking Complex is the largest petrochemical complex in Taiwan. With a total area of 2603 ha, this facility houses 64 plants within a single complex, including oil refineries, naphtha cracking plants, cogeneration plants, coal-fired power plants, heavy machinery plants, boiler plants, and downstream petrochemical-related plants in Mailiao Township, Yunling County, Taiwan (Formosa Petrochemical Corporation [FPCC], 2012).
The major air pollutants emitted from power stations and petrochemical industries include sulfur oxides (CitationJones and Harrison, 2011; CitationLuria et al., 2001), nitrogen oxides, carbon dioxide, carbon monoxide (CitationCastell et al., 2010; CitationVardar and Yumurtaci, 2010), volatile organic compounds (CitationBaltrenas et al., 2011; CitationChan et al., 2006), polycyclic aromatic hydrocarbons (CitationHu et al., 2011), metals (CitationMoreno et al., 2008; CitationPancras et al., 2011), and gaseous mercury (CitationMazur et al., 2009; CitationXu and Akhtar, 2009). Among these pollutants, sulfur dioxide (SO2) is important not only because of its key role as an exposure indicator for the petrochemical industry but also because of its health effects. Previous epidemiological studies have reported significant associations between annual average SO2 concentrations and respiratory symptoms among residents living near petrochemical facilities in Taiwan (CitationChen et al., 1998; CitationYang et al., 1998; CitationYang et al., 1997). A recent epidemiological study showed that short-term episodes of increased SO2 exposure from refinery stack emissions were associated with a higher number of asthma episodes in nearby Canadian children (CitationSmargiassi et al., 2009). Additionally, the U.S. Environmental Protection Agency (EPA) has promulgated a new hourly SO2 standard of 75 ppb based on acute exposure effects. The proposed standard is based on the 3-yr average of the 99th percentile concentration of the daily 1-hr maximum concentration for a year. Therefore, an improved approach for estimating the extreme values of SO2 and the health risks associated with such values for residents in neighboring downwind areas of industrial complexes is needed.
Pollution roses (PRs), sometimes called “concentration roses,” are used to calculate the average concentrations of a pollutant at a receptor from multiple emission sources over a period (usually a day) as a function of wind direction (CitationNic et al., 2012). In the past, many countries have used PRs to assess the environmental air pollution of industrial facilities. A 12-month study used PRs with 10˚ wind direction sectors at power stations and a petrol refinery based on average SO2 concentrations in Puertollano, Spain (CitationMoreno et al., 2006). Another study used 22.5˚ angular resolution PRs based on the annual average, median, maximum, and 90th percentile SO2 concentrations near an oil refinery plant in La Plata, Argentina, over a 4-yr period (CitationRatto et al., 2006). In addition, 10˚ angular resolution PRs based on daily averaged SO2 concentrations from eight monitoring sites were applied to the Antwerp harbor region of Belgium (CitationCosemans et al., 2008). These pollution rose methods have been used to identify unknown sources of air pollution at industrial sites, but the common feature of PRs is that they use only averaged concentrations or individual statistical parameters in their calculations. Using averages without the extreme values in PRs may not reveal the contributions of actual emissions to concentrations when pollution levels fluctuate rapidly over a short period of time.
In the present study, we combined a geographic information system (GIS)-based method incorporating point source emissions and limited air quality data with nontraditional PRs at a single air quality monitoring station over a 15-yr period to assess SO2 pollution in the surrounding areas of a large petrochemical complex.
Materials and Methods
Study area
The Taishi air quality monitoring station is located 6.4 km south of the southern border of the No. 6 Naphtha Cracking Complex and approximately 7.8–13.0 km south of the SO2 emission sources in this complex. The air quality monitoring station was installed in the Taishi Township by the Taiwan Environmental Protection Administration (TEPA) in 1993 and was the only air quality monitoring station near this complex available to evaluate the complex's emission contributions to air quality deterioration for the approximately 61,600 residents in the Mailiao and Taishi townships (together totaling an area of 134 km2) in 2012 (Taiwan Ministry of Interior [CitationTMOI], 2012).
The No. 6 Naphtha Cracking Complex's refineries, crackers, and cogenerators began major operations in 2000, and the complex has undergone one large production expansion since 2002. In 2000, a coal-fired power plant at the complex began generating 1800 MW of power. As of 2003, a circulating fluidized bed boiler, which generates approximately 300 MW of power annually, uses petroleum coke (a by-product of the oil-refining process) containing 6.5–8.5% sulfur as fuel. A total of three cogeneration plants and a circulating fluidized bed boiler began generating 2820 MW of power in 2004. In 2002, the three oil refinery plants processed 450,000 barrels of crude oil per day, and the two naphtha cracking plants produced 160 million tons of ethylene. The three oil refinery plants constitute Taiwan's second largest oil refinery, with a designed capacity of 450,000–540,000 barrels per day as of 2007. By adding a new cracking plant and expanding an existing one, annual ethylene production reached 2.9 million tons in 2007.
The Taishi air quality monitoring station and the No. 6 Naphtha Cracking Complex in Yunlin County were identified using the Universal Transverse Mercator (UTM) coordinate system ().
Emission inventory
The point source emissions inventory data from 2000 to 2009 were obtained from the Taiwan Emission Data System (TEDS 4.2 to TEDS 7.1) published by the TEPA (http://ivy2.epa.gov.tw/air-ei/new_main2.htm). The Air Pollution Control Act in Taiwan requires companies to submit their emissions to the TEPA annually using an emission factor method (CitationChan et al., 1996). TEDS uses emission factors from AP-42 of the EPA (CitationEPA, 1995), actual emission strength, location coordinates, stack height, stack diameter, stack exit gas temperature, stack exit gas velocity, chemical species, standard industrial classification (SIC), and pollution controls to estimate the annual sulfur oxide (SOx) emissions for each source classification code (SCC; http://www.epa.gov/ttn/chief/net/neip/appendix_6.mdb).
GIS
A GIS map was generated using the open source geospatial software Quantum GIS 1.74 (QGIS 2012), Google Earth 6.1, and Surfer 9.0 (Golden Software Inc., Boulder, CO, USA) to show the location and arrangements of the petrochemical complex, five categories of major SO2 emission sources (defined in this study as a source that emits more than 10 tons per year), seven major roads, and the residential areas in the Mailiao and Taishi townships. The GIS map of SOx point emissions was imported from the Taiwan Emission Data System 7.1 into QGIS. We converted the shape files into Google Earth's KML files to correctly layer the point emission sources over aerial photographs and Google Earth's satellite imagery in QGIS. The complete GIS map of the No. 6 Naphtha Cracking Complex, including its major SOx emission sources and the Taishi air quality monitoring station, is shown in
Air quality and meteorological monitoring data
The Taishi air quality monitoring station continuously monitors air quality for SO2, PM10 (particulate matter with an aerodynamic diameter ≤10 μm), PM2.5 (PM with an aerodynamic diameter ≤2.5 μm), CO, NO, NO2, NOx (nitrogen oxides), CH4, NMHC (nonmethane hydrocarbon), and ozone, as well as wind direction and speed (TEPA, 1993). In this study, hourly SO2 concentrations and meteorological conditions from 1995 to 2009 were used to plot PRs. The SO2 analyzer is based on an optical spectroscopy method (Ecotech model 9850; Ecotech Inc., Victoria, Australia). Wind speed was measured using cup anemometers (model 014A; Met One Instruments, Inc., Grants Pass, Oregon, USA), and wind direction was measured using a vane (model 024A, Met One Instruments). The TEPA has a quality assurance protocol to maintain the accuracy and reliability of the data. Hourly data of wind speed and direction over 1995–2009 were summarized and presented as a wind rose in using Microsoft Excel 2010 (Microsoft, Inc., Seattle, WA, USA). Apparent dominance in wind data towards 20–30° in implies that the meteorological data at Taishi station can be used to represent the typical annual average wind speed and direction in the area, which is dominated by a northeast prevailing wind.
Pollution roses
In this study, we used a pollution rose (PR) method to summarize the hourly SO2 data from the Taishi air quality monitoring station by examining 36 sectors of wind direction over the petrochemical complex's three operational phases from 1995 to 2009: the preoperational phase of 1995–1999, the postoperational phase of 2000–2004, and a second postoperational phase of 2005–2009. These categories were based on the operation and expansion of the petrochemical complex and served to quantify the contribution of emission sources to SO2 concentrations in the areas downwind of the petrochemical complex. In addition to PRs based on average concentrations, we also used percentile-based PRs to investigate whether the inclusion of statistics representing the full range of data can more accurately reveal the relationship between emission sources and their impact areas. Our PR was divided into 36 10˚ wind direction sectors. Microsoft Excel 2010 was then used to identify the prevailing wind directions for each hourly datum. We generated our PRs not only for the means but also for the 25th (first quartile, Q1), 50th (median), 75th (third quartile, Q3), 90th, 95th, and 99th percentiles of hourly SO2 air quality monitoring data. The average-based PR can serve as an indicator of longer-term regional or urban background levels of pollution, whereas percentile-based PRs can indicate shorter-term impacts such as plume impacts for SO2.
Statistical analysis
Summary statistics were calculated using SAS version 9.2 (SAS Institute, Inc., Cary, NC, USA). Radar plotting in Microsoft Excel 2010 was used to plot all percentile PRs. We plotted our PRs divided into three time periods based on the complex's three operational phases to compare differences over time. Based on proximity to the areas upwind and downwind of the petrochemical complex, the PRs of the Taishi air quality monitoring station provided the optimal downwind SO2 emission measurements from the complex. The generated PRs were further analyzed to determine when and at what wind speed the high SO2 concentrations occurred in the downwind areas.
Results and Discussion
As shown in , the SOx emission sources listed in the inventories were sorted into six categories: cogeneration plants (2549 ton/yr), a coal power plant (1803 ton/yr), oil refineries (654 ton/yr), aromatics and plastics plants (493 ton/yr), naphtha cracking plants (403 ton/yr), and downstream petrochemical plants (314 ton/yr) in 2009. SOx were potentially emitted from the 381 stacks of the 64 plants in the complex. The cogeneration plants contributed 41% of the SOx emissions in this complex from three 250-m stacks, and the coal power plants contributed another 29% emissions from 14 stacks with height from 150 to 250 m. Together, the power plants were the highest SOx emission sources (70%) in this complex, with average temperatures of 30–55 °C and velocities of 12.6–17.7 m/sec in the exhaust gas.
Table 1. Major SO2 emissions from manufacturing plants of the No. 6 Naphtha Cracking Complex in Taiwan in 2009
The emission inventory indicates that SOx was emitted in the complex not only from petrochemical manufacturing processes but also from power generation facilities. shows the 15 major emission sources in the Taiwan Emission Data System for which spatial coordinates have been designated and which have emissions greater than 10 ton/yr.
shows a summary of the annual SO2 concentration statistics for the Taishi air quality monitoring station from 1995 to 2009. More than 90% of the hourly SO2 data were available for each year at the Taishi station. The SO2 concentrations varied widely within each year, as indicated by the large standard deviations and maximums. The variation in SO2 concentrations has increased since 2001. The annual average SO2 concentrations decreased slightly but consistently over the 1995–1999 time period, but then increased over the 2001–2009 time period. The hourly maximum SO2 concentrations, which were 36.9 ppb in 2000, increased even more rapidly over the same period, reaching 335.6 ppb in 2005 and stabilizing between 103.8 and 180.3 ppb during the 2006–2009 time period. The general decrease in 3-yr moving average SO2 concentrations in Taishi station during 1995–1999 before the operation of the No. 6 Naphtha Cracker is a result of stringent sulfur content regulations in transportation fuels by TEPA since 1997. The allowable sulfur content in diesel fuel was gradually reduced from 3000 ppm in 1996 to 1500 ppm in 1997, and 500 ppm in 1998. A 50 ppm cap was put on gasoline fuel since 2007. Using the EPA primary standard for 1-hr daily maximum SO2 concentrations (75 ppb) as the cutoff level to compare hourly data from the Taishi air quality monitoring station, we found no concentrations above this level before 2000, but several higher days per year since 2001.
Table 2. Summary statistics for hourly SO2 concentrations (ppb) at the Taishi air quality monitoring station from 1995 to 2009
Figure 2 shows the relationship between the 3-yr moving averages of the 99th percentile SO2 concentrations from 1995 to 2009 in the Taishi air quality monitoring station and the corporate-reported annual SOx emissions since 2000. SO2 concentrations increased with SOx emissions between 2000 and 2006, but this relationship disappears after 2007. One possible reason of the disappeared relationship between emissions and concentrations after 2007 is that SO2 in recent years were emitted from higher and/or hotter stacks, and stayed longer in the atmosphere before it impacted at ground level. The phenomenon cannot be explained by changes in favorable meteorological conditions for peak concentrations because there is no significant variation in wind speed and direction over the years. The EPA primary standard for 1-hr daily maximum SO2 concentrations was violated at the Taishi air quality monitoring station between 2001 and 2003 when the annually reported SOx emissions from the complex exceeded 3000 tons. The 3-yr moving average of the 99th percentile SO2 concentrations peaked at 137.3 ppb between 2004 and 2006 when the annual SOx emissions reached 3936.4 tons. It should be noted that SOx emissions reported by the company kept increasing during 1995–2009 but SO2 concentration increased initially and then decreased. This may reflect variation in reporting procedure or underreporting of SOx emissions by the companies over the years. It has been reported that SOx emissions from flares, which were in operation for over 300 days per year, were not included in the emission inventory.
Figure 3 shows six SO2 PRs at the Taishi air quality monitoring station over the three operational phases. The downwind sectors of the Taishi air quality monitoring station relative to the No. 6 Naphtha Cracking Complex are 0–15˚ and 275–360˚. The PRs based on averages in Figure 3a–c show that the average SO2 concentrations increased from 4.8 ppb in the preoperational period (1995–1999) to 14.4–32.0 ppb in the two postoperational periods (2000–2004 and 2005–2009) at the 350˚ sector. Percentile PRs (Figure 3d–f) show that the 99th percentile of hourly SO2 concentrations increased from 28.9 ppb in the preoperational period to 86.2–324.2 ppb in the two postoperational periods at the 350˚ sector. The PRs also show an increasing frequency of high SO2 concentrations occurring first at the 0˚, 10˚, and 350˚ sectors in 2000–2004, and then spreading to the 0˚, 10˚, 280˚, 290˚, 300˚, 330˚, and 350˚ sectors in 2005–2009, compared with the PRs in 1995–1999. There seem to be more peak SO2 concentrations from the west in the later years (2005–2009). One possibility is new sources of increasing busy shipping on the Taiwan Straits and the sea–land recirculation of ever bigger emissions from the complex in recent years.
Air pollution related to industrial sources is sometimes underestimated by limited air quality monitoring sites and/or underused air quality monitoring data. In this case, the Taishi air quality monitoring station is the only monitoring station measuring a limited number of pollutants in the neighboring areas of this petrochemical complex. We did not estimate the relation between SO2 concentrations and SOx emissions in this study. However, traffic emissions are presumably a major SO2 source in phase 1 (1995–1999) and the SO2 concentrations measured in this period may reflect the traffic contribution to SO2 concentrations in the study area. Residential emission of SO2 is limited because liquid petroleum gas is the main cooking fuel for the residents in this area. Our PRs and GIS map reveal temporal and spatial patterns of air pollution in the neighboring areas of this complex that had not been well documented by traditional statistics in the past. Our PRs also reveal that the downwind areas had already become nonattainment areas for SO2 pollution in 2000, when the inventory of SOx emissions from the complex was approximately 3000 tons per year, which was well below the allowable emissions limits based on the environmental impact assessment. Our PRs can serve as a useful tool to cross-validate model-based air pollution regulations.
To investigate the effect of wind speed on pollution levels, we further classified the 1995–2009 hourly SO2 concentrations according to wind speed during sea-wind, land-wind, and downwind periods at the Taishi air quality monitoring station over the three operational phases. The frequency distributions of wind-speed-specific SO2 concentrations for the sea-wind (185–275˚), land-wind (15–185˚), and downwind (0–15˚ and 275–360˚) sectors are shown in –c. The downwind effect on SO2 concentration was not significant in phase 1 (1995–1999) but become significant since phase 2 (2000–2004). It should be noted high wind speeds (>4 m/sec) occurred mostly during daytime (11:00 a.m. to 4:00 p.m.) when high SO2 concentrations were measured at Taishi during phase 2 (2000–2004) and phase 3 (2005–2009). As shown in , downwind SO2 concentrations were always higher than sea- and land-wind SO2 concentrations regardless of wind speed, with the greatest differences occurring at wind speeds of 6–8 m/sec. The downwind/land-wind ratios of SO2 concentrations increased from 1.2 at wind speeds of less than 1 m/sec to 5.1 at wind speeds between 6 and 7 m/sec. The downwind/sea-wind ratios of SO2 concentrations increased from 1.2 at wind speeds of less than 1 m/sec to 7.1 at wind speeds between 5 and 6 m/sec. The land-wind/sea-wind ratios of SO2 concentrations increased from 1.0 at wind speeds of less than 1 m/sec to 5.1 at wind speeds between 5 and 6 m/sec. Finally, the land-wind/sea-wind ratios decreased from 1.2 at wind speeds between 5 and 6 m/sec to 0.6 at wind speeds greater than 9 m/sec at the Taishi air quality monitoring station. indicates that “downwind” ground-level SO2 concentrations have become much more associated with high wind speeds during the recent phase (2005–2009), compared with earlier phases (1995–1999 and 2000–2004). This phenomenon supports the suggestion that the recent divergence between emission and concentration trends shown in is due to generally higher discharge heights during the recent phase (2005–2009). Specifically, it seems that such higher discharge heights have led to higher plumes, which therefore require higher wind speeds to bring them down to ground level.
Figure 2. The 99th percentile 3-yr average SO2 concentration and 3-yr moving average SOx emissions at the Taishi air quality monitoring station from 1995 to 2009.
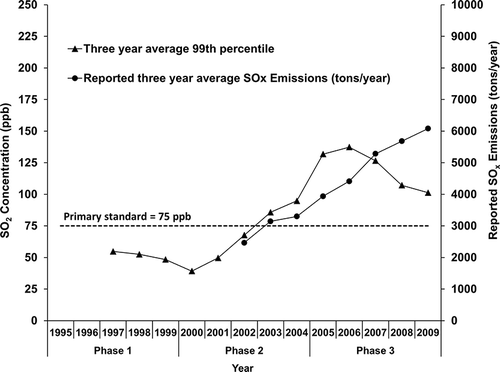
Figure 3. SO2 pollution roses of averages (a, b, c) and percentiles (d, e, f) at the Taishi air quality monitoring station from 1995 to 2009.
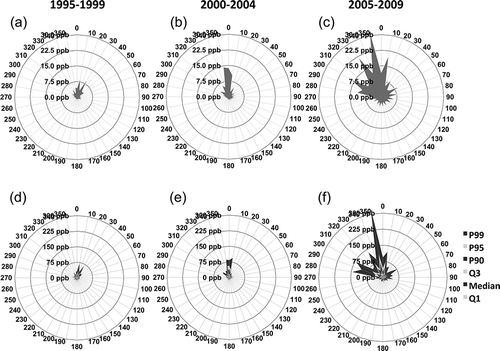
Figure 4. Wind-speed-specific SO2 concentrations (mean + SD) during emission-affecting periods (downwind) from the petrochemical complex and traffic emission-affecting periods (land-wind) and nonaffecting periods (sea-wind) at the Taishi air quality monitoring station from 1995 to 2009. (a) 1995–1999, (b) 2000–2004, and (c) 2005–2009.
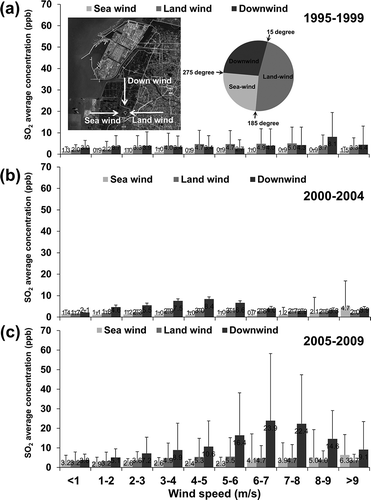
Figure 5 shows the diurnal analysis of SO2 concentrations at the Taishi air quality monitoring station over the three operational phases. As shown in , SO2 diurnal concentrations during sea-wind periods were significantly lower than those during down- and land-wind periods in phase 1. The overlap of diurnal trends of SO2 during downwind and land-wind in phase 1 indicates a regional air pollution situation of SO2 concentrations at Taishi in the absence of emissions from the No. 6 Naphtha Cracker. These two overlapped diurnal trends started to separate since phase 2, indicating a growing influence of emissions from the No. 6 Naphtha Cracker on SO2 concentrations at Taishi in phases 2 and 3. As shown in , the hourly SO2 concentrations in phase 3 were consistently higher during downwind periods when the measurements at the monitoring station were affected by emissions from the complex, compared with during land- and sea-wind periods when the measurements were unaffected by emissions from the complex. It is worthwhile to note that the SO2 concentration for the land-wind case has the similar character as the downwind case. Such phenomenon can be attributable to inland emission sources and the recirculation of emissions from the complex. The greatest contrast between downwind, land-wind, and sea-wind periods in Taishi occurs in the early afternoon between 1:00 p.m. and 3:00 p.m. and in the evening at 8:00 p.m. The downwind/land-wind ratios of SO2 concentrations at these times were 1.9–3.8 in phase 3 and were higher than the average ratio of 1.5 at other hours of the day. The downwind/sea-wind ratios of SO2 concentrations were 3.7–5.8 in phase 3 and were higher than the average ratio of 1.8 at other hours of the day. The land-wind/sea-wind ratios of SO2 concentrations were 1.1–2.3 in phase 3. indicates that “downwind” ground-level SO2 concentrations have become much more associated with convective dispersion conditions around the midafternoon (∼12:00 p.m. to 4:00 p.m.) during the recent phase (2005–2009), compared with earlier phases (1995–1999 and 2000–2004). This phenomenon supports that the recent divergence between emission and concentration trends shown in is due to generally higher discharge heights during the recent phase (2005–2009). Specifically, it seems that such higher discharge heights have led to higher plumes, which need deeper and more vigorous convection, which occurs mostly in the midafternoon, to bring them down to ground level.
Figure 5. Diurnal SO2 concentrations during emission-affecting periods (downwind) from the petrochemical complex and traffic-affecting periods (land-wind) and nonaffecting periods (sea-wind) at the Taishi air quality monitoring station from 1995 to 2009. (a) 1995–1999, (b) 2000–2004, and (c) 2005–2009.
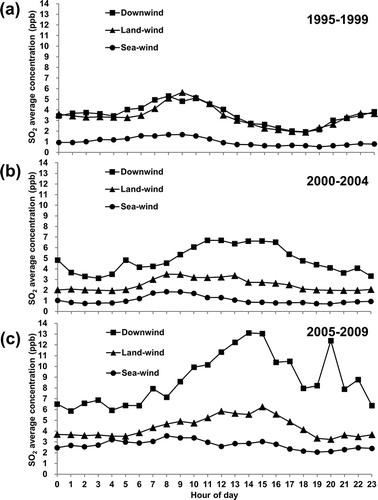
Our percentile PR, a refined form of the traditional PR, performs effectively in revealing the actual impacts of emission sources on air quality. Long-term PR statistics can be used to detect pollution episodes occurring within a short period at specific wind directions and speeds and to determine the times of day when such episodes occur. The conventional way of summarizing air pollution data without considering wind direction and speed is insufficient in assessing the impact of industrial emissions on air quality in the surrounding areas of an industrial complex, such as the No. 6 Naphtha Cracking Complex in Taiwan. The operation of the No. 6 Naphtha Cracking Complex contributes significantly to the elevated SO2 concentrations in the neighboring areas of the Mailiao and Taishi townships, as evidenced by examining the temporal and spatial comparisons of percentile PRs at the downwind air quality monitoring station.
The EPA has regulated a new 1-hr SO2 standard of 75 ppb, a level designed to protect public health from SO2 exposures. The downwind areas of the No. 6 Naphtha Cracking Complex have been exceeding this level since operations at the complex began. Efforts to reduce SOx emissions from the No. 6 Naphtha Cracking Complex to below 3000 tons per year are warranted to protect the health of susceptible populations, such as children, older people, and people with asthma, from SO2 pollution in the Mailiao and Taishi townships in Taiwan.
Conclusion
This study demonstrated the utility of pollution roses, which summarize SO2 concentrations according to wind direction at a single air quality monitoring station, for investigating the point source contributions to air pollution surrounding a petrochemical complex. It is useful to combine such directional methods with analyses of how pollution varies between different wind speeds, times of day, and periods of industrial development. The pollution rose method is particularly applicable to resource-scarce developing countries for quantifying the air pollution in their industrial areas with limited air quality monitoring capability. This paper shows a significant disparity found between predicted plume impacts from dispersion models, as required by regulatory environmental impact assessment, and the monitored plume impacts at Taishi. Therefore, the performance of existing model-based regulatory environmental impact assessment (EIA) procedures should be improved in order to increase robustness of the EIA regulation. Furthermore, systematic air quality monitoring campaign should be carried out in order to guarantee the attainment of ambient air quality limits for human health protection in the impacted areas.
Acknowledgment
This study was sponsored by a research grant from the Environmental Protection Bureau of Yun-Lin County, Taiwan (YLEPB-99-022). The authors appreciate the reviewers’ insightful comments and suggestions, which significantly improved the academic merits of this paper.
References
- Baltrenas , P. , Baltrenaite , E. , Šerevičiene , V. and Pereira , P. 2011 . Atmospheric BTEX concentrations in the vicinity of the crude oil refinery of the Baltic region . Environ. Monit. Assess. , 182 : 115 – 127 . doi: 10.1007/s10661-010-1862-0
- Castell , N. , Mantilla , E. , Salvador , R. , Stein , A.F. and Millán , M. 2010 . Photochemical model evaluation of the surface ozone impact of a power plant in a heavily industrialized area of southwestern Spain . J. Environ. Manage. , 91 : 662 – 676 . doi: 10.1016/j.jenvman.2009.09.030
- Cetin , E. , Odabasi , M. and Seyfioglu , R. 2003 . Ambient volatile organic compound (VOC) concentrations around a petrochemical complex and a petroleum refinery . Sci. Total Environ. , 312 : 103 – 112 . doi: 10.1016/s0048-9697(03)00197-9
- Chan , C.C. , Nien , C.K. and Hwang , J.S. 1996 . Receptor modeling of VOCs, CO, NOx, and THC in Taipei . Atmos. Environ. , 30 : 25 – 33 . doi: 10.1016/1352-2310(95)00261-v
- Chan , C.C. , Shie , R.H. , Chang , T.Y. and Tsai , D.H. 2006 . Workers’ exposures and potential health risks to air toxics in a petrochemical complex assessed by improved methodology . Int. Arch. Occup. Environ. Health , 79 : 135 – 142 . doi: 10.1007/s00420-005-0028-9
- Charmondusit , K. and Keartpakpraek , K. 2011 . Eco-efficiency evaluation of the petroleum and petrochemical group in the map Ta Phut Industrial Estate, Thailand . J. Cleaner Prod. , 19 : 241 – 252 . doi: 10.1016/j.jclepro.2010.01.013
- Chen , J.F. , Wei , S.Q. , Chang , K.T. and Tsai , B.W. 2007 . A comparative case study of cultivated land changes in Fujian and Taiwan . Land Use Policy , 24 : 386 – 395 . doi: 10.1016/j.landusepol.2006.05.002
- Chen , P.C. , Lai , Y.M. , Wang , J.D. , Yang , C.Y. , Hwang , J.S. , Kuo , H.W. , Huang , S.L. and Chan , C.C. 1998 . Adverse effect of air pollution on respiratory health of primary school children in Taiwan . Environ. Health Perspect. , 106:331–335 doi: 10.1289/ehp.98106331
- Cosemans , G. , Kretzschmar , J. and Mensink , C. 2008 . Pollutant roses for daily averaged ambient air pollutant concentrations . Atmos. Environ. , 42 : 6982 – 6991 . doi: 10.1016/j.atmosenv.2008.04.025
- Formosa Petrochemical Corporation. No. 6 Naphtha Cracking Project—Magnitude and facilities http://www.fpcc.com.tw/English/No.6/magnitude%20and%20facilitles.htm (http://www.fpcc.com.tw/English/No.6/magnitude%20and%20facilitles.htm)
- Hu , S.W. , Chan , Y.J. , Hsu , H.T. , Wu , K.Y. , ChangChien , G.P. , Shie , R.H. and Chan , C.C. 2011 . Urinary levels of 1-hydroxypyrene in children residing near a coal-fired power plant . Environ. Res. , 111 : 1185 – 1191 . doi: 10.1016/j.envres.2011.07.004
- Jones , A.M. and Harrison , R.M. 2011 . Temporal trends in sulphate concentrations at European sites and relationships to sulphur dioxide . Atmos. Environ. , 45 : 873 – 882 . doi: 10.1016/j.atmosenv.2010.11.020
- Kim , T.G. , Kim , J.H. , Kim , Y.D. and Kim , K. 2002 . Current risk management status of the Korean petrochemical industry . J. Loss Prevent. Proc. Ind. , 15 : 311 – 318 . doi: 10.1016/s0950-4230(02)00014-1
- Luria , M. , Imhoff , R.E. , Valente , R.J. , Parkhurst , W.J. and Tanner , R.L. 2001 . Rates of Conversion of sulfur dioxide to sulfate in a scrubbed power plant plume . J. Air Waste Manage. Assoc. , 51 : 1408 – 1413 . doi: 10.1080/10473289.2001.10464368
- Mazur , M. , Mintz , R. , Lapalme , M. and Wiens , B. 2009 . Ambient air total gaseous mercury concentrations in the vicinity of coal-fired power plants in Alberta, Canada . Sci. Total Environ. , 408 : 373 – 381 . doi: 10.1016/j.scitotenv.2009.10.006
- Moreno , T. , Querol , X. , Alastuey , A. and Gibbons , W. 2008 . Identification of FCC refinery atmospheric pollution events using lanthanoid- and vanadium-bearing aerosols . Atmos. Environ. , 42 : 7851 – 7861 . doi: 10.1016/j.atmosenv.2008.07.013
- Moreno , T. , Querol , X. , Alastuey , A. , Santos , S.G and Gibbons , W. 2006 . Controlling influences on daily fluctuations of inhalable particles and gas concentrations: Local versus regional and exotic atmospheric pollutants at Puertollano, Spain . Atmos. Environ. , 40 : 3207 – 3218 . doi: 10.1016/j.atmosenv.2006.01.041
- McNaught, A.D., and A. Wilkinson. 2012. Compendium of Chemical Terminology—The Gold Book. Zurich: International Union of Pure and Applied Chemistry http://goldbook.iupac.org/W06679.html (http://goldbook.iupac.org/W06679.html)
- Pancras , J.P. , Vedantham , R. , Landis , M.S. , Norris , G.A. and Ondov , J.M. 2011 . Application of epa unmix and nonparametric wind regression on high time resolution trace elements and speciated mercury in Tampa, Florida aerosol . Environ. Sci. Technol. , 45 : 3511 – 3518 . doi: 10.1021/es103400h
- Ratto , G. , Videla , F. , Almandos , J. , Maronna , R. and Schinca , D. 2006 . Study of meteorological aspects and urban concentration of SO2 in atmospheric environment of La Plata, Argentina . Environ. Monit. Assess. , 121 : 325 – 340 . doi: 10.1007/s10661-005-9127-z
- Smargiassi , A. , Kosatsky , T. , Hicks , J. , Plante , C. , Armstrong , B. , Villeneuve , P. J. and Goudreau , S. 2009 . Risk of asthmatic episodes in children exposed to sulfur dioxide stack emissions from a refinery point source in Montreal, Canada . Environ. Health Perspect. , 117 : 653 – 659 . doi: 10.1289/ehp.0800010
- Taiwan Environmental Protection Administration. 2012. Taiwan Air Quality Monitoring Network http://taqm.epa.gov.tw/taqm/en/default.aspx (http://taqm.epa.gov.tw/taqm/en/default.aspx)
- Taiwan Ministry of Interior. 2012. Monthly bulletin of interior statistics, population, population for township and district since 1981 http://sowf.moi.gov.tw/stat/month/elist.htm (http://sowf.moi.gov.tw/stat/month/elist.htm)
- U.S. Environmental Protection Agency . 1995 . Compilation of Air Pollutant Emission Factors—Stationary Point And Area Sources, AP-42 , Vol. I , Research Triangle , NC : U.S. Environmental Protection Agency .
- Vardar , N. and Yumurtaci , Z. 2010 . Emissions estimation for lignite-fired power plants in Turkey . Energy Policy , 38 : 243 – 252 . doi: 10.1016/j.enpol.2009.09.011
- Xu , X. and Akhtar , U.S. 2009 . Identification of potential regional sources of atmospheric total gaseous mercury in Windsor, Ontario, Canada using hybrid receptor modeling . Atmos. Chem. Phys. , 9 : 24847 – 24874 . doi: 10.5194/acpd-9-24847-2009
- Yang , C.Y. , Wang , J.D. , Chan , C.C. , Chen , P.C. , Huang , J.S. and Cheng , M.F. 1997 . Respiratory and irritant health effects of a population living in a petrochemical-polluted area in Taiwan . Environ. Res. , 74 : 145 – 149 . doi: 10.1006/enrs.1997.3762
- Yang , C.Y , Wang , J.D. , Chan , C.C , Hwang , J.S and Chen , P.C. 1998 . Respiratory symptoms of primary school children living in a petrochemical polluted area in Taiwan . Pediatr. Pulmonol. , 25 : 299 – 303 . doi: 10.1002/(SICI)1099-0496(199805)25:5<299::AID-PPUL2>3.3.CO;2-T
- Zeng , G. and Bathelt , H. 2011 . Divergent growth trajectories in China's chemical industry: The case of the newly developed industrial parks in Shanghai . GeoJournal , 76 : 675 – 698 . doi: 10.1007/s10708-009-9313-6