Abstract
Multibillion-dollar strategies control ambient air ozone (O3) levels in the United States, so it is essential that the measurements made to assess compliance with regulations be accurate. The predominant method employed to monitor O3 is ultraviolet (UV) photometry. Instruments employ a selective manganese dioxide or heated silver wool “scrubber” to remove O3 to provide a zero reference signal. Unfortunately, such scrubbers remove atmospheric constituents that absorb 254-nm light, causing measurement interference. Water vapor also interferes with the measurement under some circumstances. We report results of a 3-month field test of two new instruments designed to minimize interferences (2B Technologies model 211; Teledyne-API model 265E) that were operated in parallel with a conventional Thermo Scientific model 49C O3 monitor. The field test was hosted by the Houston Regional Monitoring Corporation (HRM). The model 211 photometer scrubs O3 with excess nitric oxide (NO) generated in situ by photolysis of added nitrous oxide (N2O) to provide a reference signal, eliminating the need for a conventional O3 scrubber. The model 265E analyzer directly measures O3–NO chemiluminescence from added excess NO to quantify O3 in the sample stream. Extensive quality control (QC) and collocated monitoring data are assessed to evaluate potential improvements to the accuracy of O3 compliance monitoring.
Two new-technology ozone monitors were compared with a conventional monitor under field conditions. Over 3 months the conventional monitor reported more exceedances of the current standard than the new instruments, which could potentially result in an area being misjudged as “nonattainment.” Instrument drift can affect O3 data accuracy, and the same degree of drift has a proportionally greater compliance effect as standard stringency is increased. Enhanced data quality assurance and data adjustment may be necessary to achieve the improved accuracy required to judge compliance with tighter standards.
Introduction
The Clean Air Act has established air-quality criteria for photochemical oxidants and requires that local authorities establish monitoring networks to assess compliance with those standards and implement pollution controls when standards are not met. The National Ambient Air Quality Standard (NAAQS) for ozone was revised to 75 ppb for an 8-hr average in 2008 (CitationU.S. EPA, 2008a), and tightening the standard to 60–70 ppb was proposed in 2010 (CitationU.S. EPA, 2010). (Following U.S. EPA terminology, parts per billion “ppb” and “mixing ratio” are used in discussing O3 levels.) However, this change was put on hold in 2011 until the NAAQS is reconsidered in 2013 (CitationObama, 2011). The current 75 ppb O3 NAAQS is determined by the 3-yr mean of the annual fourth highest daily maximum 8-hr averages (CitationU.S. EPA, 2008a). Billions of dollars are spent to control ambient ozone levels in the United States so it is essential that compliance measurements be accurate.
The ethylene chemiluminescence Federal Reference Method (FRM), which was dominant in the U.S. monitoring network during the 1970s and 1980s, had only a single common interference, a 3–4% positive bias in the O3 reading for each percent water vapor in ambient air (CitationKleindienst et al., 1993; CitationHudgens et al., 1994; Mayer et al., 2006). However, during the 1990s, the chemiluminescence method was replaced by a Federal Equivalent Method (FEM), ultraviolet (UV) photometry, to reduce operational costs and potential fire hazards from the flammable compressed ethylene gas required by the FRM.
Current FEM O3 monitor performance criteria noted in allow lower detection limits of 10 ppb, daily zero-span drifts of 20 ppb, and interferences up to 60 ppb. The U.S. EPA has acknowledged that these monitor specifications no longer provide the necessary performance requirements for monitors used to support the current 75-ppb O3 NAAQS and has called for their revision (CitationU.S. EPA, 2011a). The new monitors tested in this study strive to meet these needs. Improved calibration practices may be needed as well. For example, automated subhourly zero-span drift adjustments are currently available in the JSC Optec model 3.02 P-A solid-state chemiluminescence O3 monitor recently submitted to U.S. EPA for FEM certification (CitationU.S. EPA, 2008b).
Table 1. Monitor performance specifications for O3 based in 40 CFR Part 53
Commercial UV FEMs compare the low-pressure mercury lamp 254-nm intensity transmitted through the sample with the intensity of the reference sample scrubbed of O3. However, conventional scrubbers also remove other atmospheric constituents that perturb transmission, which then represent interferences. Such constituents include water vapor (CitationMeyer et al., 1991; CitationLeston and Ollison, 1992; CitationHudgens et al., 1994; CitationMaddy, 1998; CitationWilson and Birks, 2006; CitationSpicer et al., 2010), aromatic hydrocarbons and their photochemical reaction products (CitationHuntzicker et al., 1979; CitationGrosjean and Harrison, 1985; CitationKleindienst et al., 1993, Citation1997; CitationLeston and Ollison, 2000; CitationZdanevitch, 2002; CitationLeston et al., 2005; CitationSpicer et al., 2010), mercury vapor (CitationLeston et al., 1992; CitationGodet, 1995; CitationLi et al., 2006), and sulfur dioxide (CitationU.S. EPA, 1996).
The influence of water vapor on UV photometers is complex. Humidity interferences are associated with scratched optical cell windows (CitationMeyer et al., 1991) and with changing transmission of multiply reflected UV light down the photometer cell, although a Nafion pre-cell humidity equilibrator apparently reduces this source of interference (CitationWilson and Birks, 2006). In published supplemental information, CitationWilson and Birks (2006) report that the humidity equilibrator eliminates a positive 100-ppb initial peak and 20-ppb baseline 2B model 202 (a single-beam version of the 205) shift in response to a 90% relative humidity step change in laboratory tests; CitationSpicer et al. (2010) report positive 8-hr average differences up to 4 ppb between paired humidity-controlled 2B Tech 202 and uncontrolled Dasibi 1008 or TE 49C monitors under more realistically changing humidity patterns at several sites in the Houston area. However, because other interferences remain (CitationSpicer et al., 2010), new techniques have been developed to improve the accuracy of ozone compliance monitoring.
Experimental Methods
Ozone monitors based on two new technologies were compared with a conventional UV photometer (Thermo Scientific model 49C) from August 26 to November 19, 2010, at HRM Site 3 (EPA 48-201-0803) in an industrial area near the Houston Ship Channel. Site details, maps, and photographs are available from the CitationTexas Commission on Environmental Quality (TCEQ, 2012); air quality and meteorology parameters measured at this site are noted in . The three O3 monitors were connected to the site ambient air sampling manifold through a common particulate matter filter. New monitor installation by the site operators proceeded smoothly, and monitoring was conducted with no obvious operational problems.
Table 2. Variables routinely measured at the Houston site
The ozone instruments operated at the site are listed in . The model 211 photometer uses excess NO to react with and remove O3 from the sample air to provide a reference signal (CitationBirks et al., 2010), with NO generated in situ by upstream photolysis of added nitrous oxide (N2O), eliminating the need for a conventional O3 scrubber. Ozone is quantified by conventional UV photometry. The instrument incorporates Nafion tubing upstream of the optical cell to equilibrate the sample and reference stream humidity, as described by CitationWilson and Birks (2006). The model 265E is similar to a nitrogen oxides (NOx) monitor but measures chemiluminescence from added NO to quantify ozone (CitationTeledyne-API, 2011). The sample is dried before analysis by a Perma-Pure Nafion drier and excess reagent NO is supplied from a compressed gas cylinder (1% NO/N2). This instrument was recently certified as a FEM by the CitationU.S. EPA (2011b).
Table 3. Specifications of O3 monitors used in this study
The three O3 monitors were operated under HRM quality assurance/quality control (QA/QC) protocols, which included automated daily zero/span checks, weekly 3-point calibration checks, monthly 5-point calibrations, and an independent quarterly performance audit conducted on October 4, 2010. Except for two anomalous model 49C baseline excursions on two days, the three instruments passed all QC checks (±15%) over the test period. Weekly and monthly multipoint calibrations were used to step adjust the weekly data from each instrument between calibrations for drift along with the full data set adjustment using the regression equations from the independent performance audit. Average weekly adjustments during the study were –0.2 ppb O3 for the 211 and +0.8 ppb O3 for the 265E. Routine daily zero/span checks (0, 400 ppb) also were evaluated to explore further reductions in instrument drift.
A volatile organic compound (VOC) interference test was conducted on the three monitors part way through the study. A certified 21-component (Praxair) hydrocarbon in N2 mixture (10 ppm each of ethylene, acetylene, ethane, propylene, propane, isobutane, 1-butene, 1,3-butadiene, butane, isopentane, 1-pentene, n-pentane, n-hexane, isoprene, benzene, toluene, styrene, ethylbenzene, methyl-tert-butyl-ether, trans-2-butene, and cis-2-butene) was diluted to 1.4 ppm (66 ppb per constituent) in a zero air stream containing 30 ppb O3. Although the 1.4 ppm test mixture level may exceed current average urban total VOC levels, CitationKleindienst et al. (1993) note that such levels may not exceed those encountered during maximal O3 levels under design value day conditions; nor does the test mixture contain the ambient aromatic photochemical reaction products demonstrated to cause enhanced conventional monitor measurement bias by CitationSpicer et al. (2010).
Results and Discussion
Houston, TX, has the most extensive air monitoring network in the United States. While this region has historically reported some of the highest O3 levels in the United States, the highest annual daily maximum hourly O3 values have decreased from 265 ppb to 149 ppb over the period 1987–2010; however, a number of the region's 52 monitoring sites exceeded the current daily maximum 75 ppb 8-hr average standard in 2010 (CitationHRM, 2011).
Instrument comparison
The data from the O3 instruments and other chemical and meteorological measurements made at HRM-3 were provided by HRM staff as hourly averages. Because the data we received from the model 49C monitor had been adjusted for weekly and monthly calibrations, we applied the same weekly and monthly calibration adjustments to the data from the two new instruments. All three instruments were calibrated weekly at 0, 100, and 400 ppb O3 and monthly at 0, 90, 200, 300, and 400 ppb O3 in Drierite dried zero air by an NIST-traceable Dasibi 5008 calibrator equipped with a UV ozone generator. We also used the results of the October 4, 2010, performance audit based on the TCEQ protocol by URS QA staff with a NIST-traceable Sabio 2030 transfer standard to adjust the data for all three instruments over the entire study period. These comparably adjusted data form the basis of the subsequent instrument comparisons and assessments of various additional QC adjustments to the data.
Because the current O3 NAAQS is based on an 8-hr averaging period, we constructed rolling 8-hr averages for each O3 monitor shown in (offset for visual comparison). The diurnal O3behavior is evident, as are occasional 8-hr average values exceeding the 75-ppb standard at this site. Overall, the hourly time series trends track one another well, and regression analysis demonstrated high correlations (r 2 = 0.99) for each pair with slopes of 1.00 ± 0.01.
The daily maximum 8-hr average trends across all study days were also highly correlated (r 2 = 0.99) among the three instruments. plots this metric and shows lines representing the current 8-hr O3 standard (75 ppb) and levels representing prospective 8-hr standards of 60 and 70 ppb. Values reported by the three instruments are nearly identical for many days, with data from the model 211 and the model 49C agreeing especially well throughout the study. Agreements of the model 265E chemiluminescence monitor were more variable, especially during the early weeks of the study. During this period the model 265E differed from the other two instruments by several parts per billion, but agreement improved after mid-September.
Differences between the daily maximum 8-hr ozone average reported by the conventional instrument and each new instrument are plotted in . The dates of weekly multipoint calibrations are also noted in the figure. While the daily maximum 8-hr averages range widely (4–94 ppb) in , overall the differences in between the model 49c and the model 211 are small and rarely exceed ±2 ppb; differences between the model 49C and model 265E are similarly clustered near zero after mid-September. Although no cause of the greater model 265E variability early in the study has been identified, differences never exceeded 6 ppb.
Summary results from the 3-month monitoring period are provided in , and the ranked data for all periods when at least one monitor equaled or exceeded 60 ppb are plotted in and . The figures show several periods, all during the first two days of the study, when the model 265E signal seemed anomalously high (noted by arrows). This is during the period when the model 265E experienced higher variability than the other instruments. Accordingly, we have excluded the data from these marked periods in the summary provided in . Examination of the figures shows that the model 49C exceeded the other two instruments during many of the 8-hr averaging periods. As noted in , the average ozone (21–22 ppb) and the peak 8-hr average ozone mixing ratio (93–94 ppb) reported by the three instruments are similar. However, the model 49C reported more rolling 8-hr averages exceeding the current 75-ppb ozone standard (19) than the new technology instruments (16) and also more averages exceeding potential future standards of 70 and 60 ppb. The tendency of the model 49C to report higher ozone averages than the new technology instruments may be due to positive interferences in the conventional UV photometer, as discussed shortly.
Table 4. Summary of rolling 8-hr O3 averages during the study (excluding August 26–27)
Effect of data adjustments on data quality and comparability
Data from the three instruments passed all TCEQ QA and QC acceptance tests during the study period. Because the HRM routinely adjusts their model 49C data for weekly and monthly multipoint calibrations, the other monitor data were adjusted in the same manner. We also used this opportunity to examine how application of the various levels of QC test results can affect the monitoring data. Because small errors or biases have increasing influence on compliance as the standard is tightened, enhanced QC testing and adjustment of monitoring data to improve accuracy may be warranted. Although current practice in the HRM network is to automatically adjust the ozone data for the weekly and monthly calibrations, results from the daily zero/span tests and periodic performance audits are used only to monitor QA/QC acceptance criteria and flag data problems that could trigger corrective action, such as recalibration of the monitor. While these latter tests are not routinely used to adjust the data, we have explored their use for this purpose to assess their impact on data quality and comparability.
examines the effect on several statistical parameters of applying increasingly complex data adjustments to the model 211 data using results from the various levels of QA/QC testing performed during this study. We chose the model 211 for this comparison because it appeared most drift stable throughout the study period. Although audit adjustments had no effect on the number of standard exceedences or the study average O3 mixing ratio (, column 3), it did reduce by 2 ppb the maximum 8-hr average O3. Adjustments using the weekly 3-point and monthly 5-point calibrations lowered study average O3 and reduced the number of 8-hr 60 ppb exceedances but not at the 75 ppb or 70 ppb levels. The effects of daily zero/span adjustments (on the following 24 hourly readings after each daily evening zero/span check) are shown in to increase the number of 75 and 70 ppb exceedances but decrease those at the 60 ppb level. Effects of daily zero/span adjustments on data comparability are explored in and . Differences between the model 49C and model 211 became more negative (), whereas adjustments exacerbate differences between the model 49C and model 265E signals (), especially during the early part of the study.
Table 5. Effect of the application of QA/QC results on model 211 rolling 8-hr average O3 data parameters
Figure 5. Difference in daily maximum 8-hour O3 average between the model 49C and model 211 monitors before and after daily zero/span adjustment.
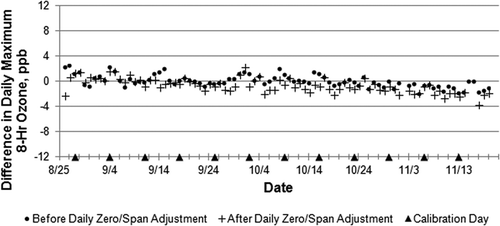
Figure 6. Difference in daily maximum 8-hr O3 average between the model 49C and the model 265E monitors before and after daily zero/span adjustment.
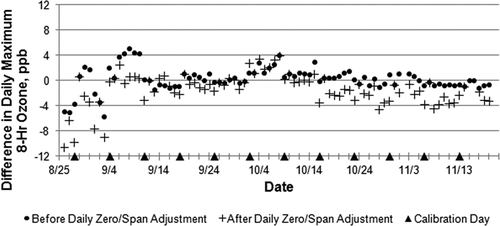
The audit and multipoint calibration adjustments would be expected to improve accuracy, and we found that they also improved data comparability among the three monitors. The case for a daily zero/span adjustment is less clear. The slope for this adjustment is based on a high span point (400 ppb) and actually degraded data comparability. Perhaps lowering span points to values more comparable to current ambient ozone levels (100 ppb) may better clarify benefits of daily drift adjustments.
Possible cause of higher ozone measured by the conventional UV monitor
Compliance with the O3 NAAQS depends on the 3-year mean of annual fourth highest daily maximum 8-hr averages. compares the four highest daily maximum 8-hr O3 averages observed in this study period at the HRM-3 site. In each case, the 8-hr average reported by the model 49C exceeds the model 211 average, and it exceeds the model 265E average on 3 of the 4 days. The fact that the conventional instrument produced generally higher O3 averages during these O3 peak episodes supports a hypothesis of positive interference bias in conventional monitors.
Figure 7. Comparison of maximum daily 8-hr O3 average among three monitors for the four highest O3 days.
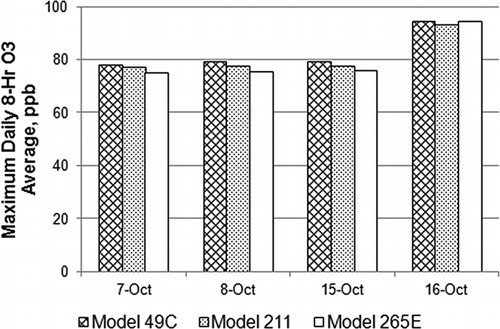
Several causes of positive bias in UV photometers have been reported in the laboratory and field tests cited earlier. Included among the sources of bias are changing water-vapor levels, gas-phase mercury, and a variety of aromatic organic compounds including photochemical oxidation products of the most ubiquitous urban aromatic hydrocarbons in ambient air. Responses of the models 49C, 211, and 265E to a mix of VOCs were examined by spiking zero air containing a low level of ozone. The instruments were zeroed and spanned (100 ppb), the input ozone concentration was set to 30 ppb, and a VOC mixture was continually added to the air stream over a 1-hr period. The mixing ratio of each VOC in the inlet air stream was 66 ppb. The contact time for ozone with these VOCs was short (< 4 sec), so reactive ozone removal was negligible (< 0.03 ppb) for even the most reactive olefin component. shows the responses of the three ozone monitors during this test. The model 49C shows an initial strong positive response to the mixture of VOCs, which declines over the remainder of the hour. The two new-technology instruments are unaffected by the VOC mixture. As noted earlier, many investigators have found that aromatic organic compounds and their photochemically oxygenated and nitrogenated derivatives interfere with UV photometers using conventional scrubbers. Of the 21 hydrocarbons used in this spiking test, styrene is the only one known to cause significant bias in conventional UV photometers, with various reported O3-equivalent responses of 3.7 to 12% (CitationZdanevitch, 2002), 20% (CitationGrosjean and Harrison, 1985), and 113% (CitationKleindienst et al., 1997). The 48-ppb peak response in would correspond to a 73% O3-equivalent response if the entire effect was due to styrene. After the VOC injection was terminated, the model 49C signal went negative, as VOC components absorbed on the scrubber eluted during the following hour. In contrast, the model 211 and model 265E monitors showed no response to the VOC mixture.
Hourly photochemical assessment monitoring site (PAMS) VOC measurements made at the HRM-3 location allowed us to investigate monitored ambient aromatic organic compounds as a possible cause of bias during this study. Hourly difference (model 49c minus model 211) plots and total aromatic hydrocarbon species measured at this site are compared in for October 16–17, 2010, a period that includes the highest hourly O3 value recorded during the study. For much of this period, the model 49C minus model 211 difference was 1 ± 2 ppb; however, during elevated aromatic hydrocarbon periods each morning (2 to 8 a.m) the differences increased to 4 ppb or more. This is consistent with the PAMS mixture spiking test and earlier studies where specific VOCs caused positive interferences in conventional O3 photometers. In both these cases in , October 16–17, 2010, increased difference signals occurred during elevated NO periods that would have titrated ambient O3 to very low levels, confirming a VOC interference rather than an O3 calibration difference between the instruments.
Conclusion
Two new instruments employing advanced O3 monitoring technology were compared with a conventional FEM UV photometry O3 monitor at an industrialized site by the Houston, TX, ship channel. The installation and operation of the new instruments were straightforward. Overall the three instruments were highly correlated (r 2 ≥ 0.99) with regression slopes of 1.00 ± 0.01, and O3 averaged over the study period agreed within 1 ppb. However, ozone standard compliance depends on the fourth highest annual daily maximum 8-hr O3 value, so monitors must measure accurately during these O3 conducive periods. Also, as the value of the ozone standard has been reduced, small errors due to drift or interference can have a greater impact on attainment of the standard.
Many previous studies have demonstrated positive interferences associated with current technology FEM UV O3 monitors, and a test in which a hydrocarbon mixture was continuously diluted into the sample streams of the three instruments during this study yielded a strong positive bias in the conventional UV photometer. The two advanced technology monitors showed no such bias. Consistent with previous observations of positive bias to current technology UV monitors, the conventional model 49C reported the highest values for the four highest 8-hr daily maxima during the test period, with differences between the new and conventional monitors ranging up to 4 ppb. Additional evidence of positive bias was observed during early morning periods with high measured ambient aromatic VOCs, when the difference between the conventional and new technology O3 instruments increased by several parts per billion. While the extent of the positive bias is not large, on the order of a few parts per billion during the study period, the effect is significant: The conventional FEM O3 monitor reported three more exceedances of the current standard than the new instruments, which could clearly play a role in an area being misjudged as “nonattainment.”
Instrument drift can affect the accuracy of ambient O3 data, and the same degree of drift has a proportionally greater effect on the data as the value of the standard is reduced. Adjustment of the raw data for weekly and monthly calibrations, performance audit results, and automated daily zero and span checks was examined to assess the effects of enhanced quality control activities on data quality and comparability. More intense data quality testing and subsequent adjustment may be necessary to achieve the degree of data accuracy required to judge compliance with tighter standards.
Acknowledgment
The authors acknowledge the efforts of the site operators, Bruce Brosch and Mark Halata, and the Houston Regional Monitoring Network for their cooperation in installing and operating the new O3 monitors. They also acknowledge and thank Ken Rozacky (TCEQ) for conducting site audits and the VOC spiking experiment.
References
- Birks , J.W. , Andersen , P.C. and Williford , P.J. 2010 . U.S. patent application publication no. US 2010/0027016 A1 , February 4
- Godet , Y. 1995 . Evaluation of a uv absorption ozone analyzer . Report INERIS-LCSQA. Ref. 737/96 Environnement SA O3 41M and 740/95 Thermo-Environmental Instrument 49C , As cited in Zdanevitch (2002)
- Grosjean , D. and Harrison , J. 1985 . Response of chemiluminescent NOx analyzers and ultraviolet ozone analyzers to organic air pollutants . Environ. Sci. Technol , 19 : 862 doi: 10.1021/es00139a016
- Houston Regional Monitoring Corporation. 2011. Houston air quality trends overview 2010accessed February 23, 2012 http://hrm.radian.com/houston/pdfs/HRM%20Trends%202011%20Final.pdf (http://hrm.radian.com/houston/pdfs/HRM%20Trends%202011%20Final.pdf)
- Hudgens , E.E. , Kliendienst , T.E. , McElroy , F.F. and Ollison , W.M. 1994 . “ A study of interferences in ozone uv and chemiluminescence monitors ” . In Measurement of Toxic and Related Air Pollutants , 405 – 15 . Pittsburgh , PA : Air & Waste Management Association . VIP-39
- Huntzicker , J.A. and Johnson , R.L. 1979 . Investigations of an ambient interference in the measurement of ozone by ultraviolet photometry . Environ. Sci. Technol , 13 : 1414 doi: 10.1021/es60159a005
- Kleindienst , T.E. , McIver , C.D. and Ollison , W.M. A study of interferences in ambient ozone monitors. VIP-74 . Proceedings A&WMA Symposium on Measurement of Toxic and Related Air Pollutants . Pittsburgh , PA . pp. 215 – 25 . Air & Waste Management Association .
- Kleindienst , T.E. , Hudgens , E.E. , Smith , D.F. , McElroy , F.F. and Bufalini , J.J. 1993 . Comparison of chemiluminescence and ultraviolet ozone monitor responses in the presence of humidity and photochemical pollutants . J. Air Waste Manage. Assoc , 43 : 213 – 22 . doi: 10.1080/1073161X.1993.10467128
- Leston , A. and Ollison , W.M. 1992 . “ Estimated accuracy of ozone design values: Are they compromised by method interferences? ” . In Tropospheric Ozone: Nonattainment and Design Value Issues , Vol. 451 , Pittsburgh , PA : Air & Waste Management Association .
- Leston , A.R. and Ollison , W.M. 2000 . “ The impact of ambient aerosols on ozone as measured by ultraviolet photometry. VIP-100/CD ” . In Measurement of Toxic and Related Air Pollutants , Pittsburgh , PA : Air & Waste Management Association .
- Leston , A.R. , Ollison , W.M. , Spicer , C.W. and Satola , J. 2005 . Potential interference bias in ozone standard compliance monitoring . J. Air Waste Manage. Assoc , 55 : 1464 – 72 . doi: 10.1080/10473289.2005.10464749
- Li , Y. , Lee , S.-R. and Wu , C.-Y. 2006 . UV-absorption-based measurements of ozone and mercury: an investigation on their mutual interferences . Aerosol Air Qual. Res , 6 : 418 – 29 .
- Maddy , J.A. A test that identifies ozone monitors prone to anomalous behavior while sampling hot and humid air . Proceedings A&WMA 91st Annual Meeting . Pittsburgh , PA . Air & Waste Management Association . Paper 98-MPB.02P
- Mayer , V.A. , S.A , Arendt S.J , Bailey , eds. 2006 . “ D 5149-02 Standard test method for ozone in the atmosphere: continuous measurement by ethylene chemiluminescence ” . In Annual Book of ASTM Standards , Vol. 11.03 , 430 West Conshohocken , PA : ASTM .
- Meyer , C.P. , Elsworth , C.M. and Galbally , I.E. 1991 . Water vapor interference in the measurement of ozone in ambient air by ultraviolet absorption . Rev. Sci. Instrum , 62 : 223 – 28 . doi: 10.1063/1.1142311
- Obama, B.H. 2011. Statement by the President on the Ozone National Ambient Air Quality Standardsaccessed February 22, 2012 http://www.whitehouse.gov/the-press-office/2011/09/02/statement-president-ozone-national-ambient-air-quality-standards (http://www.whitehouse.gov/the-press-office/2011/09/02/statement-president-ozone-national-ambient-air-quality-standards)
- Spicer , C.W. , Joseph , D.W. and Ollison , W.M. 2010 . A re-examination of ambient air ozone monitor interferences . J. Air Waste Manage. Assoc , 60 : 1353 – 64 . doi: 10.3155/1047-3289.60.11.1353
- Teledyne-API. 2011. Model 265E Chemiluminescence Ozone Analyzer Brochureaccessed November 8, 2011 http://www.teledyne-api.com/pdfs/SAL000036A_265E.pdf (http://www.teledyne-api.com/pdfs/SAL000036A_265E.pdf)
- Texas Commission on Environmental Quality. 2012. HRM-3 Haden Road C603/A114 site informationaccessed February 23, 2012 http://www.tceq.state.tx.us/cgi-bin/compliance/monops/site_photo.pl?cams=603 (http://www.tceq.state.tx.us/cgi-bin/compliance/monops/site_photo.pl?cams=603)
- U.S. Environmental Protection Agency . 1996 . Air quality criteria for ozone and related photochemical oxidants , Vol. 1 , 3 – 97 . Washington , DC : National Center for Environmental Assessment, U.S. EPA. EPA/600/P-93004aF .
- U.S. Environmental Protection Agency . 2008a . National ambient air quality standards for ozone: Final rule . Fed. Reg , 73 : 16436 – 514 .
- U.S. Environmental Protection Agency. 2008b. Environmental technology verification report—JSC Optec 3.02 P-A chemiluminescent ozone analyzer. Februaryaccessed March 8, 2012 http://www.epa.gov/etv/pubs/VR_OptecFeb2008.pdf (http://www.epa.gov/etv/pubs/VR_OptecFeb2008.pdf)
- U.S. Environmental Protection Agency . 2010 . National ambient air quality standards for ozone: Final rule . Fed. Reg , 75 : 2938 – 6052 .
- U.S. Environmental Protection Agency . 2011a . Integrated science assessment for ozone and related photochemical oxidants 3 – 53 . EPA/600/R-10/076B, September
- U.S. Environmental Protection Agency . 2011b . List of designated reference and equivalent methods . Fed. Reg , 76 : 62402
- Wilson , K.L. and Birks , J.W. 2006 . Mechanism and elimination of a water vapor interference in the measurement of ozone by uv absorbance . Environ. Sci. Technol , 40 : 6361 – 67 . doi: 10.1021/es052590c
- Zdanevitch , I. 2002 . “ Study of ozone measurement interferences. Final report DRC-02-39246-AIRE-673-v2-Izd ” . In Verneuil-en-Halatte , France : Institut National de L'Environnement Industriel et des Risques (INERIS) .