Abstract
A pilot hazardous airborne carbonyls study was carried out in Hong Kong and the Mainland of China. Workplace air samples in 14 factories of various types of manufacturing and industrial operations were collected and analyzed for a panel of 21 carbonyl compounds. The factories can be classified into five general categories, including food processing, electroplating, textile dyeing, chemical manufacturer, and petroleum refinery. Formaldehyde was invariably the most abundant carbonyl compound among all the workplace air samples, accounting for 22.0–44.0% of the total measured amount of carbonyls on a molar basis. Acetone was also found to be an abundant carbonyl in workplace settings; among the selected industrial sectors, chemical manufacturers’ workplaces had the highest percentage (an average of 42.6%) of acetone in the total amount of carbonyls measured in air. Benzaldehyde accounted for an average of 20.5% of the total amount of detected carbonyls in electroplating factories, but its contribution was minor in other industrial workplaces. Long-chain aliphatic carbonyls (C6–C10) accounted for a large portion (37.2%) of the total carbonyls in food-processing factories. Glyoxal and methylglyoxal existed at variable levels in the selected workplaces, ranging from 0.2% to 5.5%. The mixing ratio of formaldehyde ranged from 8.6 to 101.2 ppbv in the sampled workplaces. The observed amount of formaldehyde in two paint and wax manufacturers and food-processing factories exceeded the World Health Organization (WHO) air quality guideline of 81.8 ppbv. Carcinogenic risks of chronic exposure to formaldehyde and acetaldehyde by the workers were evaluated. The lifetime cancer hazard risks associated with formaldehyde exposure to male and female workers ranged from 2.01 × 10−5 to 2.37 × 10−4 and 2.68 × 10−5 to 3.16 × 10−4, respectively. Such elevated risk values suggest that the negative health impact of formaldehyde exposure represents a valid concern, and proper actions should be taken to protect workers from such risks.
Many carbonyl species (e.g., formaldehyde, acetaldehyde, and acrolein) are air toxins and they pose public health risks. The scope of this investigation covers 21 types of carbonyls based on samples collected from 14 different workplaces. Findings of the study will not only provide a comprehensive assessment of indoor air quality with regard to workers’ healthy and safety, but also establish a theoretical foundation for future formulation of intervention strategies to reduce occupational carbonyl exposures. No similar study has been carried out either in Hong Kong or the Mainland of China.
Introduction
Carbonyls (aldehydes and ketones) in the air are by-products of the photochemical oxidation of both biogenic and anthropogenic hydrocarbons. They act as photochemical precursors to free radicals and therefore play an important role in ground-level ozone formation (CitationJeffries, 1995). In urban locations, oxidation of fuel combustion contributes the largest portion of carbonyl emissions. Recent reports found that the use of alternate and reformulated automotive fuels could increase the amount of formaldehyde and acetaldehyde emitted in automobile exhaust (CitationKirchstetter et al., 1996; CitationGaffney and Marley, 2000).
Many carbonyl species (e.g., formaldehyde, acetaldehyde, and acrolein) are air toxins and they pose public health risks (CitationNational Research Council, 1980; CitationIARC, 1995; CitationWorld Health Organization [WHO], 2000). The International Agency for Research on Cancer (IARC) classifies formaldehyde as a human carcinogen (CitationIARC, 2006). In 2011, the National Toxicology Program, an interagency program of the Department of Health and Human Services, named formaldehyde as a known human carcinogen in its 12th Report on Carcinogens (CitationNational Toxicology Program, 2011). Acetaldehyde is also evidenced to be carcinogenic to animals (WHO, 2006). Other carbonyls such as acrolein can cause eye irritations, cause odor annoyance, and exacerbate asthma (WHO, 2006; CitationAmerican Conference of Government Industrial Hygienists [ACGIH], 1991; CitationBorchers et al., 1999). Methyl ethyl ketone (MEK), one of the most abundant ketones in the atmosphere, can be potentially oxidized to a neurotoxic metabolite (CitationTabershaw et al., 1977).
On average, an individual spends approximately 90 percent or more of their time indoors (CitationU.S. Environmental Protection Agency [EPA], 2009). Satisfactory indoor air quality is crucial to protecting general public health, though carbonyl levels in indoor air have been reported to be much higher than those of outdoor air, indicating that local carbonyl emissions originate predominantly from indoor sources (CitationZhang et al., 1994; CitationLiu et al., 2006). In light of the growing awareness of occupational health and safety, evaluation of indoor carbonyl levels in confined spaces in various workplaces and residential environments becomes increasingly imperative. Common objects such as furniture, carpets, fabrics, paints, and cooking fumes, as well as environmental tobacco smoke (ETS), have been reported as indoor sources of carbonyls. Other indoor carbonyl sources are identified in certain industrial settings where carbonyls are used as raw materials or are released during the manufacturing process. For example, formaldehyde is used in the production of resins, polyurethane, polyester plastics, resin coating, synthetic lubricating oils, and plasticizers. In agriculture, formaldehyde is used as a fumigant and as a preservative in animal feed. Acetaldehyde also has many industrial uses, such as in alkyd resin production, and it is also a by-product from the production of acetic acid.
In practice, carbonyl-level measurements usually focus on formaldehyde, acetone, and acetaldehyde only. In the present study, the scope of investigation is expanded to include 21 types of carbonyls based on samples collected from 14 different workplaces. Findings of the study will not only provide a comprehensive assessment of indoor air quality with regard to workers’ healthy and safety, but also establish a theoretical foundation for future formulation of intervention strategies to reduce occupational carbonyl exposures. No similar study has been carried out in Hong Kong or Mainland of China.
Experimental Section
Sample collection
Indoor carbonyl levels were measured in 14 workplaces; these workplaces were selected from different factories of various operation types and scales, and can be classified into five general categories: (1) food processing, (2) electroplating, (3) textile dyeing, (4) chemical manufacturer, and (5) petroleum refinery. lists the general information and characteristics of each sampled workplace. There were two Chinese canned food and two Chinese snack manufacturers in the food processing category, two electroplating factories of equivalent sizes in the electroplating category, three factories of different sizes in the textile dyeing category, three manufacturers in the chemical manufacturer category, and two medium-scale manufacturers of wax and lubricant in the petroleum refinery category.
Table 1. Summary of sampled industrial workplaces
Air samples were collected into silica cartridges impregnated with acidified 2,4-dinitrophenylhydrazine (DNPH) (Sep-Pak DNPH-silica, 55–105 μm particle size, 125 Å pore size, manufactured by Waters Corporation, Milford, MA) at a flow rate of 0.7 L/min. Sampling was carried out during normal industrial operating hours, with each sample being collected for 240 min. The selected sampling time ensures that the collected carbonyls would not consume >30% of the derivatizating agent coated on the cartridge. In total, six visits were made for each factory in summer (June 2011–August 2011) and winter (December 2011–February 2012), respectively, and five (for Category 3 and 4) and six (for Category 1, 2 and 5) samples were collected during each visit. The samples were collected in the workplaces where major product manufacturing and machine operations were taking place. lists detailed descriptions of the workplaces under the five factory categories. The number of workers only refers to the workers who were working in the sampling collection rooms and does not include any employees in other separated areas, offices, and pantries. In normal cases, working hours and frequency for each worker are 9 hours per day and 5 days per week, respectively. Baseline sampling was conducted in each factory during the off-duty period. No breakthrough was acknowledged at such sampling flow rates and sampling times (CitationHerrington et al., 2007; CitationU.S. EPA 1999; Waters, 2007). The flow rates were checked in the field in the beginning and at the end of each sampling period using a calibrated flowmeter (Gilibrator Calibrator manufactured by Gilian Instruments, West Caldwell, NJ). A Teflon filter assembly (manufactured by Whatman, Clifton, NJ) and an ozone scrubber (Sep-Pak manufactured by Waters Corporation) were installed in front of the DNPH-silica cartridge in order to remove any particulate matter and to prevent possible ozone interference, respectively (CitationSpaulding et al., 1999). The recovery of carbonyls collected in the process should not be affected by the ozone trap (CitationHo and Yu, 2002). Collocated samples were collected to determine sample collection reproducibility, which was found to be >95% in the field. A cartridge was designated as a field blank on each sampling trip and was handled the same way as the sample cartridges. The amounts of carbonyls detected in the cartridges were corrected for the field blank when air concentrations of carbonyls were computed. Three baseline samples were collected during nonoperation hours on each site as well. The DNPH-coated cartridges were stored in a refrigerator at <4°C after sampling and before analysis, and were analyzed in our laboratory within 2 weeks. Temperature and relative humidity (RH) were recorded during the sampling period.
Sample analysis
In total, 21 carbonyls including mono- and dicarbonyls were quantified (). Unsaturated carbonyls including acrolein and crotonaldehyde were detected but their abundances were not reported in the study; the unsaturated carbonyl DNP-hydrazones could react with excess reagent to form adducts, which could not be quantified accurately due to chromatographic and response factor issues (CitationHo et al., 2011; CitationSchulte-Ladbeck et al., 2001).
Table 2. Limit of detection (LOD) for the target carbonyls
Each DNPH-coated cartridge was eluted with 2.0 mL of acetone-free acetonitrile (high-performance liquid chromatography [HPLC] grade, manufactured by Mallinckrodt Laboratory Chemicals, Phillipsburg, NJ) to a volumetric flask. Testing has been done to demonstrate that no DNPH and its derivatives remained in the cartridge after the 2.0-mL elution (CitationHo et al., 2007). Certified calibration standards of monocarbonyl DNP-hydrazones were purchased from Supelco in Bellefonte, PA, and diluted into concentration ranges of 0.015–3.0 mg/mL. Calibration standards of dicarbonyls were prepared by mixing glyoxal and methylglyoxal (manufactured by Sigma in St. Louis, MO) in acetonitrile with 1 mL of 100 μg/mL DNPH in an acidic aqueous solution. The mixtures were allowed to stand at room temperature for at least 6 hr for complete derivatization. The final volume of each calibration mixture was made to be 2.0 mL with 8:2 (volume/volume) of acetonitrile/pyridine (HPLC grade, manufactured by Sigma). Concentrations of the dicarbonyl DNP-hydrazones in the calibration standards ranged from 0.01 to 2.0 μg/mL. Linearity was indicated by a correlation of determination (r2 ) value of at least 0.999. The cartridge extracts and calibration standards were analyzed by injecting 20 μL of the samples into a high-pressure liquid chromatography (HPLC) system (series 1200 manufactured by Agilent Technology, Santa Clara, CA) equipped with a photodiode array detector (DAD). The column for separation was a 4.6 × 250-mm Spheri-5 ODS 5μm C-18 reverse-phase column (manufactured by PerkinElmer, Norwalk, CT) operated at room temperature. The mobile phase consisted of three solvent mixtures: (A) 6:3:1 (v/v) water, acetonitrile, and tetrahydrofuran, respectively; (B) 4:6 (v/v) water and acetonitrile, respectively; and (C) acetonitrile. The gradient program was 80% A/20% B for 1 min, followed by linear gradients to 50% A/50% B for 8 min, 100% B for 10 min, 100% C for 6 min, and finally 100% C for 5 min. The flow rate was 2.0 mL/min throughout the run. The absorbances at 360 nm, 390 nm, and 420 nm were used for identification of the aliphatic carbonyls, aromatic carbonyls (benzaldehyde, tolualdehydes, and 2,5-dimethylbenzaldehyde), and dicarbonyls, respectively. The limit of detection (LOD) was obtained by analyzing a minimum of seven replicates of a standard solution containing the analytes at a concentration of 0.015 μg/mL, and is estimated using the following equation:
Health risk calculation
The carcinogenic risks of chronic exposure to carbonyls were assessed in this study. The risk estimation with a cancer endpoint is expressed in terms of the probability of developing cancer from a lifetime of continuous exposure to carbonyl. Chronic daily intake (CDI) of a carcinogenic contaminant was controlled by various factors, such as exposure frequency, exposure duration, and the body weight of the receptor. The equation used to calculate CDI in mg/kg/day is
The lifetime cancer hazard risk (R) is calculated using the equation
Results and Discussion
Carbonyl compositions
The concentrations of carbonyls in the sampled workplaces varied widely depending on individual carbonyl types, factory sizes, manufacturing raw materials and products, and ventilation systems (refer to ). During the sampling periods, workplaces within the same factory categories used identical raw materials and manufactured identical products. For each factory, samples were respectively collected in summer and winter. No obvious diurnal or seasonal variations in either absolute values or molar compositions were observed for the samples. The concentrations of the carbonyls were statistically the same by Student's t-test with a 95% confidence level for the samples collected in different periods at the same sampling location. The average value was thus taken to compare individual carbonyls that existed in different factories. Elevated levels of formaldehyde, acetaldehyde, acetone, and nonaldehyde were observed. compares the baseline and measured concentration levels of carbonyls in each workplace. A maximum of five abundant carbonyls (i.e., formaldehyde, acetaldehyde, acetone, butyraldehyde, and nonaldehyde) can be detected in the baseline. The results indicate that a substantial increase of carbonyl concentrations was directly related to factory operation. In certain factories such as those of food processing and chemical manufacturers, the baseline levels of formaldehyde and acetone were relatively high (>10 ppbv) even when no industrial activity was taking place. Poor ventilation systems cannot efficiently remove or dilute indoor polluted air. In addition, secondhand emission from factory walls or other absorbable surfaces can retain high baseline levels of these carbonyls (CitationKatsumata et al., 2008). Carbonyl molar composition profiles are plotted in for comparison of the relative abundance of different carbonyls in the various categories of factories. Mean values are plotted because more than one factory of the same category were available.
Table 3. Carbonyl mixing ratios (ppbv) in the sampled industrial workplaces
Figure 1. Comparison of carbonyl mixing ratios in various industrial environments during (a) operating hours and (b) baseline.
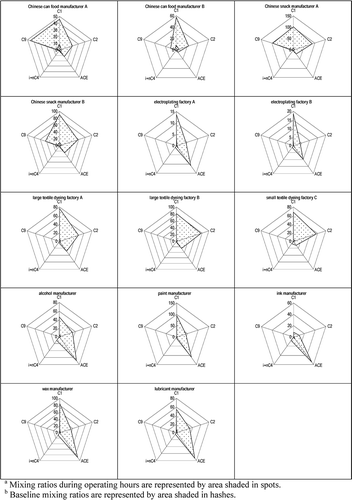
Formaldehyde was invariably an abundant carbonyl in indoor air of all the sampled factories and accounted for 22.0–44.0% of the total quantified carbonyls. It was the largest carbonyl contributor in all industries except chemical manufacture and petroleum refinery. In other industry sectors, formaldehyde was the second most abundant carbonyl after acetone. The highest contribution of formaldehyde was seen in the textile dyeing industry, which often uses formaldehyde-based resins as finishers to make fabrics crease-resistant (CitationNational Industrial Chemicals Notification and Assessment Scheme [NICNAS], 2007). In other chemical-related industries, formaldehyde is also a precursor to polyfunctional alcohols (e.g., pentaerythritol) and a starter to its derivatives, which are important components in paints and solvents (CitationThe Campaign for Safe Cosmetics, 2012). Elevated levels of formaldehyde were also found in food-processing factories samples, contradicting the findings from a previous report that formaldehyde had the highest estimated annual emission rate (222 kg/yr) in Hong Kong related to commercial cooking activities (CitationHo et al., 2006).
Acetone was detected in abundance in most of the factories, ranging from 9.8 to 109.6 ppbv. The average contribution of acetone to total carbonyls was 5.2% for food processing factories but was up to 42.6% on average for chemical manufacturers, indicating its strong impact in applications and productions of chemicals. Acetone serves as a common solvent or its intermediate in various industrial processing (CitationWHO, 1998; CitationHuang et al., 2007), and it was found to be the largest contributor of carbonyls in chemical manufacture and petroleum refinery industries. Among the sampled workplaces, acetone accounted for >50% of total carbonyls detected in the ink manufacturing factory as it is one of the essential components in writing ink; elevated levels of acetone (39.3%) were also seen for the paint manufacturer as acetone is also used as a volatile component of certain paints and varnishes. In addition, acetone accounted for an average of 19.0% of total carbonyls found in electroplating factories. Acetone is very effective in dissolving various types of greases and waxes; thus, it is often used as a cleaner before electroplating and as a polisher after the electroplating process to remove any marking trail on the end product (CitationKim et al., 2004).
Acetaldehyde was typically the second or third most abundant carbonyl after formaldehyde and acetone. The relative contribution of acetaldehyde ranged from 8.0 to 34.6%. The highest level of acetaldehyde contribution was seen in the textile dyeing factories. Acetaldehyde is used in production of polyester resins and basic dyes, which are found commonly in clothes, surfactants, and washing reagents (CitationU.S. EPA, 1998). There is a large daily consumption of dyes in the textile industry. In the sampled factories, the use of resins and dyes ranged from 10 to 35 kg per day during the sampling periods. High contributions of acetaldehyde were also seen in food-processing factories, accounting for 8.7 to 17.2% of the total quantified carbonyls. In the food-processing industry, acetaldehyde is used as fruit and fish preservative, flavoring agent, and denaturant for alcohol (CitationSittig, 1985).
The most abundant aromatic carbonyl among the samples was benzaldehyde. The highest level of benzaldehyde contribution was seen in electroplating factories, falling in the range of 16.6 to 24.4%. The electroplating industry has great demand for benzaldehyde (CitationSurvila et al., 2009; CitationAli Eltoum et al., 2011). A tin, lead, or tin–lead alloy electroplating bath comprises typically benzaldehyde and benzaldehyde derivative. Benzaldehyde is also a raw material used in many industrial sectors, such as in polymerization and manufacturing of odorants and flavors (CitationWilkinson et al., 1979; CitationWolken et al., 2004). The contribution of benzaldehyde in the samples collected in textile dyeing factories was approximately 1.0%. High concentrations of benzaldehyde are often detected in textile dyeing wastewater (CitationRajkumar et al., 2004).
The concentrations of long-chain aliphatic aldehydes (i.e., C6–C10) were comparatively high in food processing factories. The relative contribution of the sum of C6–C10 aldehydes to the total carbonyls was 37.2%, much larger than the value (0.5–2.9%) for the other categories of factories (). It is noted that nonaldehyde (C9) was the most abundant carbonyl besides formaldehyde and acetaldehyde in food-processing factories. Nonaldehyde likely arises from the breakdown of oleic acid, which is thermally decomposed from cooking oil (CitationSchauer et al., 2000). In the sampled food processing factories, a huge daily consumption of cooking oil was required for production of Chinese canned foods and snacks. In addition, electricity was used as a fuel but produces zero carbonyl emissions. As a result, carbonyls were primarily derived from the heated cooking materials (i.e., oil and food). Current results are consistent with a previous report that nonaldehyde was found to be the most abundant carbonyl in the exhaust system of commercial restaurants, where a large amount of heated oil was consumed in the cooking process (CitationHo et al., 2006).
Dicarbonyls were detected in most of the factories. Glyoxal and methylglyoxal account for 0.1–3.4% and 0.1–3.2%, respectively, of the total quantified carbonyls. Their relative contributions were higher (>2%) in the food-processing, electroplating, and petroleum-refinery industries than in the other industry categories (<0.7%). The two smallest dicarbonyls are potential precursors leading to secondary organic aerosol formation (CitationFu et al., 2008). They are emitted from incomplete combustion processes and are formed in important yields from many common anthropogenic and biogenic hydrocarbons (CitationAltshuller, 1993; CitationKawamura et al., 2000; CitationVolkamer et al., 2005). Elevated levels in the workplace suggested that industrial processes were emission sources of glyoxal and methylglyoxal.
Tolualdehydes (including o-, m-, and p-isomers) were generally detected only in the electroplating industry (<0.5 ppbv) and petroleum refinery (<3.5 ppbv). The relative contribution of all tolualdehyde isomers to the total amount of detected carbonyls was <2.0%. An exception was the trace level of m- (0.22 ppbv) and p-tolualdehydes (0.12 ppbv) detected for Chinese snack manufacturer A. The results indicated that the sampled industrial processes were not major contributors of tolualdehydes.
The contribution of MEK to total carbonyls ranged from 1.2 to 7.0% among the samples. The highest contribution was observed for the ink manufacturer, where MEK is an effective and common solvent (CitationTurner and McCreery, 1981) and is used in processes involving gums, resins, cellulose acetate and nitrocellulose coatings, and vinyl films (CitationApps, 1958). A large contribution of 1.7–3.3% was detected for MEK in food processing factories; cooking activity was shown to be one of many emission sources for MEK (CitationU.S. EPA, 1994).
For propionaldehyde, large contributions were observed in alcohol and ink manufacturers, accounting for 9.2% and 5.5%, respectively, of total detected carbonyls. Propionaldehyde is used in the manufacturing of plastics, in the synthesis of rubber chemicals, and as a disinfectant and preservative; thus, it is largely released from chemical manufacturing facilities (CitationU.S. Department of Health and Human Services, 2011). The contributions of n-butyraldehyde were also relatively high (>5.0%) in these two chemical manufacturers. n-Butyraldehyde is used as an intermediate in the production of synthetic resins, rubber accelerators, solvents, plasticizers, and high-molecular-weight polymers (CitationGrayson and Eckroth, 1985; CitationIrving and Lewis 1987; CitationWindholz et al., 1983). No any contributions were observed for iso-butyraldehyde, n-valeraldehyde, and iso-valeraldehyde in the samples. Besides, acrolein is a major carbonyl in cooking (CitationSchauer et al., 2000; CitationHo et al., 2006) or from the burning of fuels such as gasoline or oil (CitationAgency for Toxic Substances and Disease Registry [ATSDR], 2007). In this study, acrolein and crotonaldehyde measurements could not be reported due to limitations of the DNPH-coated solid sorbent cartridge method (CitationHo et al., 2011). Hence, their contributions in the workplaces were not deducible.
Guideline comparison
Formaldehyde was the most abundant carbonyl compound emitted from industrial activities. The World Health Organization (WHO) guideline for indoor formaldehyde is a 30-min average of 81.8 ppbv, or 100 μg/m3 (CitationWHO, 2010). The Japanese National Institute of Health Sciences (JNIH) and the China Indoor Air Quality Center (CIAQC) set the same indoor air quality guidelines as WHO for formaldehyde. The average formaldehyde levels in the Chinese snack manufacturers A and B, paint manufacturer, and wax manufacturer surpassed the WHO guideline. compares the observed carbonyl levels with those international guidelines for indoor air quality. The highest formaldehyde level was detected for the Chinese snack manufacturer A and it exceeded the WHO guideline by 2 times. The National Institute of Occupational Safety and Health (NIOSH) recommended exposure limit (REL) for formaldehyde for an 8- or 10-hr time-weighted average (TWA) exposure and/or ceiling is 16.3 ppbv (20 μg/m3). The average mixing ratios of formaldehyde in most industrial factories exceeded this exposure limit except in electroplating factory A, where the ratio was approximately 20% below the limit. The Office of Environmental Health Hazard Assessment (OEHHA) sets the chronic toxicity of inhalation reference exposure level for formaldehyde at 2.5 ppbv (3 μg/m3). The critical effects of formaldehyde exposure include upper and lower airway irritation and eye irritation in humans, and degenerative, inflammatory, and hyperplastic changes of the nasal mucosa in humans and animals. Formaldehyde levels measured during both the operating period and off-duty period surpassed OEHHA's exposure level, indicating a substantial risk of chronic toxicity among workers conducting industrial activities in these settings.
Figure 4. Comparison of carbonyl molar compositions in workplaces against international health guidelines.
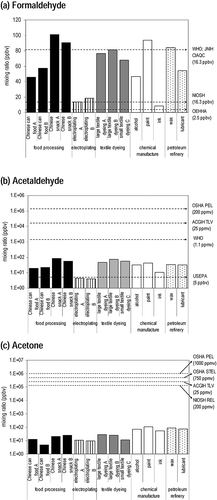
Acetaldehyde was the next abundant carbonyl species in the total carbonyl mixing ratio. Acetaldehyde has been classified as a probable human carcinogen (Group 2B) by the U.S. EPA and a possible carcinogenic to humans (Group 2B) by IARC. For acetaldehyde, the American Conference of Governmental Industrial Hygienists (ACGIH) threshold limit value (TLV) ceiling is set at 25 parts per million by volume (ppmv), which is equivalent to 45 mg/m3; the Occupational Safety and Health Administration (OSHA) sets its permissible exposure limit (PEL) for general industry at 200 ppmv (360 mg/m3) for every 8-hr TWA. The mixing ratios for acetaldehyde measured in this study were at least two magnitudes below the established exposure limits and the 24-hr average tolerable concentration of 1.11 ppmv (2000 μg/m3) set by WHO. However, with the exception of the electroplating industry, acetaldehyde levels for the remaining four industry categories were found to exceed the reference concentration (5 ppbv or 9 μg/m3) proposed by the U.S. EPA, which is defined as the daily inhalational exposure likely to be without an appreciable risk of deleterious non-cancer effects during a lifetime.
Large consumption and production of organic solvents and their usage as an intermediate in the industry result in acetone becoming an abundant carbonyl in workplace settings. The health effects of acetone have been studied extensively and the compound is generally classified as having low acute and chronic toxicity if ingested and/or breathed. It is not currently regarded as a carcinogen, a mutagenic chemical, or a concern for chronic neurotoxicity effects. ACGIH TLV and NIOSH REL for acetone is 500 ppmv (1,187 mg/m3) and 250 ppmv (590 mg/m3), respectively, for every 8-hr TWA. In addition, the OSHA PEL for acetone for general industries is 1,000 ppmv (2,400 mg/m3) for every 8-hr TWA and 750 ppmv for short-term exposure limit (STEL-15 min); acetone concentrations in the five sampled industrial categories were far below the exposure limits. Nonaldehyde, benzaldehyde, glyoxal, and methylglyoxal were other carbonyl species from industrial processing. Even though the substance potentially irritates the eyes, the skin, and the respiratory tract, no occupational exposure limits have been established for these compounds.
Health risk assessment
Potential health risks for formaldehyde and acetaldehyde exposures were examined owing to their abundances in the industrial workplaces and their carcinogenicity statuses classified by the CitationU.S. EPA (2012). Inhalation exposure is frequently associated with exposure frequency, duration, and activity pattern; these elements are essential factors in the calculation of chronic daily intake and lifetime cancer hazard risk. Several assumptions have been made based on suggestions by the U.S. EPA in regard to relative carcinogenic assessment. The volume of air inspired by a typical heavy-duty worker is assumed to be 38.8 m3/day and 23.5 m3/day for men and women, respectively, while the absorption factor of carbonyl is estimated at 90%. It has also been assumed that a worker spends 9 hours per day and 5 days per week during 40 working years. An average body weight of 70 kg (male) and 60 kg (female) and an average lifetime of 70 yr for both genders are assumed (CitationU.S. EPA, 1994). The PF values for formaldehyde and acetaldehyde are 0.045 and 0.0077 kg/day/mg, respectively. The estimated chronic daily intake and lifetime cancer hazard risk for formaldehyde and acetaldehyde are summarized in . The lifetime cancer hazard risks associated with formaldehyde ranged from 2.01 × 10−5 to 2.37 × 10−4 and 2.68 × 10−5 to 3.16 × 10−4 for male and female workers, respectively. For acetaldehyde, the risks were 2.41 × 10−6 to 5.13 × 10−5 and 3.12 × 10−6 to 6.84 × 10−5 for male and female workers, respectively. Risk value below 1 in a million (<1 × 10−6) is typically considered below the level of concern while a risk value above 100 in a million (>1 × 10−4) signifies an immediate need to initiate interventions to protect human health (CitationLee et al., 2006). Obviously, formaldehyde poses a higher cancer risk to the workers compared to acetaldehyde. The lifetime cancer hazard risks associated with acetaldehyde in all the industrial workplaces were well below 1 × 10−4. However, for formaldehyde, the values were above the risk levels in most of the factories except electroplating factories A and B and small textile dyeing factory C. The results demonstrate that formaldehyde exposure in the workplace is a valid occupational health and safety concern, and proper actions should be taken promptly to protect the workers.
Table 4. Chronic daily intake (CDI) and lifetime cancer hazard risk (R) for formaldehyde and acetaldehyde in the industrial workplaces
Summary
In this study, gaseous carbonyl levels were measured in 14 different factories of various sizes and industrial types. High levels of formaldehyde and acetaldehyde have been detected in two food-processing factories and wax and paint manufacturers. Findings from this study have established the theoretical foundation for further investigation into whether a properly designed and installed ventilation system would prevent the accumulation of carbonyls in workplaces. Thorough identification and profiling of gaseous carbonyls in workplace air would provide insight to their potential sources and emission strength in relations to industrial activities. The study results also call attention to the imminent need to reevaluate the implementation of indoor air quality standards; effective implementation of such standards is necessary to protect the health of workers by reducing occupational exposure to harmful chemicals in the workplace.
Acknowledgment
This study is in part supported by projects from the Hong Kong Premium Services and Research Laboratory (EP-012009-G and EP032009-H) and the Chinese Academy of Sciences (KZCX2-YW-BR-10 and KZCX2-YW-148).
References
- Agency for Toxic Substances and Disease Registry . 2007 . Toxicological Profile for Acrolein , Atlanta , GA : US Department of Health and Human Services, Public Health Service .
- Ali Eltoum , M.S. , Baraka , A.M. and Hassan , E.A. 2011 . Electrodeposition and characterization of nickel from gluconate baths in presence of some additives . J. Am. Sci , 7 ( 5 ) : 368 – 77 .
- Altshuller , A.P . 1993 . Natural volatile organic substances and their effect on air quality of the United States . Atmos. Environ , 17 : 2131 – 65 .
- American Conference of Government Industrial Hygienists . 1991 . Documentation of the Threshold Limit Values and Biological Exposure Indices , Cincinnati , OH : ACGIH .
- Apps , E.A. 1958 . Printing Ink Technology , London , , UK : Leonard Hill Limited .
- Borchers , M.T. , Carty , M.P. and Leikauf , G.D. 1999 . Regulation of human airway mucins by acrolein and inflammatory mediators . Am. J. Physiol , 276 : 549 – 55 . doi: 10.1021/es061247k
- Fu , T. , Jacob , D.J. , Wittrock , F. , Burrows , J.P. , Vrekoussis , M. and Henze , D.K. 2008 . Global budgets of atmospheric glyoxal and methylglyoxal, and implications for formation of secondary organic aerosols . J. Geophys. Res , 113 : D15303 doi: 10.1029/2007JD009505
- Gaffney , J.S. and Marley , N.A. 2000 . “ Alternative fuels ” . In Air Pollution Reviews—Volume 1: The Urban Air Atmosphere and Its Effects , Edited by: Brimblecombe , P. and Maynard , R.L. London , , UK : Imperial College Press .
- Grayson , M. and Eckroth , D. 1985 . Kirk–Othmer Concise Encyclopedia of Chemical Technology , New York, NY : Wiley-Interscience .
- Herrington , J.S. , Fan , Z.-H. , Lioy , P.J. and Zhang , J. 2007 . Low acetaldehyde collection efficiencies for 24-hour sampling with 2,4-dinitrophenylhydrazine (DNPH)-coated solid sorbents . Environ. Sci. Technol , 41 : 580 – 85 . doi: 10.1021/es061247k
- Ho , K.F. , Ho , S.S.H. , Cheng , Y. , Lee , S.C. and Yu , J.Z. 2007 . Real-world emission factors of fifteen carbonyl compounds measured in a Hong Kong tunnel . Atmos. Environ , 41 ( 8 ) : 1747 – 58 . doi: 10.1016/j.atmosenv.2006.10.027
- Ho , S.S.H. and Yu , J.Z. 2002 . Concentrations of formaldehyde and other carbonyls in environments affected by incense burning . J. Environ. Monit , 4 ( 5 ) : 728 – 33 . doi: 10.1039/b200998f
- Ho , S.S.H. , Yu , J.Z. , Chu , K.W. and Yeung , L.L. 2006 . Carbonyl emissions from commercial cooking sources in Hong Kong . J. Air Waste Manag. Assoc , 56 ( 8 ) : 1091 – 98 . doi: 10.1080/10473289.2006.10464532
- Ho , S.S.H. , Ho , K.F. , Liu , W.D. , Lee , S.C. , Dai , W.T. , Cao , J.J. and Ip , H.S.S. 2011 . Unsuitability of using the DNPH-coated solid sorbent cartridge for determination of airborne unsaturated carbonyls . Atmos. Environ , 45 ( 1 ) : 261 – 65 . doi: 10.1016/j.atmosenv.2010.09.042
- Huang , X.H.H. , Ip , H.S.S. and Yu , J.Z. 2007 . Determination of trace amounts of formaldehyde in acetone . Anal. Chim. Acta , 604 : 134 – 38 . doi: 10.1016/j.aca.2007.10.011
- International Agency for Research on Cancer . 1995 . IARC Monographs on the Evaluation of Carcinogenic Risks to Humans—Volume 62: Wood Dust and Formaldehyde , Lyon , , France : IARC .
- International Agency for Research on Cancer . 2006 . IARC Monographs on the Evaluation of Carcinogenic Risks to Humans—Volume 88: Formaldehyde, 2-Butoxyethanol and 1-tert-Butoxypropan-2-ol , Lyon , , France : IARC .
- Irving , N. and Lewis , R.J. 1987 . Hawley's Condensed Chemical Dictionary , New York, NY : Van Nostrand Reinhold .
- Jeffries , H.E. 1995 . “ Photochemical air pollution ” . In Composition, Chemistry, and Climate of the Atmosphere , Edited by: Singh , H.B. New York, NY : Van Nostrand Reinhold .
- Katsumata , H. , Murakami , S. , Kato , S. , Hoshino , K. and Ataka , Y. 2008 . Measurement of semivolatile organic compounds emitted from various types of indoor materials by thermal desorption test chamber method . Build. Environ , 43 ( 3 ) : 378 – 83 . doi: 10.1016/j.buildenv.2006.03.027
- Kawamura , K. , Steinberg , S. and Kaplan , I.R. 2000 . Homologous series of C1–C10 monocarboxylic acids and C1–C6 carbonyls in Los Angeles air and motor vehicle exhausts . Atmos. Environ , 34 : 4175 – 91 . doi: 10.1016/S1352-2310(00)00212-0
- Kim , J.Y. , Yu , J. , Lee , J.H. and Lee , T.Y. 2004 . The effects of electroplating parameters on the composition and morphology of Sn–Ag solder . J. Electron. Mater , 33 ( 12 ) : 1459 – 64 . doi: 10.1007/s11664-004-0087-9
- Kirchstetter , T.W. , Singer , B.C. , Harley , R.A. , Kendall , G.R. and Chan , W. 1996 . Impact of oxygenated gasoline use on California light-duty vehicle emissions . Environ. Sci. Technol , 30 ( 2 ) : 661 – 670 . doi: 10.1021/es950406p
- Lee , C.W. , Dai , Y.T. , Chien , C.H. and Hsu , D.J. 2006 . Characteristics and health impacts of volatile organic compounds in photocopy centers . Environ. Res , 100 ( 2 ) : 139 – 49 . doi: 10.1016/j.envres.2005.05.003
- Liu , W. , Zhang , J. , Zhang , L. , Turpin , B. , Weisel , C. , Morandi , M. , Stock , T. , Colome , S. and Korn , L. 2006 . Estimating contributions of indoor and outdoor sources to indoor carbonyl concentrations in three urban areas of the United States . Atmos. Environ , 40 ( 12 ) : 2202 – 14 . doi: 10.1016/j.atmosenv.2005.12.005
- National Industrial Chemicals Notification and Assessment Scheme . 2007 . NICNAS Existing Chemicals Information Sheet—Formaldehyde in Clothing and Other Textiles , Sydney : Australian Government Department of Health and Ageing .
- National Research Council . 1980 . Formaldehyde—An Assessment of Its Health Effects , Washington , DC : National Academies Press .
- National Toxicology Program . 2011 . Report on Carcinogens , Research Triangle Park , NC : U.S. Department of Health and Human Services, Public Health Service, National Toxicology Program .
- Rajkumar , D. , Song , B. and Kim , J. 2007 . Electrochemical degradation of Reactive Blue 19 in chloride medium for the treatment of textile dyeing wastewater with identification of intermediate compounds . Dyes Pigments , 72 ( 1 ) : 1 – 7 . doi: 10.1016/j.dyepig.2005.07.015
- Schauer , J.J. , Kleeman , M.J. , Cass , G.R. and Simoneit , B.R.T. 2000 . Measurement of emissions from air pollution sources. 4. C1–C27 organic compounds from cooking with seed oils . Environ. Sci. Technol , 36 ( 4 ) : 567 – 75 . doi: 10.1021/es002053m
- Schulte-Ladbeck , R. , Lindahl , R. , Levin , J.-O. and Karst , U. 2001 . Characterization of chemical interferences in the determination of unsaturated aldehydes using aromatic hydrazine reagents and liquid chromatography . J. Environ. Monit , 3 : 306 – 10 . doi: 10.1039/b101354h
- Sittig , M. 1985 . Handbook of Toxic and Hazardous Chemicals and Carcinogens , Park Ridge , NJ : Noyes .
- Spaulding , R.S. , Frazey , P. , Rao , X. and Charles , M.J. 1999 . Measurement of hydroxy carbonyls and other carbonyls in ambient air using pentafluorobenzyl alcohol as a chemical ionization reagent . Anal. Chem , 71 ( 16 ) : 3420 – 27 . doi: 10.1021/ac9900432
- Survila , A. , Mockus , Z. , Kanapeckaitė , S. , Samulevičienė , M. and Jasulaitenė , V. 2009 . Electroplating tin from acidic sulfate baths containing benzaldehyde . Prot. Met. Phys. Chem. Surf , 45 ( 4 ) : 455 – 60 . doi: 10.1134/S2070205109040145
- Tabershaw , I.R. , Utidjian , H.M.D. and Kawahara , B.L. 1977 . “ Chemical hazards ” . In Occupational Diseases: A Guild to Their Recognition , Edited by: Key , M.M. , Henschel , A.F. , Butler , J. , Ligo , R.N. and Tabershaw , I.R. Washington , DC : U.S. Department of Health, Education, and Welfare .
- The Campaign for Safe Cosmetics, 2012. No more toxic tub: Getting contaminants out of children's bath & personal care productsaccessed May 19, 2012 http://safecosmetics.org/article.php?id=414 (http://safecosmetics.org/article.php?id=414)
- Turner , C.F. and McCreery , J.W. 1981 . The Chemistry of Fire and Hazardous Materials , New York, NY : Allyn and Bacon .
- U.S. Department of Health and Human Services . 2011 . Hazardous Substances Data Bank (HSDB)—Comprehensive, Peer-Reviewed Toxicology Data for About 5,000 Chemicals , Bethesda , MD : U.S. National Library of Medicine .
- U.S. Environmental Protection Agency . 1998 . Health Assessment Document for Acetaldehyde , Washington , DC : U.S. EPA, Office of Research and Development, Office of Health and Environmental Assessment .
- U.S. Environmental Protection Agency . 2009 . The Inside Story: A Guide to Air Quality , Washington , DC : U.S. EPA/Office of Air and Radiation, Office of Radiation and Indoor Air (6609J), cosponsored with the Consumer Product Safety Commission .
- U.S. Environmental Protection Agency . 1994 . Locating and Estimating Air Emission From Sources of Methyl Ethyl Ketone , Research Triangle Park , NC : Office of Air Quality Planning and Standards Research .
- U.S. Environmental Protection Agency . 1994 . Methods for Derivation of Inhalation Reference Concentrations (RfCs) and Application of Inhalation Dosimetry , Washington , DC : U.S. EPA, Office of Research and Development, Office of Health and Environmental Assessment .
- U.S. Environmental Protection Agency . 1999 . Compendium Method TO-11A: Determination of Formaldehyde in Ambient Air Using Adsorbent Cartridge Followed by High Performance Liquid Chromatography , Cincinnati , OH : U.S. EPA, Center for Environmental Research Information, Office of Research and Development .
- U.S. Environmental Protection Agency. 2012. Integrated Risk Information Systemaccessed June 19, 2012 http://www.epa.gov/iris (http://www.epa.gov/iris)
- Volkamer , R. , Molina , L.T. , Molina , M.J. , Shirley , T. and Brune , W.H. 2005 . DOAS measurement of glyoxal as an indicator for fast VOC chemistry in urban air . Geophys. Res. Lett , 32 : L08806 doi: 10.1029/2005GL022616
- Waters Corporation . 2007 . Determination of Formaldehyde in Ambient Air , Milford , MA : Waters Corporation . 720001988EN MC-CP
- Wilkinson , M.C. , Sherwood , R. , Hear,n , J. and Goodall , A.R. 1979 . The influence of residual styrene monomer, benzaldehyde, and microbial activity on the surface properties of polystyrene lattices . Br. Polym. J , 11 ( 1 ) : 1 – 6 . doi: 10.1002/pi.4980110102
- Windholz , M. , Budavari , S. , Blumetti , R.F. and Otterbein , E.S. 1983 . The Merck Index: An Encyclopedia of Chemicals, Drugs, and Biologicals , Rahway , NJ : Merck and Company .
- Wolken , W.A.M. , Tramper , J. and van der Werf , M.J. 2004 . Amino acid-catalysed retroaldol condensation: the production of natural benzaldehyde and other flavour compounds . Flavour Fragrance J , 19 ( 2 ) : 115 – 20 . doi: 10.1002/ffj.1326
- World Health Organization . 1998 . Environmental Health Criteria, No 207—Acetone , Geneva , , Switzerland : WHO .
- World Health Organization . 2000 . Guidelines for Air Quality , Geneva , , Switzerland : WHO .
- World Health Organization . 2010 . WHO Guidelines For Indoor Air Quality—Selected Pollutants , Geneva , , Switzerland : WHO Regional Office for Europe .
- Zhang , J. , Lioy , P.J. and He , Q. 1994 . Characteristics of aldehydes: Concentrations, sources, and exposures for indoor and outdoor residential microenvrionments . Environ. Sci. Technol , 28 ( 1 ) : 146 – 52 . doi: 10.1021/es00050a020