Abstract
Several U.S. state and tribal agencies and other countries have implemented a methodology developed in the arid intermountain western U.S. where short-term (1- to 3-hr) particulate matter (PM) with aerodynamic diameters less than 2.5 µm (PM2.5) concentrations are estimated from an observed visual range (VR) measurement. This PM2.5 concentration estimate is then linked to a public health warning scale to inform the public about potential health impacts from smoke from wildfire. This methodology is often used where monitoring data do not exist (such as many rural areas). This work summarizes the various approaches, highlights the potential for wildfire smoke impact messaging conflicts at state and international borders, and highlights the need to define consistent short-term health impact category breakpoint categories. Is air quality “unhealthy” when 1- to 3-hr PM2.5 is ≥ 139 µg/m3 as specified in the Wildfire Smoke, A Guide for Public Health Officials? Or is air quality unhealthy when 1- to 3-hr PM2.5 is ≥ 88.6 μg/m3 as specified in the Montana categorizations? This work then examines the relationship between visual range and PM2.5 concentrations using data from the Interagency Monitoring of PROtected Visual Environments (IMPROVE) program and the IMPROVE extinction coefficient (βext) equation to simulate an atmosphere dominated by smoke for sites in the arid intermountain western U.S. and great plains. This was accomplished by rearranging the βext equation to solve for organic mass as a function of VR. The results show that PM2.5 and VR are related by PM2.5 = 622 * VR−0.98 with a correlation of 0.99 and that at low VR values (<10 km) a small change in VR results in a large change in PM2.5 concentrations. The results also show that relative humidity and the presence of hygroscopic pollutants from sources other than fire can change the VR/PM2.5 relationships, especially at PM2.5 concentrations less than approximately 90 µg/m3.
This work illustrates the limitations of current approaches linking VR, PM2.5 concentrations, and air quality health impact indices. It is the first published summary of known VR/PM2.5/health impact approaches nationally and internationally and the first application of the IMPROVE data and extinction coefficient equation to investigate these approaches scientifically for smoke-filled atmospheres. These VR/PM2.5/health impact indices are used to estimate PM2.5 concentrations when monitoring data are not available in order to inform the public during periods of wildfire smoke impacts. This work also offers discussion points to inform future work.
Short-Term Exposure Categorizations
Smoke from wildfires can impact air quality locally and on regional scales, causing concerns for public health. Several U.S. state and tribal agencies and other countries have implemented a methodology developed in the arid intermountain western U.S. where short-term (1- to 3-hr) particulate matter (PM) with aerodynamic diameters less than 2.5 μm (PM2.5) concentrations are estimated from an observed visual range (VR) measurement. This PM2.5 concentration estimate is then linked to a public health warning scale to inform the public about potential health impacts from smoke from wildfire. This methodology is often used where monitoring data do not exist (such as many rural areas).
The linkage between VR and short term (1- to 3-hr) PM2.5 concentrations in smoky conditions was originally created from an empirical study performed in Helena, Montana, in the summer of 2000. Then, based on expert local knowledge, a table was created linking VR, 1-hr PM2.5, and a health effect category drawn from the U.S. Environmental Protection Agency's (EPA) air quality index (AQI). The Montana study correlated airport Automated Surface Observing System VR measurements with PM measurements made with a tapered element oscillating microbalance during a period when the Helena, Montana, airport was heavily impacted by smoke from wildfires. Data were taken every 5 min and resulted in a correlation where VR × PM = 450 and VR is in units of miles. Expert knowledge was then applied to establish various short-term health impact breakpoints of PM2.5 concentrations (“unhealthy” etc.). These breakpoints were color-coded by AQI levels and AQI terminology was used, but the breakpoints do not correspond with the AQI. Note that the AQI levels for PM are established by the EPA and are based on 24-hr PM2.5 concentrations. Helena, Montana, is a city located in a rural wide mountain valley where relative humidity (RH) is typically low and background air pollution is also typically low, especially in the summertime when this analysis was conducted. This leads to two important caveats about the application of this study:
1. | It should only be applied if RH < 65%. | ||||
2. | It should only be applied when the majority of air pollution is from wildfire smoke. |
Because a VR can be estimated by the human eye, this VR and PM2.5 relationship was useful for situations where monitoring data measuring PM2.5 concentrations did not exist (such as many rural areas) and there was a need to inform the public about potential health consequences from regional-scale smoke episodes.
In 2001 (with updates in 2008 and 2013), the EPA, California Department of Public Health, California Office of Environmental Health Hazard Assessment, California Air Resources Board, and Missoula County Health Department authored the Wildfire Smoke, A Guide for Public Health Officials (CitationLipsett et al., 2008). The Wildfire Smoke Guide is used nationally and internationally by public health officials to inform the public when wildfire smoke impacts occur. Within the Wildfire Smoke Guide is a table linking 1-, 8-, and 24-hr PM2.5 concentrations with VRs and health impact categories (see ). The visibility column in only corresponds to the 1- to 3-hr PM2.5 concentrations. This table is a variation on the Montana table where additional expert logic was used to set the PM2.5 breakpoint levels for the health impact categories.
The Wildfire Smoke, A Guide for Public Health Officials (CitationLipsett et al., 2008) linkage of air quality index categories with 1-, 8-, and 24-hr PM2.5 concentrations and visibility for arid conditions.
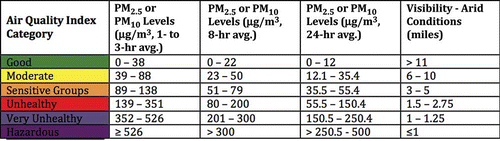
Other state and tribal agencies have since adopted either the Montana table or the Wildfire Smoke Guide table or created a variation of their own. summarizes many of the U.S. state and tribal agencies using a VR, PM2.5, and health index relationship including Fairbanks, Alaska; California; Colorado; Idaho; Montana; the Navajo Nation, New Mexico; and Oregon. Also included in is the Canadian Air Quality Health Index (AQHI) and the Canadian province of Manitoba. The Canadian AQHI is a multipollutant index combining PM2.5, ozone, and nitrogen dioxide (NO2); however, in the values for ozone and NO2 are not included. summarizes the publicly available agency websites and references used in . Note, neither nor is designed to give an exhaustive listing of VR/PM2.5/health index relationships, and other states/tribes/agencies may use other approaches. Many of these VR, PM2.5 concentration, and air quality category tables use different health impact category breakpoints. For example, Montana gives air quality an unhealthy rating if short-term PM2.5 exposure is at a concentration greater than 88.6 μg/m3, whereas the Wildfire Smoke, A Guide for Public Health Officials (used by neighboring Idaho) gives air quality an unhealthy rating if short-term PM2.5 exposure is greater than 139 μg/m3. Thus, there is the potential for different health impact messages to be told to the public for wildfire smoke crossing state lines.
Table of U.S. state and tribal agencies and Canadian agencies using a short-term health impact categorization
Summary of several of the U.S. state and tribal agencies and countries applying a relationship between 1- and 3-hr PM2.5 concentrations and human health impact categories. The Canadian AQHI includes 3-hr average PM2.5, ozone, and NO2; however, for this figure, the ozone and NO2 concentrations are set to zero. The “Wildfire Smoke Guide” is the Wildfire Smoke, A Guide for Public Health Officials (CitationLipsett et al., 2008) publication and it is used by California, Idaho, Oregon, and the Navajo Nation. *Manitoba is using the same scale as Fairbanks, Alaska, and Colorado with the exception that the “Moderate” and “Unhealthy for Sensitive Groups” categories are combined into a single category, which for purposes of this figure has been color-coded to the “Unhealthy for Sensitive Groups” category color.
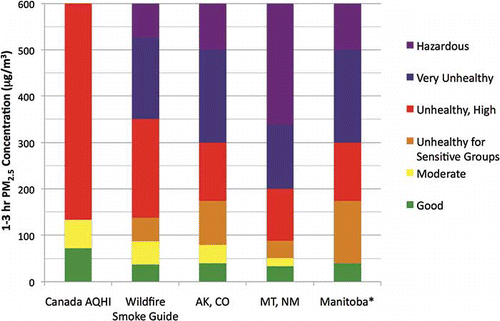
The EPA (2007) acknowledges that there is a need for a public advisory system based on short-term (e.g., 1- to 3-hr) exposures to PM2.5 concentrations during events such as wildfires but that there is not enough information on which to base a national standard. Canada, meanwhile, has also been trying to address short-term exposures and has used epidemiological data to develop a multipollutant Air Quality Health Index (CitationStieb et al., 2008) that is based on 3-hr average concentrations of PM2.5, ozone, and NO2. The Canadian AQHI is an example of an index that addresses the potentially compounding impacts of multiple pollutants.
This paper further investigates the relationship between VR and PM2.5 concentrations using data and equations from the Interagency Monitoring of PROtected Visual Environments (IMPROVE) program. The analysis includes how the VR/PM2.5 relationship may change with increased organic mass in the atmosphere, which can occur in large wildfire smoke impact situations. The analysis also includes how the presence of other pollutants and higher RH conditions can also influence the VR/PM2.5 relationship.
IMPROVE Data Analysis
A PM2.5 concentration can “look” very different in the atmosphere (and thus translate to a variety of VR estimates) depending on the physical and chemical characteristics of the particles, atmospheric conditions, and the relationship of the human eye with sun angle and reflectance off of objects. For example, and show Great Smoky Mountains National Park when the PM2.5 concentration is 21 μg/m3, with (a) RH = 30% and (b) RH = 90%. With an RH of 30%, the VR was 47 km, whereas at an RH of 90% the VR was 19 km. was generated by the WinHaze program (Molenar, n.d.) using 20% worst-day data from the IMPROVE project. Ammonium sulfate concentrations comprise approximately two thirds of the total PM2.5 concentrations. Ammonium sulfate is highly hygroscopic; thus, at a higher humidity (such as 70%) it will absorb water, causing the aerosol particle to grow and change its light scattering properties, creating a hazier view.
Great Smoky Mountains National Park when PM2.5 concentrations are 21 μg/m3 for (a) RH = 30% and (b) RH = 90%. Visual range is 47 km in (a) and 19 km in (b). Figures and calculations provided by the WinHaze program (Molenar, n.d.).
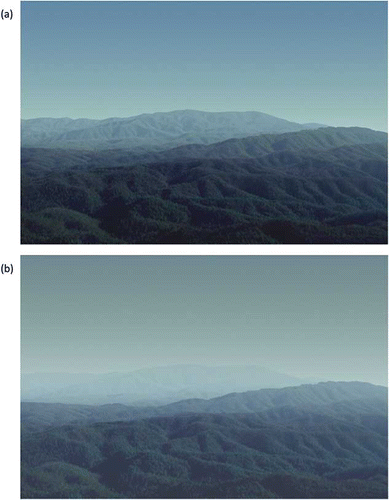
The second version of the IMPROVE extinction coefficient (βext) equation (CitationPitchford et al., 2007; Equationeq 1) and the equation relating βext and VR ( Equationeq 2) developed by Koschmieder (1924) were applied to investigate the relationship between VR and PM2.5 concentration.
EquationEquations 1 and Equation2 were set equal to each other and then rearranged to solve for organic mass as a function of VR ( Equationeq 3). Thus, as VR decreases, organic mass increases. This was done because organic mass is one of the primary aerosols emitted from fires (followed by elemental carbon) and in this way the VR/PM2.5 relationship could be investigated mathematically for a smoky atmosphere. The organic mass components of the equation were further split out to have an organic mass from background/anthropogenic concentrations and organic mass from fire. Though the relationship between VR, βext, and PM2.5 concentrations has long been known and is well studied, this rearrangement of the equations to specifically mathematically simulate a smoky atmosphere is unique and useful to investigate given the various health impact indexes linking VR and PM2.5 that are used to inform the public during regional smoke-filled wildfire episodes.
The IMPROVE βext algorithm used here and as described in CitationPitchford et al. (2007) accounts for the increase in light scattering efficiencies with concentration in the atmosphere for the various PM components. The “large” mode terms represent aged and/or cloud processed particles, whereas the “small” mode terms represent freshly formed particles. The fraction of the fine particle component (sulfate, nitrate, or organic mass) that is in the large mode is estimated by dividing the total concentration of the component by 20 μg/m3. If the total concentration of a component exceeds 20 μg/m3, then all of it is assumed to be in the large mode (CitationPitchford et al., 2007). The Koschmieder equation assumes that the sky brightness of the observer is similar to the sky brightness of the observed object, that there is a homogeneous distribution of pollutants, that the viewing distance is horizontal, that the Earth's curvature can be ignored, that the viewing object is a black object, and that the threshold contrast is 0.02 (CitationEPA, 1979). The threshold contrast of 0.02 indicates that a typical person can perceive a 2% change in visibility and the natural log of 0.02 is 3.9; the value of the Koschmieder coefficient used in this analysis (see CitationEPA [1979, ch. 2] for further details). Once the organic mass from fire was solved for using Equationeq 3, the total PM2.5 concentration was obtained by adding the sulfate, nitrate, organic mass, elemental carbon, and fine soil PM components together.
Calculations were made using Equationeq 3 for IMPROVE sites in the intermountain west and northern Great Plains for months when wildland fire is most likely to occur. IMPROVE sites had been grouped by region in CitationHand et al. (2011) and monthly averages were calculated for each aerosol specie by region and month. Specifically, nine regions from CitationHand et al. (2011) were used: Northern Rockies, Hells Canyon, Northern Great Plains, Great Basin, Central Rockies, Colorado Plateau, Mogollon Plateau, Southern Arizona, and West Texas. IMPROVE monthly average values for each of the regions for 2005–2008 for ammonium sulfate, ammonium nitrate, organic mass, light-absorbing carbon, fine soil, sea salt, and coarse mass were applied from CitationHand et al. (2011). Rayleigh scattering was assumed to be 10/Mm, and NO2 was neglected. shows how PM2.5 varies with VR for the IMPROVE sites in the nine regions. For VR values of 2, 5, 10, 20, and 50 km the rearranged βext equation ( Equationeq 3) was solved for each region and identified fire month. The black diamonds in are the median PM2.5 values calculated for each of the VR distances. A power law curve fit then yields the relationship shown in Equationeq 4 with a regression correlation of 0.99.
PM2.5 as a function of VR for IMPROVE sites in the intermountain west and northern Great Plains for months when wildland fire is most likely to occur. IMPROVE sites had been grouped by region in CitationHand et al. (2011) and monthly average values for each of the regions for 2005–2008 for ammonium sulfate, ammonium nitrate, organic mass, light-absorbing carbon, fine soil, sea salt, and coarse mass were applied from CitationHand et al. (2011). For VR values of 2, 5, 10, 20, and 50 km the rearranged βext equation ( Equationeq 3) was solved for each region and identified fire month. The black diamonds are the median PM2.5 values calculated for each of the VR distances. A power law curve fit yields the relationship PM2.5 = 622 * VR−0.98 with a regression correlation of 0.99.
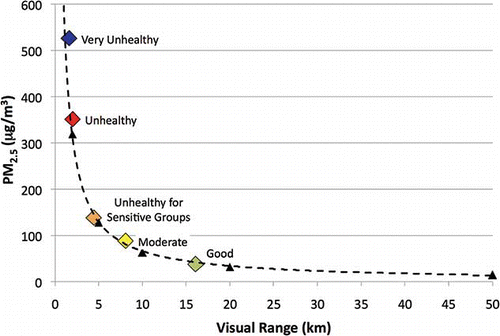
Figure 4 and Equationeq 4 illustrate the nonlinear relationship between VR and PM2.5 concentration. For VR = 1–10 km, on average a 1-km change in VR results in a 62 μg/m3 change in PM2.5 per kilometer. Then, for VR = 10–100 km, on average a 1-km change in VR results in a 1 μg/m3 change in PM2.5 per kilometer. This illustrates how a small (e.g., 1 km) error in a VR estimate (such as what can happen with the human eye) can have a large impact on estimated PM2.5 concentrations. also includes the VR/PM2.5 breakpoints from the Wildfire Smoke Guide () for the 1-hr PM2.5 concentrations.
Further analysis using Equationeq 3 was conducted for a humid environment that experiences greater background anthropogenic PM2.5 concentrations than the arid intermountain western U.S. Shenandoah National Park, located in the Appalachian Mountains of Virginia, was utilized for this and 1988–1998 IMPROVE 20% worst days average background concentrations for ammonium sulfate (14.21 μg/m3), ammonium nitrate (0.45 μg/m3), background organic mass carbon (4.17 μg/m3), light-absorbing carbon (0.53 μg/m3), fine soil (0.74 μg/m3), and course mass (6.47 μg/m3) were applied (CitationMolenar, 2001). Rayleigh scattering was assumed to be 10/Mm, and sea salt and NO2 were not accounted for. is a table of PM2.5 concentrations and it illustrates how PM2.5 concentrations can change as a function of RH and VR using Equationeq 3. Color-coded cells contain PM2.5 concentrations; the two left columns are VR in miles and kilometers, respectively, and RH is across the top of the table. PM2.5 concentration cells are color-coded according to the Wildfire Smoke, A Guide for Public Health Officials health effect categories in and the 1-hr PM2.5 breakpoints.
Calculated PM2.5 concentrations for Shenandoah National Park as a function of VR and RH based on rearranging the second form of the IMPROVE extinction coefficient equation to solve for organic mass as a function of VR. As VR is decreased, organic mass will increase, simulating a smoke-filled atmosphere. IMPROVE 20% worst-day base data for ammonium sulfate, ammonium nitrate, light-absorbing carbon, fine soil, and coarse mass were included. Color-coding is according to the 1-hr PM2.5 breakpoints from the Wildfire Smoke, A Guide for Public Health Officials (CitationLipsett et al., 2008; see Figure 1).
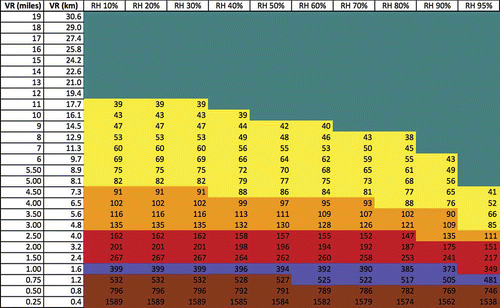
Figure 5 shows how the relationship between VR and PM2.5 is most sensitive to RH in the lower ranges of PM2.5 values (and longer/higher VR values). For example, at 30% RH, air quality is “good” (e.g., green) when the VR is 12 miles or greater, but at 90% RH, air quality is good when the VR is 7 miles or greater. Good air quality, as listed in the Wildfire Smoke, A Guide for Public Health Officials, is when 1- to 3-hr PM2.5 concentration is between 0 and 38 μg/m3. In the “moderate” air quality categorization, the influence of RH becomes less pronounced, where air quality is moderate at 30% RH when the VR is 5–11 miles, but at 90% RH, air quality is moderate when the VR is 4–6 miles. Moderate air quality, as listed in the Wildfire Smoke, A Guide for Public Health Officials, is when 1- to 3-hr PM2.5 concentration is between 39 and 88 μg/m3. At the higher impact categories (e.g., unhealthy, etc.), the difference in VR between RH = 30% and RH = 90% is half a mile or less, and below 2.5 miles VR, the categorizations are not greatly influenced by RH. This is because the organic mass is dominating the atmospheric aerosol loading and PM2.5 concentration becomes independent of RH. Potential organic aerosol hygroscopicity was not addressed in this work.
Discussion
Several U.S. state and tribal agencies and other countries have implemented a methodology developed in the arid intermountain western U.S. where short-term (1- to 3-hr) PM2.5 concentrations are estimated from an observed VR measurement. This PM2.5 concentration estimate is then linked to a public health warning scale to inform the public about potential health impacts from smoke from wildfire. summarizes many of these approaches. This methodology is used in situations where monitoring data measuring PM2.5 concentrations do not exist (such as many rural areas) and there is a need to inform the public about potential health consequences from regional-scale smoke episodes. This section discusses issues highlighted by the analysis in the previous two sections.
Consequence of a human-sighted visual range
The human eye operates much like a camera, detecting differences in brightness; thus, the geometry between an observer and the sun and the angle with which the sun illuminates a vista play a role in how a vista is perceived (CitationMalm, 1999). CitationMalm (1999) also discussed how sun angle can play a role, where with a higher sun angle there is less scattering of light and therefore a greater perceived scenic distance for the same particulate matter concentrations in the atmosphere. The Wildfire Smoke, A Guide for Public Health Officials includes instructions about how to estimate a VR with the human eye; however, the method cannot adjust for atmospheric conditions such as RH.
A human-sighted VR is an instantaneous value, which is then extrapolated to a time-averaged PM2.5 concentration. The inherent assumption is that the conditions/concentrations “seen” at the moment are constant for the next 1–3 hr. CitationSchichtel and Husar (1999) found that human-sighted VR did not correlate well with PM2.5 concentrations. They analyzed data for the eastern U.S. for the time period 1948–1992 from stations operated by the National Weather Service, Navy, Air Force, and Federal Aviation Administration. Finally, this work shows that at the lower VR values (1–10 km) a small (1 km) error in a VR estimate can result in a large error in PM2.5 concentration estimation (from 10 to over 100 μg/m3). There is concern that a human-sighted VR can contain greater uncertainty than the PM2.5 ranges in , especially at the higher health impact levels.
Multiple approaches currently in use
Figure 1 and Table 1 summarize many of the approaches used to inform the public about potential short-term health impacts from wildfire smoke. Many of these approaches change when crossing state lines. For example, Montana gives air quality an unhealthy rating if short-term PM2.5 exposure is at a concentration greater than 88.6 μg/m3, whereas the Wildfire Smoke, A Guide for Public Health Officials (used by neighboring Idaho) gives air quality an unhealthy rating if short-term PM2.5 exposure is greater than 139 μg/m3. If you have a wildfire at the Idaho/Montana border, what do you tell the public? Does the public in Idaho get a different message than the public in Montana? And which health category breakpoint is “correct”?
Need for a short-term health impact index
There is a need for a science-based short-term (1- to 3-hr) health impact index public advisory system, something the U.S. EPA recognizes (EPA, 2007). Canada also recognized a similar need and through a multistakeholder process developed an index for acute exposures in order to inform the public (CitationStieb et al., 2008). The Canadian AQHI is a multipollutant (O3, NO2, and PM2.5) short-term (3-hr) exposure metric derived from urban-based epidemiological data.
PM2.5 is the pollutant most commonly associated with wildfire smoke, and the current U.S. national ambient air quality standard for PM2.5 is a 24-hr average of 35 μg/m3 and an annual average of 12 μg/m3. The U.S. AQI system is based on the 24-hr PM2.5 value, and the various approaches to a 1- to 3-hr health index public advisory systems draw upon AQI terminology but should not be confused with being AQI values. With wildland fire there is also the potential for multipollutant impacts due to the mixing of the smoke plume with surrounding regional pollution and due to emissions from the fire itself, which can contain NO2, volatile organic compounds, carbon monoxide, and other trace gas species. Throughout this discussion the assumption has been made that it is biomass only that is burning; however, in cases where structures are burning (such as in the wildland urban interface), the chemical composition of the fire emissions can be very different, further complicating a potential index and the messaging that should go to the public.
Influence of relative humidity and other pollutant sources on the VR/PM2.5 relationship
Finally, though there is a need for consistency across state lines, there is also a need to recognize that different regional atmospheric conditions (such as RH) can influence the VR/PM2.5 relationship. The analysis described in this paper using Shenandoah National Park highlights how RH can play a large role in the PM2.5/VR relationship, especially when other hygroscopic pollutants are present. This influence of RH and other pollutant sources is most evident at the good and moderate short-term PM2.5 categories as defined by the Wildfire Smoke, A Guide for Public Health Officials (e.g., when 1- to 3-hr PM2.5 concentration is less than 88 μg/m3). Once VR drops below approximately 2.5 miles, the influence of RH and other pollutant sources becomes negligible because the atmospheric aerosol loading is dominated by organic mass.
Summary and Recommendations
This work summarizes the VR, short-term (1- to 3-hr) PM2.5 concentrations and health impact categorizations used by many U.S. state and tribal agencies as well as Canada to inform the public during regional-scale wildfire smoke impacts. In addition, it examines the relationship between VR and PM2.5 concentrations when regional-scale wildfire smoke impacts occur, using the IMPROVE data and βext equation, and provides a discussion of concerns.
The summarization of the U.S. state and tribal agency and Canadian PM2.5/VR/health impact categorizations shows how many different approaches exist and highlights the potential for wildfire smoke impact messaging confusion at state and international borders. It also highlights the need to know what the short-term health impact category breakpoints should be set at.
The application of the IMPROVE data and βext equation to simulate a smoky atmosphere is unique and was accomplished by rearranging the IMPROVE βext equation ( Equationeq 1) and the Koschmieder VR/βext relationship ( Equationeq 2) to solve for organic mass as a function of VR ( Equationeq 3). Thus, as VR decreases, organic mass increases, mathematically simulating a smoke-filled atmosphere. Calculations were made using Equationeq 3 for IMPROVE sites in the intermountain west and northern Great Plains for months when wildland fire is most likely to occur. IMPROVE monthly average values for each of the regions for 2005–2008 for ammonium sulfate, ammonium nitrate, organic mass, light-absorbing carbon, fine soil, sea salt, and coarse mass were applied from CitationHand et al. (2011). Analysis yielded a VR/PM2.5 relationship of PM2.5 = 622 * VR−0.98 with a correlation of 0.99. These results also illustrate how at low VR values (<10 km) a small change in VR results in a large change in PM2.5 concentration.
Finally, there is a need for a short-term health impact categorization system applicable outside the U.S. arid intermountain west. This was investigated for Shenandoah National Park, an environment more humid than the arid intermountain west and an area impacted by other hygroscopic pollutant sources. The results show that the relationship between VR and PM2.5 is most sensitive to RH in the lower ranges of PM2.5 values (and longer/higher VR values). For example, at 30% RH, air quality was good when the VR was 12 miles or greater, but at 90% RH, air quality was good when the VR was 7 miles or greater. Good air quality, as listed in the Wildfire Smoke, A Guide for Public Health Officials, is when 1- to 3-hr PM2.5 concentrations are between 0 and 38 μg/m3. Below 2.5 miles VR, the air quality categorizations are not greatly influenced by RH because the organic mass is dominating the atmospheric aerosol loading and, using the methods in this analysis, PM2.5 concentration becomes independent of RH.
Acknowledgment
The National Wildfire Coordinating Group (NWCG) Smoke Committee (SmoC) provided the impetus for this work and the authors thank the SmoC for their insightful and spirited discussions on this topic. The authors also thank John Coefield and the Montana Department of Environmental Quality for discussing the original development of this methodology. The authors also want to thank visibility experts Bill Malm, Scott Copeland, and Bret Schichtel for providing their insights and expertise. Finally, the authors thank the Joint Fire Science Program (JFSP) project “Sub-canopy Transport and Dispersion of Smoke: A Unique Observation Dataset and Model Evaluation” (project #09-1-04-2) for supporting the publication of this work.
References
- Canada, 2013. (accessed June 14, 2013) http://www.gov.mb.ca/health/publichealth/wildlandfiresmokeexposure.pdf (http://www.gov.mb.ca/health/publichealth/wildlandfiresmokeexposure.pdf)
- Fairbanks, Alaska, 2013. (accessed June 14, 2013) http://co.fairbanks.ak.us/AirQuality/Docs/Particulate Levels.pdf (http://co.fairbanks.ak.us/AirQuality/Docs/Particulate Levels.pdf)
- Hand, J.L., S.A. Copeland, D.E. Day, A.M. Dillner, H. Indresand, W.C. Malm, C.E. McDade, C.T. Moore, B.A. Schichtel, and J.G. Watson. Spatial and Seasonal Patterns and Temporal Variability of Haze and Its Constituents in the United States: Report V [Online], 2011. (accessed April 7, 2013) http://vista.cira.colostate.edu/improve/Publications/Reports/2011/2011.htm (http://vista.cira.colostate.edu/improve/Publications/Reports/2011/2011.htm)
- Interagency Monitoring of PROtected Visual Environments. WinHaze [Online]. (accessed April 7, 2013) http://vista.cira.colostate.edu/improve/tools/win_haze.htm (http://vista.cira.colostate.edu/improve/tools/win_haze.htm)
- Koschmeider, H. 1924. Theories der Horizontalen Schtweite. Beilr. Phys. Frei. Atmos. 12:33–53
- Lipsett, M., B. Materna, S.L. Stone, S. Therriault, R. Blaisdell, J. Cook, J. Waldman, L. Wohl-Sanchez, L. Kent, P. Jenkins, D. Westerdahl, T. Phillips, L. Smith, J. Behrman, S. DuTeaux, R. Lam, D. Gold, B. Nakamura, A. Smith, and M. Brauer. Wildfire Smoke, A Guide for Public Health Officials [Online], 2008. (accessed April 7, 2013) http://oehha.ca.gov/air/risk_assess/wildfirev8.pdf (http://oehha.ca.gov/air/risk_assess/wildfirev8.pdf)
- Malm, W.C. Introduction to Visibility. Cooperative Institute for Research in the Atmosphere (CIRA) NPS Visibility Program [Online], 1999. (accessed April 7, 2013) http://www.epa.gov/visibility/pdfs/introvis.pdf (http://www.epa.gov/visibility/pdfs/introvis.pdf)
- Molenar, J. WinHaze - A computer-imaging software program. Air Resources Specialists Inc. [Online], n.d. (accessed April 7, 2013) http://vista.cira.colostate.edu/improve/tools/win_haze.htm (http://vista.cira.colostate.edu/improve/tools/win_haze.htm)
- Navajo Nation, 2013. (accessed June 14, 2013) http://www.navajonationepa.org/airq/wildfire11.html (http://www.navajonationepa.org/airq/wildfire11.html)
- Revised Algorithm for Estimating Light Extinction from IMPROVE Particle Speciation Data . Journal of the Air & Waste Management Association , 57 1326 – 1336 . Pitchford, M., W. Malm, B. Schichtel, N. Kumar, D. Lowenthal, and J. Hand doi: 10.3155/1047–3289.57.11.1326
- Schichtel , B.A. and R.B. , Husar . , Grant No. 827981. Research Triangle Park, NC: U.S. Environmental Protection Agency . Evaluation of the Models-3C/CMAQ System—Relationship between Airport Visibility and PM2.5 Concentrations in the Eastern U.S doi: 10.3155/1047–3289.57.11.1326
- State of Colorado, 2013. (accessed June 14, 2013) http://www.colorado.gov/airquality/wildfire.aspx (http://www.colorado.gov/airquality/wildfire.aspx)
- State of Idaho, 2013. (accessed June 14, 2013) http://www.deq.idaho.gov/media/883596---visibility---guide.pdf (http://www.deq.idaho.gov/media/883596---visibility---guide.pdf)
- State of Montana, 2013. Revised.mcpx (accessed June 14, 2013) http://www.deq.mt.gov/FireUpdates/Breakpoints (http://www.deq.mt.gov/FireUpdates/Breakpoints)
- State of New Mexico, 2013. (accessed June 14, 2013) http://www.nmenv.state.nm.us/aqb/Wildfire---PM.html (http://www.nmenv.state.nm.us/aqb/Wildfire---PM.html)
- State of Oregon, 2013. (accessed June 14, 2013) http://www.deq.state.or.us/aq/aqi/docs/ForestFire CatBreak.pdf (http://www.deq.state.or.us/aq/aqi/docs/ForestFire CatBreak.pdf)
- New Multipollutant, No-Threshold Air Quality Health Index Based on Short-Term Associations Observed in Daily Time-Series Analyses . Journal of the Air & Waste Management Association , 58 ( 3 ) 435 – 450 . Stieb, D.M., R.T. Burnett, M. Smith-Doiron, O. Brion, H.H. Shin, and V.A. Economou. doi: 10.3155/1047–3289.57.11.1326
- U.S. Environmental Protection Agency. 1979. Protecting Visibility: An EPA Report to Congress Research Triangle Park, NC: U.S. Environmental Protection Agency Office of Air, Noise, and Radiation Office of Air Quality, Planning, and Standards.
- U.S. Environmental Protection Agency. OAQPS Issue Paper for Discussion at National Air Quality Conference, February 2007 [Online], 2007. (accessed April 7, 2012) http://www.epa.gov/airnow/aqi_issue_paper_020707.pdf (http://www.epa.gov/airnow/aqi_issue_paper_020707.pdf)