Abstract
In this study, gaseous benzene, toluene, ethylbenzene, and o-xylene (BTEX) were extracted by passive needle trap samplers (NTS) using divinylbenzene (DVB) particles (mesh sizes 60–80, 80–100, and 100–120, respectively) as packed sorbents. An aspirating pump measured sampling flow rates of NTS, and the relations between BTEX mass and sampling flow rates were sufficient to maintain the extraction performance of these self-designed DVB-NTS. Furthermore, this investigation compared the extraction efficiency of NTS with that of the 100-µm polydimethylsiloxane solid-phase microextration (PDMS SPME) fiber when applied to sample heating products from electric-vaporization anti-mosquito mats, and the experimental results indicated that NTS effectiveness increased with decreasing adsorbent particle diameter. Substantially less mass of gaseous BTEX was extracted using 100-µm PDMS SPME fiber than with NTS of 100–120 mesh DVB for 60-min TWA sampling of anti-mosquito mats. The 100–120 mesh DVB-NTS primarily adsorbed 4.2 ng acetone, 13.3 ng dichloromethane, and 4.5–25.3 ng C10–C12 alkanes.
Implications:
The needle trap sampler (NTS) has been evaluated to be a device for sampling heating products from electric-vaporization anti-mosquito mats. Based on the experimental results, this investigation assessed NTS as suitable for occupational and environmental health applications.
Introduction
The U.S. Environmental Protection Agency (EPA) is considering recommending longer-term sampling to achieve more accurate time-weighted-average (TWA) detections for indoor air monitoring of volatile organic compounds (VOCs) (Odencrantz et al., Citation2009). Due to the health effects caused by indoor VOCs, the demand for fast, solventless sampling and analysis methods to monitor VOCs has increased (Warren and Pawliszyn, Citation2011). The passive diffusion sampler for quantifying indoor VOCs is common practice throughout Europe (Odencrantz et al., Citation2009), and an environmentally friendly approach, solid-phase microextraction (SPME), has been widely used to sample gaseous organic compounds (Lord and Pawliszyn, Citation2000). Based on the distance from the needle opening, the commercial SPME () can sample on-site VOCs via a passive TWA sampling mode (Cheng, Citation2008). The needle trap sampler (NTS; ) is an extraction trap containing a sorbent within a small needle via which VOCs can be passively introduced to the extraction phase by diffusion (Lord et al., Citation2010). However, although TWA sampling of VOCs theoretically resembles sampling by SPME, users can select sorbent types packed in a NTS and their packing structures based on the adsorption characteristics of analytes (Warren and Pawliszyn, Citation2011; Zhan and Pawliszyn, Citation2012). Gong et al. (Citation2008) packed an NTS with sorbent Carboxen 1000 as a TWA diffusive sampler to collect benzene, toluene, ethylene, and o-xylene (BTEX) under different sampling conditions, namely, airflow speeds of 1.44–32.48 cm/min and relative humidity (RH) of 17–53%. Relative standard deviation (RSD) values for BTEX mass extracted under different sampling conditions were below 5%, which indicated that airflow and RH were independent of NTS extraction performance. Cheng et al. (Citation2011) also extracted gaseous toluene, ethylbenzene, and o-xylene via 60–80 mesh divinylbenzene (DVB) particle as a sorbent packed in NTS. The feasibility of using these self-designed DVB-NTS as diffusive TWA samplers for occupational hygiene applications was assessed for 4–12-hr sampling periods under 180–210 ppm VOCs. In practical applications of occupational and environmental health, Cheng et al. (Citation2012) used NTS as the breakthrough indicator for the activated carbon adsorbent; and Cheng et al. (Citation2013) further confirmed the feasibility of sampling gaseous and particle-bound VOCs from mosquito coils combustion.
This investigation used DVB particles with mesh sizes of 60–80, 80–100, and 100–120 as sorbents packed in passive NTS. In all NTS, the sampling flow rates were measured through the packing phase. The relations between extracted organic compound mass and its sampling flow rate were also assessed. These qualified self-prepared NTS were used to sample products from heating electric-vaporization anti-mosquito mats. The 100-μm polydimethylsiloxane (PDMS) SPME fibers were simultaneously sampled to compare the extraction efficiency of the NTS.
Methods
Theory of gaseous compound extraction via a needle trap sampler
The only mechanism for gaseous compounds extraction through the needle is diffusion. During this process, the linear gaseous concentration profile (c(Z), as shown in ) established along the diffusion path distance (Z) between the small needle opening and the extraction phase position is characterized by the opening area (A) and the diffusion path length. The amount of analyte extracted (n) during time interval (t) can be estimated using Fick’s first law of diffusion (Lord et al., Citation2010):
As expected, the quality of extracted analyte is proportional to the integral amount of the sample concentration over time (C(t)) under constant Dm for given VOCs under the uniform needle opening area and diffusion path distance. Notably, eqs 1 and 2 are valid only when the analyte extracted onto the sorbent is a small fraction of the equilibrium amount for the lowest concentration in the sample, which can be assessed based on the RSD of double or triple duplicates of measurements, which is typically below 5% in this study.
Chemicals, materials, and equipment
The 3.5-inch, 22-G stainless steel needles (inner diameter [ID] 0.41 mm and outer diameter [OD] 0.71 mm) were bought from Herling Co. Ltd. (Pingtung, Taiwan). The DVB adsorbent particles, which were packed as sorbents in the NTS, as well as the 100-μm PDMS SPME fiber, were obtained from Supelco (Bellefonte, PA, USA). The aspirating pump for testing the sampling flow rates of NTS was from Kitagawa (AP-20; Kawasaki, Japan), and the 5-min syringe epoxy glue was from Nao-Pao Applied Material Co. Ltd. (Taoyuan, Taiwan). All gases for chromatographic analysis were supplied by Jing-De Gases Co., Ltd. (Kaohsiung, Taiwan) and were of ultrahigh purity. Additionally, the tested VOCs (benzene, toluene, ethylbenzene, and o-xylene [BTEX]) were obtained from Merck, Taiwan Branch (Taipei, Taiwan). The electric-vaporization anti-mosquito mats and associated hot plate used in the tests were Mieh-Fei brand, and manufactured by Chu-Chen Industrial Co., Ltd. (Taipei, Taiwan).
The BTEX were analyzed using a gas chromatograph (GC) (6890N; Agilent Technologies, Inc., Wilmington, DE, USA) equipped with a flame ionization detector (FID). The VOC separations were performed using a HP19091Z-413 HP-1 methyl siloxane (30 m × 320 μm × 0.25 μm) capillary column (Agilent Technologies). The heating products of electric-vaporization mosquito coils were also identified via the mass spectrometry equipment (5973N; Agilent Technologies). The packed mass of DVB particles were measured using a precision scale (ME type; Sartorius, Göttingen, Germany) with a minimum reading of 0.0001 mg and reproducibility <0.0001 mg.
Preparation of needle trap sampler
The NTS mainly comprised a stainless steel needle packed with DVB particles with mesh sizes of 60–80, 80–100, and 100–120 (). Sorbent particles in the positioned needle were immobilized without bleeding. A stainless steel wire spring plug was initially fixed in position (Z = 10 mm). The DVB particles were then individually packed by aspiration until the desired length of 7 mm was reached; namely, the diffusion path was 3 mm long. Packing was then performed by applying a very small amount of epoxy glue to the exposed portion of the sorbent layer to firmly hold the sorbent particles. Precision measurements demonstrated that the packed DVB mass of the tested NTS average of 0.347 mg (standard deviation of 0.0380 mg for total 14 NTS packing adsorbents in this study). For convenience, another long, straight stainless steel wire was used as a pole to moisten the epoxy glue. To avoid the epoxy-resin plug completely blocking the NTS, air was continuously drawn through the NTS packing during epoxy curing. Finally, the DVB packed in the NTS was conditioned by heating at the injection port of the gas chromatography–flame ionization detection (GC-FID) spectrometer under 260 °C for 30 min. The prepared NTS was stored at room temperature until use. Fourteen pieces of NTS were prepared (4–6 pieces per DVB adsorbent diameter range).
All NTS were examined in terms of the desired sampling flow rates (mL/min) drawn by an aspirating pump through the packing phase. The RSD for triple duplication tests of sampling flow rates should be less than 5%. The filled materials in the NTS thus should be considered uniformly immobilized. Sampler reproducibility was also assessed using the RSD of adsorbed VOC mass between different NTS (Cheng et al., Citation2011, Citation2013; Warren, Citation2011; Zhan, Citation2011).
Sampling and analysis
Static VOC samples were prepared by injecting BTEX into a 1000-mL glass bulb. All NTS were used to take static BTEX samples within the bulb in an oven heated to 27 °C. In each experiment, VOC calibrations were performed with a gas-tight syringe sampler. The VOC analysis of both calibrations and TWA samples were also performed using the same GC-FID. After VOC sampling, NTS and SPME were injected into the injection ports of GC for VOC desorption and subsequent analysis. The desorption time at the injection port of GC was 30 sec. The temperature of the GC increased from 50 °C at increments of 15 °C/min. The final temperature was 180 °C, with a holding time of 2 min. The FID detector was heated to 300 °C. The carrying gas flow rate was 1.2 mL/min for nitrogen, and the split off operation mode was selected. Notably, following the operation and subsequent analysis, no carryovers were available for samples extracted using NTS and SPME.
Sampling and analysis products from electric-vaporization anti-mosquito mats
Electric-vaporization anti-mosquito mats were heated in a circular glass chamber (inner dimensions: 30 cm [diameter] and 22 cm [height], with total volume of approximately 17.5 L). Air was then pumped into the chamber at a flow rate of 0.5 L/min as the anti-mosquito mat was heated. Initially, triple duplicate samplings were taken using 1-L Teflon bags (SKC, Eighty Four, PA, USA) and then analyzed with gas chromatography–mass spectrometry (GC-MS) to quantify the gaseous products of anti-mosquito mats. lists the concentration distributions of gaseous products. For sampling using test samplers, the SPME and NTS were inserted into the chamber through the septa, which sealed the sampling ports installed on the side of the chamber. The PDMS SPME fibers and NTS were exposed in the chamber for TWA sampling and then analyzed via the GC-FID.
Table 1. Main air compounds of heating electric-vaporization anti-mosquito mats
Results and Discussion
Products of heating electric-vaporization anti-mosquito mats
lists the 29 most significant chemicals (concentrations >1.0 ppbv) to heat electric-vaporization anti-mosquito mats. Notably, the heated products included VOCs of dichloromethane (211 ppbv) and acetone (80 ppbv), and C10–C12 alkanes (135–754 ppbv). Dichloromethane is the Group B2 cancer classification (probable human carcinogen) (EPA, 2000), and the National Toxicology Program (Citation2005) also identified dichloromethane as a likely human carcinogen; its threshold limit value (TLV) is 50 ppmv (American Conference of Industrial Hygienists [ACGIH], Citation1996), which significant exceeds the 221 ppbv actually measured in this study. Acetone and C10–C12 alkanes primarily cause irritation of the human respiratory tract, headache, sleepiness, and dermatitis.
Tests of the sampling flow rates of needle trap samplers
Fourteen pieces of NTS, packed with DVB adsorbents with three different diameter ranges (60–80, 80–100, 100–120 meshes) were used to sample gaseous BTEX of 100 ppm in glass bulbs. shows that, for all NTS, the RSD values for sampling flow were below 5%, which indicated uniformity of the packed DVB phases. However, the mean sampling flow rates of NTS gradually decreased with the declining of diameters of the packed DVB; namely, the NTS packed with 60–80 mesh DVB had the highest mean flow rate (81.6 mL/min), whereas that packed with 100–120 mesh had the lowest (49.8 mL/min). Compared with the largest-diameter adsorbents, the smaller adsorbents had lower porosity in the packing zone.
Table 2. Sampling flow rates for needle trap samplers in this study
VOC extraction rates of needle trap samplers
shows that BTEX adsorption by NTS of 60–80, 80–100, and 100–120 meshes increased from 1 to 2 hr. Additionally, shows that BTEX extraction rates (BTEX extraction mass/sampling time) are inversely proportional to adsorbent diameters. The NTS packed with 100–120 meshes DVB actually had substantially higher BTEX extraction rates than that packed with 60–80 meshes DVB. Equation 2 shows that VOC (n) extraction by NTS correlates with sampling time (t) under the constant VOC concentrations (C). However, further comparison of VOC extraction rates for different extraction times revealed that they remained almost constant for extraction times of 1–2 hr for NTS of individual DVB adsorbents. However, studies found that when the extraction times were increased to 4 hr, the extraction rates decreased for NTS under over equilibrium adsorption (Cheng et al., Citation2013). This phenomenon shows that the extracting mass of BTEX seldom reaches equilibrium for 2-hr adsorption under BTEX concentrations of 100 ppm.
Table 3. BTEX extracting mass and rates for needle trap samplers
Sampling by SPME fiber and NTS for electric-vaporization anti-mosquito mats
and display the analyzed integral area by GC-FID of pollutants from electric-vaporization anti-mosquito mats using SPME fiber and NTS for TWA sampling time of 60 min. The main five compounds for tested electric-vaporization mosquito coils are acetone, dichloromethane, n-decane, n-undecane, and dodecane, which also were exactly identified by GC-MS (see ). The integral area of individual compounds extracted using 100-μm PDMS SPME fiber nearly equaled those of 60–80 and 80–100 mesh DVB-NTS. Comparison of all mesh DVB-NTS and SPME fiber revealed that the NTS packed with 100–120 mesh DVB adsorbed most VOCs, that is, 4.2 ng acetone, 13.3 ng dichloromethane, and 4.5–25.3 ng 10–12-C alkanes. Notably, the chromatograph diagrams of 100-μm PDMS SPME fiber in showed the compounds extracted from heating products were multiplicate (numerous peaks); however, the peak area for extraction with SPME fiber was obviously less than for 100–120 mesh DVB-NTS ( and ). The experimental results demonstrated that SPME fibers can generally extract wide distributions of organic compounds, whereas man-made NTS, packed with adsorbents of proper diameter and affinity to adsorbates, extract a larger mass of specific chemicals.
Table 4. Gas chromatographic analysis for main products from electric-vaporization anti-mosquito mats via needle trap and SPME samplers
Figure 3. Chromatograph diagrams of NTS and SPME for extracting products from electric-vaporization anti-mosquito mats.
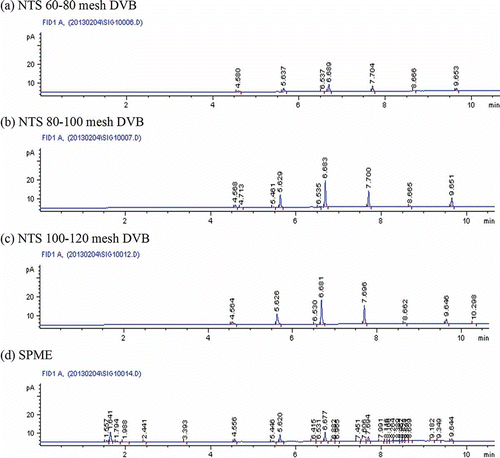
In an earlier study (Cheng et al., Citation2013), following three to five times samplings using DVB-NTS for the products of mosquito coils combustion, the overall performances for collecting gaseous VOCs failed because of clogging of the diffusion path between adsorbents packed in the samplers, and no further integral peak counts of VOCs could be analyzed via the GC-FID. This phenomenon of clogging by combustion aerosols in the packed phase after several samplings was also reported by tests on cigarette burning (Warren, Citation2011) and man-made aerosols (medium diameters of around 100 nm) (Zhan, Citation2011). These experimental tests demonstrate that for aerosol extraction by NTS, clogging during the packed phase is the main limitation when sampling products from heating sources, particularly large-scale-diameter aerosols, for example, mosquito coil aerosol and cigarette smoke. To specify whether electric-vaporization anti-mosquito mats will clog NTS, this study repeated ten 60-min samplings using a single 100–120 mesh DVB-NTS. No decrease in VOC extraction performance was observed during the long-time sampling of electric-vaporization mosquito coils. This test clearly showed that heating of electric-vaporization anti-mosquito mats does not result in suspension of critical air aerosols as occurs for combustion of mosquito coils.
Conclusion
The packed DVB-NTS is a recommended passive sampler because it extracts a larger BTEX mass than does 100-μm PDMS SPME fiber when applied to sample heating elements in electric-vaporization anti-mosquito mats. NTS can be promoted as a novel personal sampler for air compounds in work places. Method 1501 of the National Institute of Occupational Safety and Health (NIOSH, Citation2003) requires workers to wear a charcoal sorbent tube connected to an active personal sampling pump when assessing exposure to organic compounds. Workers often complain about the inconvenience of carrying the equipment to comply with this procedure. This study evaluated the feasibility of applying a small sampler, such as a badge-like or pen-like NTS, for occupational hygiene applications. Typically, NTS, packed with smaller diameter adsorbent (100–120 mesh DVB particles) achieves higher indoor air compounds extraction efficiency than NTS packed with larger particle size adosrbents. This investigation assessed NTS as suitable for occupational and environmental health applications.
Funding
The authors would like to thank the National Science Council, Taiwan, Republic of China, for financially supporting this research under contract no. NSC 99-2622-E-242-001-CC3. Mr. Ted Knoy is appreciated for his editorial assistance.
Acknowledgment
Mr. Ted Knoy is appreciated for his editorial assistance.
Additional information
Notes on contributors
Wen-Hsi Cheng
Wen-Hsi Cheng is a professor and Jia-Rong Jiang is an experimental assistant at the Department of Occupational Safety and Hygiene, Fooyin University, Kaohsiung City, Taiwan, Republic of China.
Chitsan Lin
Chitsan Lin is a professor at the Department of Marine Environmental Engineering, National Kaohsiung Marine University, Kaohsiung City, Taiwan, Republic of China.
Jyun-Jie Liou
Jyun-Jie Liou, Zih-Hao Wu, Ya-Han Hsu, and Zih-Yi Yang are undergraduates at the Department of Occupational Safety and Hygiene, Fooyin University.
References
- American Conference of Industrial Hygienists. 1996. TLV and BEIs Threshold Limit Value for Chemical Substances and Physical Agents, Biological Exposure Indices. Cincinnati, OH: American Conference of Industrial Hygienists.
- Cheng, W.H. 2008. Adsorption characteristics of granular activated carbon and SPME indication of VOCs breakthrough. Aerosol Air Qual. Res. 8:178–187.
- Cheng, W.H., J.R. Jiang, Y.N. Huang, S.C. Huang, and Y.P. Yu. 2012. Breakthrough indicator for aromatic VOCs using needle trap samplers for activated carbon adsorbent, J. Air Waste Manage. Assoc. 62:948–954. doi:10.1080/10962247.2012.693056
- Cheng, W.H., W. Zhan, and J. Pawliszyn. 2011. Extraction of gaseous VOCs using passive needle trap samplers. Aerosol Air Qual. Res. 11:387–392.
- Cheng, W.H., W. Zhan, and J. Pawliszyn. 2013. Gaseous and particle-bound VOC products of combustion extracted by needle trap samplers. J. Chin. Chem. Soc. 60:1027–1032. doi:10.1002/jccs.201200654
- Gong, Y., I. Y. Eom, D. W. Lou, D. Hein, and J. Pawliszyn. 2008. Development and application of a needle trap device for time-weighted average diffusive sampling. Anal. Chem. 80:7275–7282. doi:10.1021/ac800884f
- Lord, H., and J. Pawliszyn. 2000. Evolution of solid phase microextraction technology. J. Chromatogr. A 885:153–193. doi:10.1016/S0021-9673(00)00535-5
- Lord, H., W. Zhan, and J. Pawliszyn. 2010. Fundamentals and applications of needle trap devices. Anal. Chim. Acta 677:3–18. doi:10.1016/j.aca.2010.06.020
- National Institute of Occupational Safety and Health. 2003. Manual of Analytical Methods, 4th ed. Atlanta, GA: National Institute of Occupational Safety and Health.
- National Toxicology Program. 2005. Eleventh Report on Carcinogens: Dichloromethane, 2005. http://ntp.niehs.nih.gov/ntp/roc/eleventh/profiles/s066dich.pdf (available as of July 31, 2009; accessed June 18, 2013).
- Odencrantz, J.E., S.C. Thornley, and H. O’Neill. 2009. An evaluation of the performance of multiple passive diffusion devices for indoor air sampling of VOCs. Remediation 19:63–72. doi:10.1002/rem.20217
- U.S. Environmental Protection Agency. 2000. Integrated Risk Information System (IRIS). Summary on Dichloromethane. http://www.epa.gov/iris/ (available as of March 15, 2000; accessed June 18, 2013).
- Warren, J. 2011. Needle trap device and solid phase microextraction combined with portable GC-MS for on-site applications. Master thesis, University of Waterloo, Waterloo, Ontario, Canada.
- Warren, J., and J. Pawliszyn. 2011. Development and evaluation of needle trap device geometry and packing methods for automated and manual analysis. J. Chromatogr. A 1218:8982– 8988. doi:10.1016/j.chroma.2011.10.017
- Zhan, W. 2011. Optimization of needle trap device. Master thesis, University of Waterloo, Waterloo, Ontario, Canada.
- Zhan, W., and J. Pawliszyn. 2012. Investigation and optimization of particle dimensions for needle trap device as an exhaustive active sampler. J. Chromatogr. A 1260:54– 60. doi:10.1016/j.chroma.2012.08.089