Abstract
A self-designed device was applied to treat a simulated exhaust gas loaded with toluene by aqueous solutions of five kinds of fluorocarbon surfactant (FS-3100, FS-22, FSN-100, FSO-100 and FSG) under the controlled laboratory conditions. The simulated exhaust gas is generated by a mixture of clean air and toluene vapor, and its toluene concentration can be controlled by changing the volume ratio of the inlet air to the vapor. Two mass transfer methods: liquid-liquid transfer and gas-liquid transfer, were compared for their toluene saturation capacities of these absorbent solutions, and it was found that more toluene was dissolved by the liquid-liquid transfer than by the gas-liquid transfer. According to the saturation capacities of these absorbent solutions and their Henry’s Constants, FSO-100 is the best absorbent to treat the simulated exhaust gas and was selected for further experiments. The FSO-100 absorbent solution with a concentration of 0.1 % shows an efficient absorption to the simulated exhaust gas, with a toluene saturation capacity of 4.2 mg/g. Heating distillation (90- 95 oC) is highly efficient to recover toluene from the FSO-100 absorbent solution as well as regenerate it. A toluene recovery of about 85 % was achieved. The regenerated absorption solution can keep its initial toluene absorption capacity during the reuse.
Implications: There is a lack of information on fluorocarbon surfactants to be used for removing volatile organic compounds (VOCs) of industrial exhaust gases. This research investigated five kinds of fluorocarbon surfactant with different chemical structures as absorbents to treat a simulated exhaust gas loaded with toluene, and made a comparison between their toluene saturation capacities. The research revealed that FSO-100 (an ethoxylated nonionic fluorosurfactant) presents the best performance in trapping toluene, and heating distillation is feasible to recover toluene and regenerate the absorbent solution. The knowledge gained in this research provides a theoretical basis for selecting an appropriate fluorocarbon surfactant and preparing its aqueous solution to treat industrial exhaust gases loaded with toluene.
Introduction
The total amount of volatile organic compounds (VOCs) from waste gases generated by industrial productions in China is estimated to be more than 12 million tons per year (CEGPC, 2010). Thermal oxidation, catalytic oxidation, biodegradation, and photolysis and liquid absorption are techniques recommended to remove VOCs from the waste gases (Khan and Ghoshal, Citation2000; Zehraou et al., Citation2012; Kim et al., Citation2014). Among these, liquid absorption has been addressed for a long period of time, since it is possible to recover organic solvents from absorption liquids that absorb VOCs (Geisthardt et al., Citation1989; Stockley, Citation1994; Heymes et al., Citation2006; Ma and Wang, Citation2009; Roizard et al., Citation2009; Xiang et al., Citation2010; Berenjian et al., Citation2012; Khan et al., Citation2013; Jia et al., Citation2014; You, Citation2014). The essential principle of this method is that VOCs in exhaust gases are first transferred into an absorption liquid through physical absorption, and the absorbed VOCs are then recovered by distillation, air stripping, pervaporation, and other techniques (Volodin et al., Citation1997a; Majumdar et al., Citation2001; Wang et al., Citation2001; Heymes et al., Citation2007). The key of this method is the selection of a suitable absorption liquid for a specific exhaust gas (Heymes et al., Citation2006; Zhang et al., Citation2014). An absorption liquid that is attractive for industrial processes should have the following properties: (a) a high capacity to absorb VOCs, (b) a recyclable property through a simple regeneration, and (c) no toxicity nor fire or explosion risks (Tao, Citation1999; Khan and Ghoshal, Citation2000; Heymes et al., Citation2006; Zhu and Liu, Citation2008).
The absorption liquids can be largely classified into two types: organic solvents, and aqueous solutions of surfactants. The solvents reported from literature reports include mainly different oils (e.g., mineral oil, vegetable oil, silicone oil, and diesel oil: Armand et al., Citation1990; Poddar and Sirkar, Citation1996; Assuncao and Vasconcelos, Citation1997; Volodin et al., Citation1997a; Xia et al., Citation1999), some high-class alcohols (e.g., polyethylene glycol: Porter et al., Citation1991; Cotte et al., Citation1995; Gearhart, Citation1998; Heymes et al., Citation2006), di(2-ethylhexyl) adipate (Heymes et al., Citation2006), and diisobutyl phthalate (Heymes et al., Citation2006). However, they are usually flammable, explosive, and expensive, and easily cause secondary pollution, which restrains their practical uses.
In recent years, a development of the liquid absorption method has been to apply some surfactants to improve the solubility of VOCs in aqueous solutions. According to literature reports, aqueous solutions of Tween 81 and pentaethylene glycol monododecyl ether (Park et al., Citation2008), Span 20 (Park et al., Citation2008; Tian et al., Citation2010), β-cyclodextrin (β-CD; Blach et al., Citation2008), sodium citrate (Cen et al., Citation2007), sodium acetate (Chen et al., Citation2009), and some cationic surfactants (Jiang et al., Citation2010) present an obvious absorption to a hydrophobic VOC (e.g., toluene). Here are some data based on a dynamic absorption experiment. The toluene absorption capacity is about 0.51 mg/g for the aqueous solution of the β-CD at an inlet concentration of 500 mg/m3 and a gas flow rate of 60 L/hr (Blach et al., Citation2008), about 0.093 mg/mL and 0.142 mg/mL for the aqueous solution of the cetyl trimethyl ammonium bromide and for its microemulsion formed with a cosurfactant, respectively, at an inlet concentration of 2000 mg/m3 and a gas flow rate of 18 L/hr (Jiang et al., Citation2010), and 0.11 mg/mL for the aqueous solution of Tween 20 at the same conditions as the latter case (Tian et al., Citation2010). However, the absorption capacities of these surfactants are obviously lower as compared with solvent absorbents, although the experimental conditions are not totally similar. For instance, Heymes et al. (Citation2006) reported the toluene absorption capacities of seven solvent absorbents ranging from 0.39 to 1.09 g/L and from 4.22 to 13.2 g/L at an inlet concentration of 380 mg/m3 and 3700 mg/m3, respectively (a gas flow rate of 100 L/hr in both cases). Additionally, heating distillation is usually unsuitable to regenerate their absorption solution for reuse owing to their lower thermal stability, and there is lack of research on other regeneration methods (Cen et al., Citation2007; Park et al., Citation2008; Chen et al., Citation2009). For these reasons, these surfactants have not been applied to treat exhaust gases loaded with VOCs on an industrial scale.
In order to improve the performance of hydrocarbon surfactants, new surfactants with special chemical structures have been developed, and fluorocarbon surfactant or fluorosurfactant is a typical representative. This surfactant has a strong surface activity and a high thermal stability, and is now widely applied in high-performance extinguishers, high-rank cleaners, and high-temperature emulsifiers (Hoffmann and Wurtz, Citation1997; Estelle et al., Citation2005). However, it is rare to report its use to treat industrial exhaust gases loaded with VOCs. On the basis of the characteristics of fluorocarbon surfactant, it will be more suitable than hydrocarbon surfactants for this purpose.
Nonionic surfactants are generally applied to make oil-in-water emulsions (Myers, Citation1992), present a stronger solubilization to a hydrophobic VOC, as compared with anionic or cationic surfactants with a similar length hydrophobic chain (Kile and Chiou, Citation1989), and some of them are proposed to treat industrial exhaust gases loaded with VOCs (e.g., Park et al., Citation2008). Therefore, in this paper, some nonionic fluorocarbon surfactants were used as absorbents to form their aqueous solutions to treat a simulated exhaust gas loaded with toluene under controlled laboratory conditions. Their absorption capacities were compared. One of them with the best performance was further selected to investigate the influence of its concentration on the absorption capacity, and the feasibility of recovering toluene from its aqueous solution and regenerating the absorbent for reuses by heating distillation.
Materials
Five kinds of nonionic fluorocarbon surfactant with a serial number of FSG, FS-22, FS-3100, FSN-10,0 and FSO-100, respectively, used in the present study were purchased from Du Pont Company (USA). FSG and FS-22 belong to the fluorinated acrylic copolymers, and FS-3100, FSN-100, and FSO-100 belong to the ethoxylated fluorosurfactants. Although they have different chemical structures, they are soluble in water and it is easy to prepare their aqueous solutions. Some available properties of the fluorosurfactants are presented in .
Table 1. Some available physical and chemical properties of the selected fluorocarbon surfactants
Experimental device
The principle of the experimental device is shown in . A tank of compressed dry air (the ratio of nitrogen to oxygen is the same with air) (1) provides an air flow with a pressure. The air flux is controlled by two rotary flowmeters (2) and split into two paths. One path of air passes through a vessel (4: vessel 1) with some amounts of liquid toluene, and the passed air will contain some amount of toluene vapor. The gas stream mixes with another path of clean air in another vessel (6: vessel 2), where the toluene-bearing gas is diluted to form a simulated exhaust gas with a desirable toluene concentration. The simulated exhaust gas then enters into a gas-dispersing device located at the bottom of a long vessel (8: vessel 3) filled with an absorption solution. As the result, the incoming gas is effectively dispersed to form small bubbles to pass through the absorption solution. The tail gas is finally emitted from a small hole opened at the top of the absorption vessel. During the experiment, the toluene concentration in the simulated exhaust gas and the inlet air flux can be controlled by adjusting the two rotary flowmeters (2).
Figure 1. A sketch map showing the formation and absorption of the simulated exhaust gas loaded with toluene: 1, air bottle; 2, rotary flowmeter; 3, valve; 4, vessel 1 (organic exhaust gas producer); 5, thermostatic bath; 6, vessel 2 (organic exhaust gas dilution vessel); 7, gas dispersing device; 8, vessel 3 (glass tube).
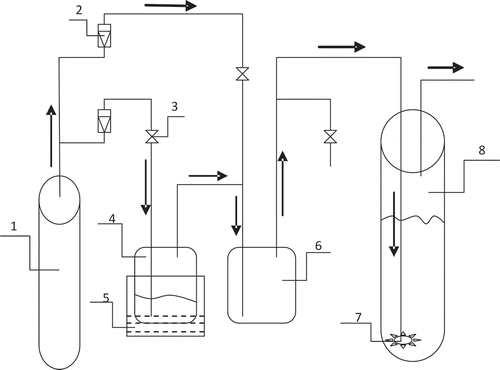
Methods
The absorption solutions used in the present study were made up according to the optimum weight ratio of the fluorocarbon surfactants to water (0.1:99.9) suggested in the product description, that is, a concentration of 0.1%. These absorption solutions were used to compare their absorption capacities to toluene through liquid–liquid mass transfer and gas–liquid mass transfer.
The liquid–liquid mass transfer experiment was conducted by fully mixing 25 g of an absorption solution with 15 g toluene (an excess amount) in a closed glass tube. The mixture was then centrifuged for 30 min to separate the upper undissolved toluene from the absorption solution. The toluene concentration absorbed in the absorption solution was determined by an ultraviolet absorption method. This toluene concentration is regarded as the saturation capacity of the absorption solution by the liquid–liquid mass transfer.
A gas–liquid mass transfer experiment was conducted using the self-designed absorption device under the conditions of 25 g of an absorption solution, 25 L/hh of total inlet gas flow with a toluene concentration of 3000 mg/m3, and a room temperature of 25ºC. After the absorption solution reaches its saturation toluene absorption, its toluene concentration was determined by an ultraviolet absorption method.
The toluene concentration of the inlet gas and outlet tail gas was measured by a Mini RAE 3000 VOC detector (made in the United States). This instrument is equipped with a third-generation photoionization detector (PID), and isobutylene is taken as the calibration material, with an error of ≤3% within its detection range of VOCs. A quantitative detection for VOCs concentration in a gas can be carried out by the detector in a very short time (the shortest responding time is 2 sec). Thus, it is possible to conduct an on-line VOCs measurement. The instrument can only report a total concentration of VOCs since there is no separation column as in a gas chromatography instrument, which can separate a mixture into single compounds. The simulated exhaust gas used in the experiment contains only toluene; thus, the total VOCs concentration measured by the VOC detector is regarded as the toluene concentration.
A whole absorption curve of an absorption solution to treat the simulated exhaust gas was built according to the data obtained by the VOC detector, which was set to record the toluene concentration of an outlet tail gas once per minute until its toluene concentration was equal to that of the inlet gas.
The toluene concentration in an absorption solution was determined by a Lambda 850 ultraviolet and visible spectrophotometer. For each measurement, the maximum absorption wavelength for toluene was fixed to 261 nm and a quartz colorimetric tube with 1 cm optical length was used. The main procedures are: (1) A series of ethanol-toluene solutions with toluene concentrations ranging from 0 mg/g to 10 mg/g was prepared and their absorbances were measured to establish a standard relation curve between their toluene concentrations and absorbances; (2) an absorption solution with its saturation toluene concentration was diluted to control the toluene concentration within the range of the standard curve; (3) the absorbance of the diluted solution was measured and its toluene concentration was determined according to the standard curve; and (4) the toluene concentration of the absorption solution was calculated by multiplying the times of the dilution.
Three main absorption parameters of an absorption solution to treat the simulated exhaust gas can be obtained according to the absorption experiment data:
Henry’s constant (H):
(1)
Saturation toluene concentration (cL, mg/g). This was directly determined by the ultraviolet absorption method already described.
Toluene absorptivity at a certain absorption time (At, %):
According to the saturation toluene concentration and Henry’s constant of the aqueous solutions of the five kinds of fluorocarbon surfactant for treating the simulated exhaust gas, one of them with the greatest absorption capacity can be further selected for two experiments: influence of absorbent concentration on the absorption capacity, and regeneration of absorbent solution for reuse.
A series of absorption solutions with a concentration (the selected fluorocarbon surfactant) of 0.01%, 0.05%, 0.1%, 0.5%, 1%, 1.5%, and 2%, respectively, was prepared to investigate the influence of absorbent concentration on the absorption capacity. The absorption experiments were conducted under the same conditions as the gas–liquid mass transfer, that is, 25 g of absorption solution, 25 L/hr of total inlet gas flow with 3000 mg/m3 of toluene concentration, and a room temperature of 25ºC. The toluene concentration in the outlet tail gas and the absorption solution was determined by the method already described.
Theoretically, such techniques as pervaporation (Heymes et al., Citation2007), air stripping (Blach et al., Citation2008), solvent extraction (Volodin et al., Citation1997b), and heating distillation (Cen et al., Citation2007; Chen et al., Citation2009) can more or less recover toluene from the absorption solution used in the present study. Another aim in recovering toluene is to regenerate the absorption solution for reuse. With consideration of the excellent thermal stability of the used fluorocarbon surfactant, as well as the much more simple equipment required for heating distillation than for the other techniques, heating distillation was selected to recover toluene and regenerate the absorption solution in the present study. The detailed process is as follows. A saturated toluene absorption solution obtained under the conditions as mentioned earlier (25 g of absorption solution, 25 L/hr of total inlet gas flow with 3000 mg/m3 of toluene concentration, and a room temperature of 25ºC) was distilled at 90–95ºC to obtain a distillate that includes water and toluene. After the toluene was separated from the water, the recovered toluene was weighed to calculate the initial toluene recovery of the absorption solution. The separated water was then returned to the distilled residual to keep the original constitutes of the absorption solution. The same experiment process (including absorption, distillation and regeneration) already described was repeated five times for reusing the absorption solution. For each cycle, the saturation toluene concentration was measured and the toluene recovery was calculated. By using these data, variations of the absorption capacity of the absorption solution can be evaluated during the reuse. For the absorption solution, its toluene recovery (Pn) is calculated by following formula:
Results and Discussion
Comparison of absorption capacities of different absorbents
The liquid–liquid mass transfer experiment carried out in the present study in fact gives a theoretical saturation toluene concentration achieved by an absorption solution. It mainly depends on the physical and chemical properties and the concentration of the surfactant used in the absorption solution. However, the gas–liquid mass transfer experiment gives an actual saturation toluene concentration, and it is also influenced by some other factors, such as gas–liquid ratio, inlet gas toluene concentration, and so on. For a specific absorption solution, the saturation toluene concentration based on the liquid–liquid mass transfer will be much greater than that on the gas–liquid mass transfer (Park et al., Citation2008). shows the saturation toluene absorption concentrations of the absorption solutions of the five kinds of fluorocarbon surfactant with concentrations of 0.05% and 0.1%. It can be seen that these absorbent solutions with different concentration have different saturation toluene concentrations for the two mass transfer manners. The FSO-100 absorbent solution shows the greatest saturation concentration, the FSN-100 is the second, FS-3100 is the third, and the saturation concentration of the other two absorbent solutions (FS-22 and FSG) is obviously lower. It seems that the absorbent concentrations do not alter this sequence. A positive correlation can be observed between the two kinds of saturation toluene concentrations obtained by the liquid–liquid mass transfer and the gas–liquid mass transfer, respectively (). For an absorption solution, a higher saturation toluene concentration obtained by the liquid–liquid mass transfer indicates a higher absorption capacity for gaseous toluene. This relationship will be useful to rapidly find a suitable fluorocarbon surfactant from a few candidates as an absorbent to treat a specific exhaust gas loaded with toluene according to the saturation concentration of the liquid–liquid mass transfer.
Figure 2. A comparison of saturation toluene concentrations of the absorption solutions of the studied fluorocarbon surfactants with absorbent concentrations of 0.05% and 0.1% obtained by liquid–liquid mass transfer (a) and gas–liquid mass transfer (b).
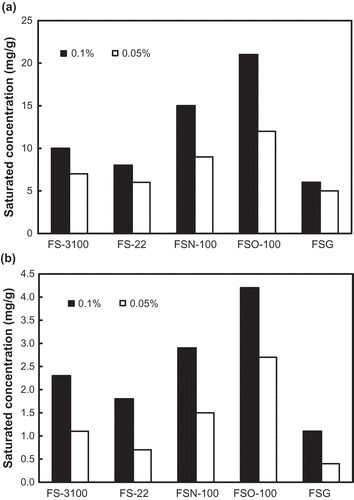
Figure 3. Plot showing the relationship between the two kinds of saturation toluene concentrations obtained respectively by the liquid–liquid mass transfer and gas–liquid mass transfer for absorption solutions of the studied fluorocarbon surfactants.
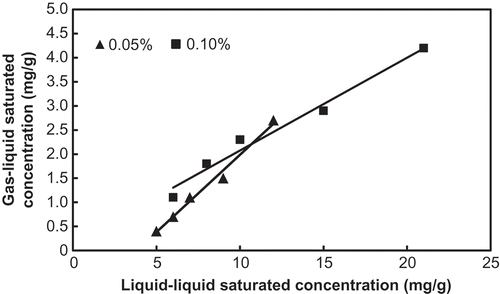
presents the absorption curves of the absorption solutions for the simulated exhaust gas with absorbent concentrations of 0.05% (a) and 0.1% (b). Their absorption curves are similar in shapes, but different in the absorptivities and absorption durations. The FSO-100 absorbent solution shows a very high toluene absorptivity (about 92%) at the beginning of the absorption, and the high absorptivity (>85%) can hold for a longer absorption duration, and then the absorptivity goes down rapidly, to reach zero. The curve inflection point of the FSO-100 absorbent solution occurs at about 40 min (0.05%) and 60 min (0.1%), and the time to arrive to its saturation absorption is about 123 min (0.05%) and 178 min (0.1%). The FSN-100 absorbent solution can keep a toluene absorptivity of 90–85% for about 20 min (0.05%) and 50 min (0.1%), and the saturation absorption point occurs at 75 min (0.05%) and 120 min (0.1%). For the other three absorbent solutions, maximum absorptivities range from 85% to 80%; the higher absorptivity holds only for a short period with the inflection point at 5–15 min (0.05%) and 1–20 min (0.1%), and their saturation absorption point occurs at about 30–60 min (0.05%) and 50–100 min (0.1%).
Figure 4. Absorption curves of the absorption solutions of the studied fluorocarbon surfactants to the simulated exhaust gas with an inlet toluene concentration of 3000 mg/m3. The absorbent concentrations in the absorption solutions are 0.05% (a) and 0.1% (b).
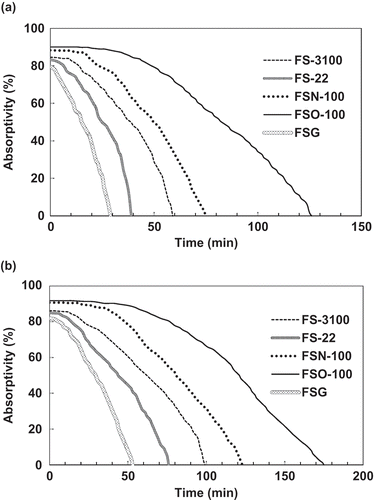
The differences in the toluene absorption capacities of the absorption solutions of the five kinds of fluorocarbon surfactant should be mainly related to their chemical structures and surfactant nature, although there is yet lack of relative knowledge on solubilization concerned with fluorocarbon surfactant, as indicated by Matsuoka et al. (Citation2014). It is widely accepted that solubilization is caused by micellization in a surfactant aqueous solution when the surfactant concentration is over its critical micelle concentration (CMC) value (Edward et al., Citation1991; Myers, Citation1992), and the solubilization capacity for a specific hydrophobic VOC (e.g., toluene) is positively correlated with the concentration of micelles, which mainly depends on the nature and concentration of the surfactant (Liu et al., Citation2010). As compared with the two high-polymer fluorosurfactants (FSG and FS-22), the three ethoxylated fluorosurfactants (FS-3100, FSN-100, and FSO-100) are inferred to have a much lower molecular mass, and would form a greater micelle concentration at the same weight concentration (e.g., 0.1%) if their CMC values (molar concentration) are at a similar level, which may be a possible reason for the greater toluene absorption capacities of these ethoxylated fluorosurfactants. For different ethoxylated nonionic hydrocarbon surfactants, a longer ethoxylated chain will inhibit micellization to reduce micelle concentration (Yoem et al., Citation1996), which may be extrapolated to explain the different absorption capacities of the studied three ethoxylated nonionic fluorosurfactant surfactants. For example, FSN-100 and FSO-100 are similar in their CF2 groups, but have on average 14 and 10 polyoxyethylene units, respectively (Szymczyk et al., Citation2014), and a greater toluene absorption capacity of FSO-100 was achieved from the present study.
According to the present study result, it is believed that the ethoxylated nonionic fluorocarbon surfactant (FSO-100) shows a stronger toluene absorption capability than the other fluorocarbon surfactants.
Henry’s constant and selection of absorbents
For the present study, the Henry’s constant of an absorption solution reflects the ratio of the toluene concentration in gas phase to that in liquid phase at the absorption equilibrium state (i.e., the saturation toluene absorption state). Therefore, a lower Henry’s constant (H) or a higher reciprocal value of the Henry’s constant (1/H) of an absorption solution suggests a higher saturation toluene concentration in the absorption solution, which indicates a better absorption capability. The comparison of the 1/H values of these absorption solutions with an absorbent concentration of 0.1% shows that the FSO-100 absorbent solution has the highest 1/H value of 5.29 (gas–liquid mass transfer), respectively ().
Table 2. Henry’s constant reciprocal values of the absorption solutions with an absorbent concentration of 0.1%
It is also noted that for the absorption solution, its saturated toluene absorption concentrations obtained by the liquid–liquid mass transfer is much greater than that by gas–liquid mass transfer. This is mainly because the toluene concentration gradient between the two liquid phases is much greater than that between gas phase and liquid phase, enhancing the diffusing trend of toluene into the absorption solution.
According to the data on the saturation toluene absorption concentration, absorption curve, and Henry’s constants of these absorption solutions, FSO-100 fluorocarbon surfactant presents the best toluene absorption performance for the simulated exhaust gas.
Effect of absorbent concentration
The absorbent concentration of an absorption solution is an important factor to influence its absorption capability to VOCs (Blach et al., Citation2008). Further experiments were taken to investigate the absorption capability of the aqueous solutions of FSO-100 with different concentrations to the simulated exhaust gas. From and , the saturation toluene concentration of these absorption solution increases with increasing the absorbent concentration, and the increasing extent is obviously different in different absorbent concentration ranges. The saturation toluene concentration increases remarkably from 0.9 mg/g to 4.2 mg/g with increasing the absorbent concentration from 0.01% to 0.1%, but increases very slowly after the absorbent concentration is more than 0.1%. The saturation toluene concentration is only 4.8 mg/g when the absorbent concentration increases to 2%. Thus, with consideration of economy, an absorbent concentration of 0.1% is suggested for the FSO-100 absorbent solution in practical use.
Figure 5. Absorption curves of FSO-100 absorbent solutions with concentrations from 0.01% to 2%. The inlet gas toluene concentration is 3000 mg/m3.
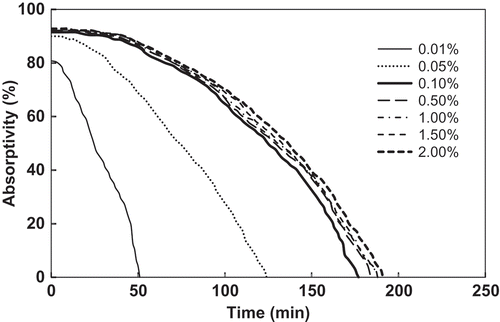
Table 3. Saturation toluene concentrations of the FSO-100 absorbent solutions with different concentrations
Toluene recovery and absorption solution reuse
The distillation experiment on an absorption solution with FSO-100 concentration of 0.1% and its saturation toluene absorption concentration of 4.2 mg/g indicates that an azeotropy occurred when the heating temperature was in the range of 90–95ºC, most of the toluene was easily distilled out from the absorption solution in a short period time (3–5 min), and there was no a significant increase of the distilled toluene amount with further increasing distillation time or rising the distillation temperature. Thus, the temperature to recover toluene from the absorbent solution was set to 90–95ºC with a distillation duration of 5 min.
From the result of the five times of distillation and reuse of the FSO-100 absorbent solution (with concentration of 0.1%), it can be seen that the shapes of their absorption curves are totally identical (), their saturation toluene concentrations do not decrease with increasing the reused times (), and their toluene recovery is very similar, in the range of 80–90% (). Thus, heating distillation (≤95ºC) does not damage the chemical structures of the fluorocarbon surfactant, and is the feasible method to recover toluene and regenerate the absorption solution.
Figure 6. Absorption curves of the FSO-100 absorbent solution to the simulated exhaust gas during reusing.
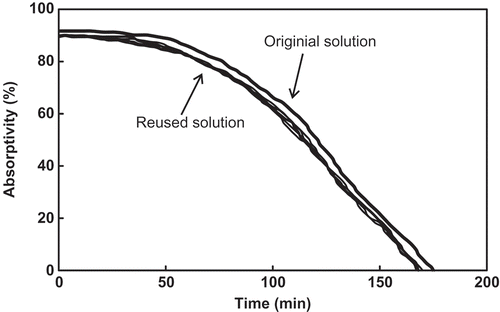
Figure 7. Variations of saturation toluene concentration of the FSO-100 absorbent solution during distillation and reusing.
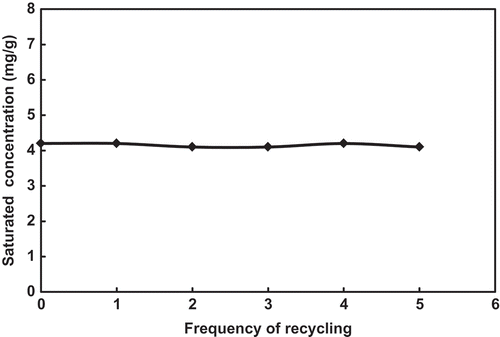
Figure 8. Variations of toluene recovery from the FSO-100 absorbent solution during distillation and reusing.
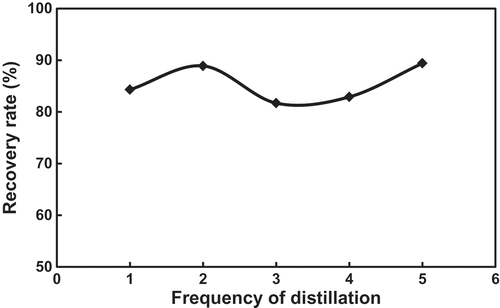
For industrial applications of an absorption solution, a reasonable design of regeneration cycle is necessary, and its saturation capacity to a specific waste gas is critically important for this purpose. For the proposed FSO-100 absorbent solution (e.g., a concentration of 0.1%), the experiment data presented in are equivalent to a scale of 1000 kg (about 1 m3) of the absorption solution to be used to treat a gas flow of 1000 m3/hr with a toluene concentration of 3000 mg/m3, having an absorption duration of about 160 min to reach the saturation capacity. Toluene concentrations in waste gases from most industries range between 500 and 1500 mg/m3, and thus the absorption duration will be much longer in practical use as compared with the experiment. This is a simple theoretical enlargement. It is necessary to conduct an industrial pilot-scale test to determine these relative absorption parameters of the FSO-100 absorbent solution in further work.
Conclusion
The aqueous solutions of the five kinds of fluorocarbon surfactants were used to treat the simulated exhaust gas loaded with toluene under controlled laboratory conditions. The following conclusions have been drawn.
The aqueous solutions of these fluorocarbon surfactants have quite different toluene absorption capacities. Fluorocarbon surfactant FSO-100 is shown to be highly efficient to trap toluene.
The absorption capacity of the aqueous solution of FSO-100 will increase with increasing its concentration from 0.01% to 2%, but a concentration of 0.1% is effective and economic.
Heating distillation (90–95ºC) is highly efficient to recover toluene from the FSO-100 absorbent solution. The regenerated absorption solution can keep its initial toluene absorption capacities during reuse.
Funding
This research is financially supported by the Science and Technology Bureau of Guangdong Province (2010B090400550) and Development of Reformation Committee of Guangdong Province (2011A709). This is contribution NO.IS-1973 from GIGGAS.
Additional information
Notes on contributors
Xiao Xiao
Xiao Xiao is a Ph.D. candidate and Bo Yan and Jiamo Fu are professors at the State Key Laboratory of Organic Geochemistry, Guangzhou Institute of Geochemistry, Chinese Academy of Sciences, Guangzhou, Guangdong, China.
Bo Yan
Xiao Xiao is a Ph.D. candidate and Bo Yan and Jiamo Fu are professors at the State Key Laboratory of Organic Geochemistry, Guangzhou Institute of Geochemistry, Chinese Academy of Sciences, Guangzhou, Guangdong, China.
Jiamo Fu
Xiao Xiao is a Ph.D. candidate and Bo Yan and Jiamo Fu are professors at the State Key Laboratory of Organic Geochemistry, Guangzhou Institute of Geochemistry, Chinese Academy of Sciences, Guangzhou, Guangdong, China.
Xianming Xiao
Xianming Xiao is a professor at Guangzhou Institute of Geochemistry, Guangzhou, China.
References
- Armand, B.L., H.B. Uddholm, and P.T. Vikstroem. 1990. Absorption method to clean solvent-contaminated process air. Ind. Eng. Chem. Res. 29 (3): 436–39. doi:10.1021/ie00099a020
- Assuncao, J.V.D., and S.M.F. Vasconcelos. 1997. Control of toluene and xylene by absorption in mineral oil. Proceedings of Air and Waste Management Association’s 90th Annual Meeting and Exhibition, Toronto, Canada.
- Berenjian, A., N. Chan, and H. J. Malmiri. 2012. Volatile organic compounds removal methods: a review. Am. J. Biochem. Biotechnol. 8(4): 220–29. doi:10.3844/ajbbsp.2012.220.229
- Blach, P., S. Fourmentin, D. Landy, F. Cazier, and G. Surpateanu. 2008. Cyclodextrins: A new efficient absorbent to treat waste gas streams. Chemosphere 70:374–80. doi:10.1016/j.chemosphere.2007.07.018
- CEGPC (Committee of Exhaust Gas Purification of China). 2011. China development report on prevention and control industry of organic waste gas in 2010. China Environ. Protect. Ind. 8: 8–15 (in Chinese with English abstract). doi:10.3969/j.issn.1006-5377.2011.08.002
- Cen, C.P., D.S. Chen, R.H. Lan, and Z.X. Tang. 2007. Experimental study on treatment of waste gas containing toluene by absorption. Environ. Eng. 25 (6):40–42 (in Chinese with English abstract). doi:10.3969/j.issn.1000-8942.2007.06.012
- Chen, D.S., C.P. Cen, H.M. Zeng, Z.X. Tang, and P. Fang. 2009. Experiment study on absorption purification of waste gas containing toluene by sodium acetate and additives. Environ. Sanit. Eng. 17(2):4–6 (in Chinese with English abstract). doi:10.3969/j.issn.1005-8206.2009.02.002
- Cotte, F., J.L. Fanlo, P. L. Cloirec, and P. Escobar. 1995. Absorption of odorous molecules in aqueous solutions of polyethylene glycols. Environ. Technol. 16(2): 127–36. doi:10.1080/09593331608616253
- Edwards, D.A., R.G. Luthy, and Z.B. Liu. 1991. Solubilization of polycyclic aromatic hydrocarbons in micellar nonionic surfactant solutions. Environ. Sci. Technol. 25:127–33. doi:10.1021/es00013a014
- Estelle, M., G.C. Christine, and S. Claude. 2005. Highly fluoroalkylated amphiphilic triazoles: Regioselective synthesis and evaluation of physicochemical properties. J. Fluorine Chem. 126:715–20. doi:10.1016/j.jfluchem.2005.02.006
- Gearhart, L.E. 1998. New glycol-unit design achieves VOC, BTEX reductions. Oil Gas J. 96(28): 61–64.
- Geisthardt, K., J. Holtze, R. Ludwig, and T. Pilhofer. 1989. Absorption process for the removal of organic solvents from exhaust air. Chem. Eng. Technol. 12(1): 63–70. doi:10.1002/ceat.270120111
- Heymes, F., P.M. Demoustier, F. Charbit, J.L. Fanlo, and P. Moulin. 2006. A new efficient absorption liquid to treat exhaust air loaded with toluene. Chem. Eng. J. 115: 225–31. doi:10.1016/j.cej.2005.10.011
- Heymes, F., P.M. Demoustier, F. Charbit, J.L. Fanlo, and P. Moulin. 2007. Treatment of gas containing hydrophobicVOCs by a hybrid absorption–pervaporation process: The case of toluene. Chem. Eng. Sci. 62:2576–89. doi:10.1016/j.ces.2007.02.001
- Hoffmann, H., and J. Wurtz. 1997. Unusual phenomena in perfluorosurfactants. J. Mol. Liq. 72:191–230. doi:10.1016/S0167-7322(97)00039-1
- Jia. L.J., W.H. Yu, C. Long, and A.M. Li. 2014. Adsorption equilibrium and dynamics of gasoline vapors onto polymeric adsorbents. Environ. Sci. Pollut. Res. 21:3756–63. doi:10.1007/s11356-013-2328-z
- Jiang, L., S.L. Tian, P. Ning, and H. Mo. 2010. Solubilization absorption of toluene vapor by formation of oil-in water microemulsions with cation surfactants. Sep. Sci. Technol. 45:508–14. doi:10.1080/01496390903526378
- Khan, F.I., A.K. Ghoshal. 2000. Removal of volatile organic compounds from polluted air. J. Loss Prevent. Proc. Ind. 13: 527–45. doi:10.1016/S0950-4230(00)00007-3
- Khan, N.A., Z. Hasan, and S.H. Jhung. 2013. Adsorptive removal of hazardous materials using metal-organic frameworks (MOFs): A review. J. Hazard. Mater. 244–45:444–56. doi:10.1016/j.jhazmat.2012.11.011
- Kile, D.E., and C.T. Chiou. 1989. Water solubility enhancements of DDT and trichlorobenzene by some surfactants below and above the critical micelle concentration. Environ. Sci. Technol. 23(7): 832–38. doi:10.1021/es00065a012
- Kim, J.H., J.S. Jurng, G.-N. Bae, J.-K. Jeon, K.Y. Jung, S.-C. Kim, J.-H. Yim, and Y.-K. Park. 2014. Benzene oxidation with ozone at low temperature over an MnOx nanoparticle synthesized by spray pyrolysis. Energy Sources Part A 36:866–73. doi:10.1080/15567036.2010.547923
- Liu L., S.L. Tian, and P. Ning. 2010. Principle and prediction for absorption of toluene by solubilization with Tween-20-containing micelle solutions. China Environ. Sci. 30(5): 615–18 (in Chinese with English abstract).
- Ma, S.B., and B. Wang. 2009. The research progress of organic waste gas treatment technology. Inner Mongolia Environ. Sci. 21(2):55–58 (in Chinese with English abstract). doi:10.3969/j.issn.1007-0370.2009.02.014
- Majumdar, S., D. Bhaumik, K.K. Sirkar, and G. Simes. 2001. A pilot-scale demonstration of a membrane based absorption stripping process for removal and recovery of volatile organic compounds. Environ. Prog. 20:27–35. doi:10.1002/ep.670200113
- Matsuoka, K., R. Yamashita, M. Ichinose, M. Kondo, and T. Yoshimura. 2014. Solubilization of naphthalene and octafluoronaphthalene in ionichydrocarbon and fluorocarbon surfactants. Colloids Surfaces A Physicochem. Eng. Aspects 456:83–91. doi:10.1016/j.colsurfa.2014.04.060
- Myers, D. 1992. Surfactant Science and Technology, 2nd ed., 240–265. New York, NY: Wiley-VCH.
- Park, B., G. Hwang, S. Haam, C. Lee, I. S. Ahn, and K. Lee. 2008. Absorption of a volatile organic compound by a jet loop reactor with circulation of a surfactant solution: Performance evaluation. J. Hazard. Mater. 153:735–41. doi:10.1016/j.jhazmat.2007.09.016
- Pierucci, S., R. D. Rosso, D. Bombardi, A. Concu, and G. Lugli. 2005. An innovative sustainable process for VOCs recovery from spray paint booths. Energy J. 30:1377–86. doi:10.1016/j.energy.2004.02.017
- Poddar, T.K., and K.K. Sirkar. 1996. Henry’s law constant for selected volatile organic compounds in high-boiling oils. J. Chem. Eng. Data 41:1329–32. doi:10.1021/je960064i
- Porter, K.E., S. Sitthiosoth, and J.D. Jenkins. 1991. Designing a solvent for gas absorption. Trans IChemE 69:229–36.
- Roizard, D., F. Lapicque, E. Favre, and C. Roizard. 2009. Potentials of pervaporation to assist VOCs’ recovery by liquid absorption. Chem. Eng. Sci. 64: 1927–35. doi:10.1016/j.ces.2009.01.014
- Stockley, N.J. 1994. VOC abatement by absorption. Eur. Coat. J. 10:751–757.
- Szymczyk, K., M.L. González-Martín, J.M. Bruque, and B. Janczuk. 2014. Effect of two hydrocarbon and one fluorocarbon surfactant mixtures on the surface tension and wettability of polymers. J. Colloid. Interface Sci. 417:180–87. doi:10.1016/j.jcis.2013.11.033
- Tao, Y.S. 1999. Treatment technology for waste gas containing benzene, toluene, and dimethyl benzene. Environ. Protect. 8: 20–24 (in Chinese with English abstract).
- Tian, S.L., L. Liu, and P. Ning. 2010. Phase behavior of tweens/toluene/water microemulsion systems for the solubilization absorption of toluene. J. Solution Chem. 39:457–72. doi:10.1007/s10953-010-9519-8
- Tian, S.L., Y.J. Li, H. Mo, and P. Ning. 2012. Vapour–liquid equilibrium relationship between toluene and mixed surfactants, Environ. Technol. 33:1561–67.doi:10.1080/09593330.2011.635711
- Volodin, N.I., V.M. Puzyreva, and V.E. Soroko. 1997a. Absorption treatment of gases to remove impurities of organic solvents. Russ. J. Appl. Chem. 70:1745–47.
- Volodin, N.I., V.M. Puzyreva, and V.E. Soroko. 1997b. On the problem of extraction of organic solvents from saturated absorbent. Russ. J. Appl. Chem. 70:1985–86.
- Wang, X., R. Daniels, and R.W. Baker. 2001. Recovery of VOCs from high-volume, low-VOC-concentration air streams. AIChE J. 47:1094–100. doi:10.1002/aic.690470516
- Xia,B., S. Majumdar, and K.K. Sirkar. 1999. Regenerative oil scrubbing of volatile organic compounds from a gas stream in hollow fiber membrane device. Ind. Eng. Chem. Res. 38:3462–72. doi:10.1021/ie980657h
- Xiang, Z. Y., Y. C. Lu, X. C. Gong, and G. S. Luo. 2010. Absorption and desorption of gaseous toluene by an absorbent microcapsules column. J. Hazard. Mater. 173:243–48. doi:10.1016/j.jhazmat.2009.08.075
- Yeom, I.T., M.M. Ghosh, and C.D. Cox. 1996. Kinetic aspects of surfactant solubilization of soil-bound polycyclic aromatic hydrocarbons. Environ. Sci. Technol. 30(5): 1589–95. doi:10.1021/es950567t
- You, H. 2014. Tail gas utilization in China. Energy Sources Part A 36:907–13. doi:10.1080/15567036.2010.549909
- Zehraoui, A., A.A. Hassan, and G.A. Sorial. 2012. Effect of methanol on the biofiltration of n-hexane. J. Hazard. Mater. 219–20:176–82. doi:10.1016/j.jhazmat.2012.03.075
- Zhang, J.H., B.X. Shen, J.C. Liu, H. Sun, and W.J. Hu. 2014. Absorption selectivities of solvents for organosulfurs in high sour natural gas. Energy Sourcees Part A 36:822–29. doi:10.1080/15567030903330926
- Zhu, W., and J.X. Liu. 2008. Research progress of technology for removing volatile organic compounds in petrochemical industry. Chem. Ind. Times 22(3): 71–75 (in Chinese with English abstract). doi:10.3969/j.issn.1002-154X.2008.03.023