Abstract
137Cs+/90Sr2+-containing radioactive wastewater is one of the most important problems that the world has been facing with. A by-product, activated porous calcium silicate, is generated at high levels by the pre-desiliconizing and soda-lime-sintering processes for producing Al2O3 from high-alumina fly ash. In order to examine if this by-product could be used as an absorbent for removal of 137Cs+/90Sr2+ from radioactive wastewater, various parameters, such as pH, adsorbent dose, contact time, and initial concentration, were discussed. Results indicated that the equilibrium reached in about 2 hr. Activated porous calcium silicate was highly pH sensitive and able to remove Cs+/Sr2+ in a near-neutral environment. The adsorption equilibrium was best described by Freundlich isotherm equations, and the adsorption of Cs+/Sr2+ was a physical process. The adsorption kinetic data could be better fitted by the pseudo-second-order model, and the adsorption was controlled by multidiffusion. Current study showed that activated porous calcium silicate has a good adsorption of Cs+/Sr2+ for their removal. However, other characteristics, such as selectivity because of coexisting cations, elution and regeneration, thermal stability, and acid resistance, should be discussed carefully before using it in an actual field.
Implications:
Removing 137Cs+/90Sr2+ from radioactive wastewater is one of the tough issues that has been attracting more and more attention world widely, which is the same as fly ash. For recycling high-alumina fly ash, in which Al is extracted to produce Al2O3, a huge amount of activated porous calcium silicate is generated year by year. In this paper, this by-product was successfully used as an absorbent to remove 137Cs+/90Sr2+ from radioactive wastewater for the first time. Factors that affect the absorbability and the mechanisms were discussed in details, providing a possible choice for disposal of 137Cs+/90Sr2+-containing radioactive wastewater.
Introduction
During the last four decades, world nuclear energy consumption has increased more than 37% (Al-Mulali, Citation2014). Nuclear energy has been recognized as an environmentally friendly energy source due to a lower level of carbon emission than fossil fuels (Lee and Jung, Citation2007). However, during the production of nuclear energy, radioactive wastes were produced inevitably (Galambos et al., Citation2009). These wastes caused environmental problems and radiation hazards. Due to the recognition of its importance for the protection of human health, measures for the safety control and minimization of radioactive wastewater is one of the most important problems that are urgent to be solved. In the aspect of radiological safety, 90Sr2+ and 137Cs+ are the major radioactive isotopes because of their relatively long half-lives, high solubility, and biological hazards (Sheha and Metwally, Citation2007).
There are many different methods to remove 137Cs+/90Sr2+ from nuclear wastewater, including chemical precipitation, traditional filtration, ion exchange, extraction, adsorption, and evaporate (Chen and Wang, Citation2008). Extraction and membrane filtration show high stability, high selectivity, and wide adaptation range and have been widely discussed. Sodium tetraphenylboron (NaTPB) is a well-known precipitating agent for Cs+ precipitation, with a poor stability (Anthony et el., Citation1994). Evaporation can reduce the volume of radioactive wastewater efficiently. But this method has many disadvantages, such as high energy consumption, difficulty in operation, and easily causing secondary pollution (El-Kamash, Citation2008). Comparing with other methods, adsorption or ion-exchange technology becomes one of the most commonly used methods due to its simplicity, selectivity, and efficiency (Danilin and Drozhzhin, Citation2007).
To achieve high efficiency, it is important to choose a proper adsorbent. A wide range of materials, natural or synthetic, having different chemical and physical properties, are available for adsorption. Several kinds of adsorbents were investigated, both inorganic and conjugate adsorbent, including carboxymethylate cellulose (CMC) (Wang et al., Citation2009), magnetically modified yeast cells (Ji et al., Citation2010), ferrite (Sheha and Metwally, Citation2007), NiHCF/PTCE (nickel hexacyanoferrate/porous three-dimensional carbon felt; Sun et al., Citation2012), ammonium molybdophosphate (Park et al., Citation2010), and so on (Awual et al., Citation2014). These adsorbents have been widely used owing to their chemical and temperature stabilities and radiation resistance. According to the previously developed adsorbents, crystalline calcium titanate has high specific surface area and large pore volumes. And most important, its crystal channel size is similar to Cs+/Sr2+ ionic radius.
Currently in China, high-alumina fly ash is a tough issue, the total amount of which is over a hundred million tons. Datang International Renewable Resources Development Corporation (Inner Mongolia, China) developed a so-called pre-desiliconizing and soda-lime-sintering technology to recycle high-alumina fly ash to the high value-added product Al2O3. However, this technology also generates activated porous calcium silicate in a huge amount at the same time. Then, opening a new market for this by-product becomes a big problem for this company. Since this by-product is porous with a large surface area, in this study, we tried to solve the two problems together, using this by-product as an absorbent to remove 137Cs+/90Sr2+ (simulated by Cs+/Sr2+) from radioactive wastewater.
In the present work, we conducted batch adsorption experiments to test various parameters, such as pH, adsorbent dose, contact time, and initial concentration. And to interpret adsorption mechanism, we evaluated the adsorption equilibrium, analyzed the thermodynamic and kinetic parameters. In short, the overall goal of this work was to preliminarily evaluate the adsorption of activated porous calcium silicate on Cs+/Sr2+.
Materials and Methods
Materials
Activated porous calcium silicate (surface area: 150 m2/g; average pore diameter: 5–15 nm; density: 1.3–1.4 g/cm3; bulk density: 0.17–0.30 g/cm3) was provided by Inner Mongolia Datang International Renewable Resources Development Corporation. Chemical composition of activated porous calcium silicate is SiO2 of 43.6%, CaO of 44.8%, Fe2O3 of 0.065%, and LOI (loss on ignition) of 10%. Activated porous calcium silicate was characterized by scanning electron microscopy (SEM; S-3000N; Hitachi, Tokyo, Japan) and X-ray diffraction (XRD; D/MAX2500; Rigaku, Almelo, Holland). The results are presented in and . SEM results show that activated porous calcium silicate particles is honeycomb-shaped, bedded. XRD analysis indicates that the main phase of activated porous calcium silicate is CaSiO3·xH2O and there is no sign for CaO, Ca(OH)2, and SiO2.
Adsorption experiments
For a typical experiment, 20 mL of Cs+/Sr2+ solution was mixed with 100 mg activated porous calcium silicate. The bottles were shaken at 100 rpm. And the examined Cs+/Sr2+ initial concentration, contact time, and temperature were at 50–500 mg/L, 2–36 hr, and 15–45 °C. The effect of pH was studied in the range of 1–14, which was adjusted by 0.1 M HCl or 0.1 M NaOH. After each experiment, the sample was centrifuged, filtered, and finally analyzed by inductively coupled plasma optical emission spectrometry (ICP-OES; OPTIMA 2000; PerkinElmer, Waltham, MA, USA). Each experiment was preformed twice, and mean value was given in each table or figure without an error bar. The equilibrium adsorption capacity (qe), partition coefficient (Kd), and adsorption ratio (η) were calculated using the following equations:
Results and Discussion
Effect of pH
The effect of pH on removing Cs+/Sr2+ by activated porous calcium silicate is presented in . It is known that calcium silicate will be dissolved at a low pH value, decreasing adsorption active sites. Thus, in this condition, Cs+/Sr2+ sorption efficiency would be significantly low.
Figure 3. Effect of pH on Kd of Cs+/Sr2+ (50 mg/L Cs+/Sr2+ 20 mL, adsorbent: 100 mg activated porous calcium silicate, 2 hr).
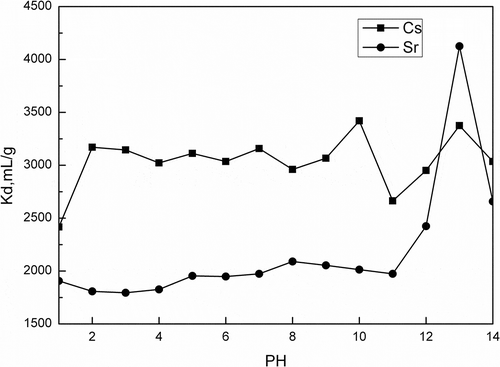
As shown in , when pH increases from 2 to 8, Kd(Sr2+) increases from 1900 to 2124.95 mL/g. The total Sr2+ uptake at acidic pH values is mainly attributed to sorption. At acidic pH values, the removal of metal ion is inhibited due to the presence of H+ ions that compete with Sr2+ ions for the adsorption sites (Awual et al., Citation2014). The total Sr2+ uptake also increases sharply from 1900 to 4124.95 mL/g when pH consecutively increases from 11 to 13. The total Sr2+ uptake at this stage is mainly attributed to precipitation and more adsorption active sites than acidic pH ranges. It can also be seen from that the adsorption capacity of Sr2+ at pH 14 is dramatically decreased. Sr(OH)+ is dominant at pH values above 13 (Park et al., Citation2010); OH− makes Sr2+ convert into Sr(OH)+. However, the effect of pH on total Cs+ uptake is quite different; Kd(Cs+) is nearly constant at all pH ranges, slightly increases from 2416.13 to 3100 mL/g. As we know, the change of pH affects the adsorptive process through dissociation of functional groups on the active sites of the adsorbent. The surface charge and functional group activity of adsorbents could vary with the changing of solution pH. However, cesium does not hydrolysis or form complexes readily, this is because Cs exists as Cs+ at all pH ranges (Awual et al., Citation2014). The total Cs+ uptake at acidic pH values is suppressed due to the excessive protons competing with Cs+ for the available sorption sites. On the other hand, most of the surface sorption sites are deprotonated at high pH region, this greatly increases electrostatic interactions to take up Cs+ with extreme high efficiency (Park et al., Citation2010). Current study shows that activated porous calcium silicate is highly pH sensitive and able to remove Cs+/Sr2+ in neutral pH region.
Effect of adsorbent dosage
The effect of adsorbent dosage on the removal of Cs+/Sr2+ by activated porous calcium silicate is presented in . As shown in , the removal rate of Cs+/Sr2+ increases respectively from 0% to 35.46% and 88.38% when the adsorbent dosage increases from 0 to 200 mg. Increase in adsorption with adsorbent dosage can be attributed to the increase of adsorbent surface area and adsorption sites. When the adsorbent dosage is more than 200 mg, the removal rate of Cs+ does not decrease. The probable reason for the decrease of adsorption capacity is the saturation of the adsorption active sites that are involved in the adsorption process (Ngah and Fatinathan, Citation2010).
Effect of contact time
The effect of contact time on the removal of Cs+/Sr2+ by activated porous calcium silicate is presented in . As shown in , at the first 30 min, Cs+/Sr2+ removal is in the rapid phase. This is probably caused by the distribution of high-quality porous surface on activated porous calcium silicate. From 30 min to 2 hr, Cs+/Sr2+ sorption efficiency gradually decreases with time until saturation is attained. It is indicated that Cs+/Sr2+ transports through much more direct and facile diffusion to network sites (Awual et al., Citation2014). The reason of an insignificant enhancement in adsorption in 36 hr compared with that in 2 hr could be the aggregation of Cs+/Sr2+ ions and the intraparticle diffusion of Cs+/Sr2+ into the interior pores of activated porous calcium silicate. Since the difference in the adsorption values at 2 hr and at 36 hr is small, further experiments were conducted at 2 hr.
Effect of initial concentration
Cs+/Sr2+ initial concentration plays an important role in the adsorption capacity. shows the effect of initial concentration on the removal of Cs+/Sr2+ by activated porous calcium silicate. The adsorption capacity of activated porous calcium silicate increases rapidly as the increase of initial concentration. Adsorption equilibrium concentration of Cs+/Sr2+ increases from 1.92 and 0.58 mg/L to 36.65 and 23.65 mg/L, respectively, when the initial concentration increases from 0.5 to 50 mg/L. This result shows that the initial ions concentration provide a driving force to overcome the mass transfer resistances between the adsorption medium and adsorbent (Fan et al., Citation2008). In addition, with the increase of Cs+/Sr2+ concentration, the number of collisions between Cs+/Sr2+ and adsorbent increases, which enhances the adsorption process.
Adsorption isotherm
To optimize an adsorption system, it is important to establish the most appropriate equilibrium correlation. Adsorption isotherms describe the equilibrium concentration of adsorbent in liquid at a constant temperature. In order to establish the best-fit model, Langmuir (Langmuir, Citation1918) and Freundlich (Freundlich, Citation1906) equations were employed.
Langmuir adsorption isotherm assumes that adsorption takes place at specific homogeneous sites within the adsorbent (Langmuir, Citation1918) and has been successfully applied in many sorption processes. The Langmuir equation is as follows:
Equation 4 can be rearranged to linear form:
The values of Langmuir constants are listed in . The maximal sorption capacity for Cs+/Sr2+ calculated from Langmuir model by activated porous calcium silicate is 163.9 and 131.57 mg/g, respectively. Such high capacity of activated porous calcium silicate indicates that activated porous calcium silicate is highly effective for Cs+/Sr2+ decontamination. shows that the correlation coefficient (R2) are low (R2 = 0.882–0.913), suggesting that the model is not suitable for describing the adsorption system.
Table 1. Adsorption equilibrium data using Langmuir and Freundlich isotherm models
Freundlich model is derived to describe the multilayer sorption and generally used for the sorption on heterogeneous surfaces (Freundlich, Citation1906). The Freundlich equation is as follows:
The logarithmic form of eq 6 is given as follows:
Comparison with different materials
A comparison has been made between activated porous calcium silicate and previously reported sorbents for Cs+/Sr2+ sorption (). The sorption capacity for Cs+/Sr2+ is 163.9 and 131.57 mg/g, respectively. The comparison data of qm show that activated porous calcium silicate outperforms many other sorbents. Base on this consideration, activated porous calcium silicate has a good sorption capacity for the removal of Cs+/Sr2+ from radioactive wastewater.
Table 2. Comparison of sorption capacity for Cs+ /Sr2+ by using various adsorbents
Adsorption thermodynamics
Adsorption thermodynamic data provide information about adsorption mechanism and also the inherent energy change. Thermodynamic parameters, i.e., free energy (ΔG°; kJ/mol), enthalpy (ΔH°; kJ/mol), and entropy (ΔS°; kJ/mol·K), are calculated using eqs 8–10 (Singh et al., Citation2003) and are given in .
Table 3. Thermodynamic parameters for the adsorption Cs+/Sr2+ by activated porous calcium silicate
The change in enthalpy (ΔH°) for both Cs+ and Sr2+ is positive, which indicates that the adsorption of Cs+ and Sr2+ is endothermic. The positive values of the entropy changes (ΔS°) show the increased randomness at the interface of adsorbent and also indicate an affinity of the adsorbent toward Cs+/Sr2+ (Mohan et al., Citation2002). A negative ΔG° value confirms the spontaneous nature of this system. The absolute value of ΔG° is smaller than 20 KJ/mol, indicating that physical adsorption is dominating (Ding et al., Citation2012). As chemisorption is not the main process, high Cs+/Sr2+ removal rate is mainly dependent on high surface area and large pore volumes of activated porous calcium silicate. It is also indicated that activated porous calcium silicate would not undergo any structural changes, since this process is mainly physical.
Adsorption kinetics
Adsorption kinetics describes the adsorption rate, which defines the efficiency of adsorption. The adsorption dynamics can be described by the following three consecutive steps (Ahmaruzzaman, Citation2010):
transport of solute from fluid body through liquid film or boundary layer to adsorbent exterior surface—external diffusion;
solute diffusion from adsorbent exterior surface into pore of adsorbent—intraparticle transport; and
adsorption of solute on interior surface of the pores and capillary spaces of adsorbent.
For the three steps, the third step is rapid and negligible. The overall rate of adsorption will be controlled by the slowest step that would be either external diffusion or intraparticle diffusion. In order to investigate the adsorption process of Cs+/Sr2+ on the adsorbents, pseudo-first-order (Ho and McKay, Citation1998), pseudo-second-order (Ho and McKay, Citation2000), and intraparticle (Ho and McKay, Citation1999) diffusion kinetic models were used, which are expressed by eqs 11–13.
To understand the diffusion mechanism of Cs+/Sr2+ adsorption by activated calcium silicate, the intraparticle diffusion model was used to predict the rate-limiting step. If the plot shows multilinear characteristic or does not pass the origin, the adsorption process is controlled by two or more diffusion mechanisms (Vasiliu et al., Citation2011). As shown from , such deviation, two separated lines and not passing the origin, indicates that the intraparticle diffusion is not a sole rate-limiting step. The adsorption is controlled by multiple diffusion steps (Huang et al., Citation2011), involving film diffusion and intraparticle diffusion. The results also reveal that the rate constants (ki) of the first stage and the second stage () of Cs+ are not very different, suggesting both film diffusion and intraparticle diffusion may play an important role in the adsorption kinetics; for Sr2+ the rate constant (ki) of the second stage is much higher than the first stage, suggesting that film diffusion play a more important role than intraparticle diffusion in the adsorption.
Table 4. The kinetic parameters of intraparticle diffusion model
Summary
The present study shows that activated porous calcium silicate can successfully remove Cs+/Sr2+. What should be pointed out is that we need to check the selectivity in the presence of K, Na, Ca, Mg, and Ba, since they coexist in the nuclear waste samples. Furthermore, elution and regeneration operations need to be performed to make the material cost-effective. Other examinations such as thermal stability and acid resistance should also be discussed before using it in a real field. The following conclusions can be drawn from the current experiments:
Activated porous calcium silicate showed a high Cs+/Sr2+ removal rate in neutral pH region, and the equilibrium was reached in about 2 hr. Activated porous calcium silicate adsorption capacity increased with the increase of time, calcium silicate adding amount, and Cs+/Sr2+ initial concentration.
The adsorption equilibrium was best described by Freundlich isotherm equations. Comparison with other sorbents, activated porous calcium silicate was a promising alternative for Cs+/Sr2+ removal.
Adsorption thermodynamics indicated that Cs+/Sr2+ removal by activated porous calcium silicate was a physical adsorption process that was endothermic. And this process happened spontaneously.
The adsorption kinetics could be well fitted by pseudo-second-order model and the adsorption process was controlled by multidiffusion, involving film diffusion and intraparticle diffusion.
Funding
This work was financially supported by the National Natural Science Foundation of China, funding number 21007052.
Additional information
Funding
Notes on contributors
Yingying Chu
Yingying Chu is a master candidate working on solid waste treatment and resource recycle.
Rong Wang
Rong Wang is a lecturer on nuclear waste control and prevention.
Mengjun Chen
Mengjun Chen is a Ph.D. and associate professor with a focus on solid waste treatment and resource recycle.
References
- Ahmaruzzaman, M. 2010. A review on the utilization of fly ash. Prog. Energy Combust. 36:327–363. doi:10.1016/j.pecs.2009.11.003
- Al-Mulali, U. 2014. Investigating the impact of nuclear energy consumption on GDP growth and CO2 emission: A panel data analysis. Prog. Nucl. Energy 73:172–178. doi:10.1016/j.pnucene.2014.02.002
- Anthony, R.G., R.G. Dosch, D. Gu, and C. Philip. 1994. Use of silicotitanates for removing cesium and strontium from defense waste. Ind. Eng. Chem. Res. 33:2702–2705. doi:10.1021/ie00035a020
- Awual, Md. R., S. Suzuki, T. Taguchi, H. Shiwaku, Y. Okamoto, and T. Yaita. 2014. Radioactive cesium removal from nuclear wastewater by novel inorganic and conjugate adsorbents. Chem. Eng. J. 242:127–135. doi:10.1016/j.cej.2013.12.072
- Chen, C., and J. Wang. 2008. Removal of Pb2+, Ag+, Cs+, and Sr2+ from aqueous solution by brewery’s waste biomass. J. Hazard. Mater. 151:65–70. doi:10.1016/j.jhazmat.2007.05.046
- Danilin, L.D., and V.S. Drozhzhin. 2007. Inorganic sorbents based modified microspheres for the treatment of liquid radioactive waste. Radiochemistry 49:319–322. doi:10.1134/S1066362207030204
- Ding, L., H.P. Deng, C. Wu, and X. Han. 2012. Affecting factors, equilibrium, kinetics and thermodynamics of bromide removal from aqueous solutions by MIEX resin. Chem. Eng. J. 181:360–370. doi:10.1016/j.cej.2011.11.096
- El-Kamash, A.M. 2008. Evaluation of zeolite a for the sorptive removal of Cs+ and Sr2+ ions from aqueous using batch and fixed bed column operations, J. Hazard. Mater. 151:432–445. doi:10.1016/j.jhazmat.2007.06.009
- Fan, T., Y.G. Liu, and B.Y. Feng. 2008. Biosorption of cadmium(II), zinc(II) and lead(II) by Penicillium simplicissimum: Isotherms, kinetics and thermodynamics. J. Hazard. Mater. 160:655–661. doi:10.1016/j.jhazmat.2008.03.038
- Freundlich, H.M.F. 1906. Uber die adsorption in losungen. Z. Phys. Chem. 57:385– 470.
- Galambos, M., J. Kufcakova, and P. Rajec. 2009. Sorption of strontium on slovak betonites. J. Radioanal. Nucl. Chem. 281:347–357. doi:10.1007/s10967-009-0017-7
- Ho, Y.S., and G. McKay. 1998. The kinetics of sorption of basic dyes from aqueous solution by Sphagnum moss peat. Can. J. Chem. Eng. 76:822–827. doi:10.1016/S0043-1354(98)00207-3
- Ho, Y.S., and G. McKay. 1999. Competitive sorption of copper and nickel ions from aqueous solution using peat. Adsorption 5:409–417. doi:10.1023/A:1008921002014
- Ho, Y.S., and G. McKay. 2000. The kinetics of sorption of divalent metal ions onto sphagnum moss peat. Water Res. 34:735–742. doi:10.1016/S0043-1354(99)00232-8
- Huang, J.H., R.J. Deng, and K.L. Huang. 2011. Equilibria and kinetics of phenol adsorption on a toluene modified hyper cross linked poly(styrene codivinylbenzene) resin. Chem. Eng. J. 171:951–957. doi:10.1016/j.cej.2011.04.045
- Ji, Y.Q., Y.T. Hu, Q. Tian, X.Z. Shao, J.Y. Li, M. Safarikova, and I. Safarik. 2010. Biosorption of strontium ions by magnetically modified yeast cells. Sep. Sci. Technol. 45:1499–1504. doi:10.1080/01496391003705664
- Langmuir, I. 1918. The adsorption of gases on plane surfaces of glass, mica and platinum. J. Am. Chem. Soc. 40:1361–1402. doi:10.1021/ja02242a004
- Lee, Y.E., and Y.B. Jung. 2007. Reliable role of nuclear power generation under CO2 emission constranints. Nucl. Eng. Technol. 39:655–662. doi:10.5516/NET.2007.39.5.655
- Mohan, D., K.P. Singh, G. Sing, and K. Kumar. 2002. Removal of dyes from wastewater using fly ash, a low-cost adsorbent. Ind. Eng. Chem. Res. 41:3688–3695. doi:10.1021/ie010667+
- Ngah, W.S.W., and S. Fatinathan. 2010. Adsorption characterization of Pb(II) and Cu(II) ions onto chitosan–tripolyphosphate beads: Kinetic, equilibrium and thermodynamic studies. J. Environ. Manage. 91:958–969. doi:10.1016/j.jenvman.2009.12.003
- Park, Y., Y.C. Lee, W.S. Shin, and S.J. Choi. 2010. Removal of cobalt, strontium and cesium from radioactive laundry wastewater by ammonium molybdophosphate polyacrylonitrile (AMP–PAN). Chem. Eng. J. 162:685–695. doi:10.1016/j.cej.2010.06.026
- Sheha, R.R., and E. Metwally. 2007. Equilibrium isotherm modeling of cesium adsorption onto magnetic matelicals. J. Hazard. Mater. 14:354–361. doi:10.1016/j.jhazmat.2006.09.041
- Singh, K.P., D. Mohan, S. Sinha, G.S. Tondon, and D. Gosh. 2003. Color removal from wastewater using low-cost activated carbon derived from agricultural waste material. Ind. Eng. Chem. Res. 42:1965–1976. doi:10.1021/ie020800d
- Sun, B., X.G. Hao, Z.D. Wang, G.Q. Guan, Z.L. Zhang, Y.B Li, and S.B. Liu. 2012. Separation of low concentration of cesium ion from wastewater by electrochemically switched ion exchange method: Experimental adsorption kinetics analysis. J. Hazard. Mater. 30:177–183. doi:10.1016/j.jhazmat.2012.07.010
- Vasiliu, S., I. Bunia, S. Racovita, and V. Neagu. 2011. Adsorption of cefotaxime sodium salt on polymer coated ion exchange resin microparticles: Kinetics, equilibrium and thermodynamic studies. Carbohydr. Polym. 85:376–387. doi:10.1016/j.carbpol.2011.02.039
- Wang, M., L. Xu, J. Peng, M.L. Zhai, J.Q. Li, and G.S. Wei. 2009. Adsorption and desorption of Sr(II) ions in the gels based on polysaccharide derivates. J. Hazard. Mater. 27:820–826. doi:10.1016/j.jhazmat.2009.06.071