Abstract
Few studies have been made regarding carbonyl concentrations in Monterrey, México. The Monterrey Metropolitan Area (MMA) has the third largest population in the country and has increasing pollution issues. The concentrations of 10 aldehydes and two ketones were measured in the MMA, in the spring and fall of 2011 and 2012. Formaldehyde (16–42 ppbv) was the most abundant carbonyl, followed by acetaldehyde (5–15 ppbv) and acetone (7–15 ppbv). The concentrations showed marked diurnal trends with maximum values between 10:00 a.m. and 2:00 p.m., when photochemical activity is intense. Thus, secondary production of carbonyls is statistically significant in the city. Biogenic production of several carbonyls, such as 2-butanone, was supported by their mid correlation with solar radiation and low correlation with propionaldehyde, which is mainly emitted by anthropogenic sources. The seasonal variability of the concentrations was observed in the first three samplings, with the highest levels reached in the fall. The rainy conditions during the fourth sampling did not allow comparison. Carbonyl–NOx–O3 analysis was made. Results indicated a carbonyl-sensitive atmosphere, especially during the midday samplings of 10:00 a. m. to 2:00 p.m. and 2:00 p.m. and 6:00 p.m. because of the intense solar radiation during these periods.
Implications: Monitoring of carbonyls in Monterrey, Mexico, was performed to quantify the pollutant concentration in the city’s atmosphere. Although primary emission is significantly important, the secondary production of the pollutants, along with ozone production being carbonyl sensitive, indicates that air pollution controls must address the direct sources and the precursors of the pollutants to achieve air quality.
Introduction
Carbonyls are common components of urban atmospheres. Carbonyls are emitted directly into the environment by anthropogenic sources such as automotive vehicles, coal-fired power plants, trash burning, and industrial processes (Possanzini et al., Citation2002; Ho et al., Citation2012; Villanueva et al., Citation2013). Sources can also be natural (Cerón et al., Citation2005; Villanueva et al., Citation2013); for example, forests emit formaldehyde, acetaldehyde, and acetone (Villanueva-Fierro et al., Citation2004; Cojocariu et al., Citation2005). These are also produced in the atmosphere from the photo-oxidation of atmospheric hydrocarbons (Altshuller, Citation1993; Carter, Citation1994; Atkinson et al., Citation1999; Possanzini et al., Citation2002).
Despite their short residence time, barely 4 hr for formaldehyde (photolysis) and 8 hr for acetaldehyde (reaction with OH) (Atkinson, Citation2000), carbonyls participate in multiple reactions, promoting the secondary production of peroxyacetyl nitrate (PAN), secondary organic aerosol, and ozone (O3) (Altshuller, Citation1993; Possanzini, Citation1996).
In addition, carbonyls have adverse effects on health. Some are toxic or carcinogenic (World Health Organization [WHO], Citation2000; García-Reynoso et al., Citation2007). For example, formaldehyde causes irritation to the respiratory system and cancer (Ho et al., Citation2002), and acetaldehyde irritates the eyes, the mucosa, and the skin (Viskari et al., Citation2000). Hence, the quantification of the pollutants is of interest.
Carbonyls have been quantified in industrialized and developing countries (Báez et al., Citation2001; Ho et al., Citation2002; Cerón et al., Citation2007; Dai et al., Citation2012; Duan et al., Citation2012; Ho et al., Citation2012; Villanueva et al., Citation2013). Formaldehyde, acetaldehyde, and acetone are the most common carbonyls in the atmosphere (Ho et al., Citation2012; Villanueva et al., Citation2013). The diurnal and seasonal variations have also been studied (Cerón et al., Citation2007; Wang et al., Citation2007; Lu et al., Citation2010; Herrera-Murillo et al., Citation2012; Ho et al., Citation2012). In Mexico, carbonyl concentrations have been measured mainly in Mexico City (Báez et al., Citation2000; Báez et al., Citation2001; Grutter et al., Citation2005). Concentrations of the most abundant carbonyls, formaldehyde (33 ppbv) and acetaldehyde (23 ppbv), have been measured (Báez et al., Citation2000; Grutter et al., Citation2003). Some studies have also been made in Xalapa (Báez et al., Citation2001), Campeche (Cerón et al., Citation2007), and the forest areas of the State of Veracruz (Cerón-Bretón et al., Citation2005). Information about carbonyls is limited in Monterrey, Mexico (Facundo-Torres et al., Citation2012), so this work seeks to quantify carbonyls in the atmosphere of Monterrey, Mexico, and to describe diurnal and seasonal variation and their effects on ozone (O3) formation. Ozone is an important pollutant in Monterrey with high concentrations that often exceed the hourly average of 0.110 ppm set by the Mexican Standard NOM-020-SSA-1993. O3 concentration was higher than the regulation limit for 30 days (sum of all monitoring stations) in 2013 according to the Sistema Integral de Monitoreo Ambiental (SIMA: Integrated Environmental Monitoring System) and has been over the limit for 20 days up to September 2014 (SIMA, Citation2014a; SIMA, Citation2014b).
Methodology
General description of the sampling site
Sampling was done in the city of Monterrey, Mexico. The Monterrey Metropolitan Area (MMA; ) is the third most populated in the country, with 3.9 million people in an area of 5,346.80 km2. The municipalities of Apodaca, General Escobedo, García, Guadalupe, Juárez, Monterrey, San Nicolás de los Garza, San Pedro Garza García, and Santa Catarina comprise this metropolis. The MMA is located in a valley with an altitude of 540 m and is surrounded by mountains that act as natural barriers to the wind, which is predominantly from the east.
The city has a high number of industrial complexes and more than 1.89 million vehicles, so pollution levels are high. For example, the official Mexican regulations for O3 and particles with a diameter of less than 10 µm (PM10) were exceeded during 17 and 90 days in 2007, respectively (SEMARNAT, Citation2012). Furthermore, Gonzalez-Santiago et al. (Citation2011) found concentration levels of particles with a diameter less than 2.5 µm (PM2.5) constantly higher than the 50 µg/m3 established in the standard NOM-025-SSA1-1993, with average concentrations of up to 124 µg/m3 in the area of Santa Catarina.
Sampling site
Considering the supersites particulate matter (PM) monitoring system of the U.S. Environmental Protection Agency (EPA, Citation1998), the number of repetitions in the experiment is vital for statistical analysis, attribution of sources, and subsequent preparation of emission standards; thus, sampling was done at a single point. Samples were taken at the Obispado station (center) of the SIMA (), located in the southeast of downtown Monterrey at an altitude of 556 m above sea level, with the geographical coordinates: 25° 40’ 32’’ N, 100° 20’ 18’’ W. The station is surrounded by main avenues () that carry heavy traffic flow; for example, Gonzalitos Ave. has an average 7000 vehicles/hr (Villasáez, Citation2014) and Constitución Ave. has 20,000 vehicles/hr (SDU, Citation2014).
The choice of the Obispado station from among the five SIMA monitoring stations available at the beginning of the investigation was based on a spatial homogeneity analysis of carbon monoxide (CO) and O3 concentrations. Variation of CO concentration helps to establish possible primary sources of pollution, while O3 is an indicator of reactivity in the atmosphere. Divergence coefficient was used (Pinto et al., Citation2004):
The compounds concentrations from 2006 to 2009 were analyzed. CO CODs are presented in . The northeastern and southeastern stations had the lowest COD value when compared with the center station. The stations to the west of the city had a low COD between each other; however, when compared to the eastern stations, pollution was divergent (COD ~0.5). Therefore, the center station was selected.
Table 1. CO CODs
Wind roses were made for each monitoring stations for 2006–2010 to support the selection of the sampling site. Three wind direction graphs are shown in . For the southeastern and northwestern stations, the predominant direction was from the east, while for the center station the predominant direction was from the east/northeast. In general, the winds arriving at the different monitoring stations originate in same location, which provides a similar background. Furthermore, the east-to-west transport of pollutants and the city’s natural barriers cause pollutant concentration divergence to increase when monitoring points are farther away. These observations support the selection of the center monitoring station.
Sampling
Four sampling campaigns consisting of seven nonconsecutive days in 2 weeks were made on the following dates: May 28 to June 9 and October 23 to November 3, 2011; and June 6 to 18 and October 13 to 25, 2012. Campaign date selection was made according to the days with historically high ozone concentration. Sampling was done in 4-hr intervals from 6:00 a.m. to 10:00 p.m. For each campaign, 28 samples and two field blanks were collected.
Sampling was done following the EPA (Citation1999) TO11 method. We used a Thomas pneumative compressor/vacuum pump (60 Hz, 115 V), a calibrated flowmeter, Waters (USA) Sep-Pak DNPH-Silica cartridge, and a heated ozone scrubber, to prevent the degradation of dinitrophenylhydrazine (DNPH) (Báez et al., Citation2000; Possanzini et al., Citation2002; Grutter et al., Citation2003; Cerón-Bretón et al., Citation2005). The scrubber was prepared for every campaign by (i) cleaning the copper tube with deionized water for 15 min, (ii) filling the tube for 15 min with a saturated solution of potassium iodide (CTR, 99% purity), and (iii) drying the denuder with a flow of 1 L/min of nitrogen (AOC, high-performance liquid chromatography [HPLC] grade) during 4 hr. The denuder was sealed until use and between sampling days in the campaigns. During sampling, the scrubber inner temperature was maintained at 86°C to prevent air humidity from flushing the DNPH derivatives. Flow rates were set at 1 L/min. Rate fluctuation, measured for 10 min at the beginning and end of the interval, was between 0 and 5%. Leak checks were performed at the beginning and end of the interval.
Sampled cartridges were sealed, labeled, wrapped in aluminum foil, and stored at a temperature below 4°C until chemical analysis.
Chemical analysis
Following the TO-11 method (EPA, Citation1999), cartridges were eluted with 5 mL of HPLC-grade acetonitrile. Subsequently, 20-μL aliquots of the solutions were analyzed in an HPLC Shimadzu LC-10AD with a UV-Vis-SPD-10AV detector, adjusted to 360 nm, using an Allure AK analysis column. The mobile phase had a ratio of 60:40 acetonitrile/water up to 100:0. The analysis flow was of 1 mL/min.
Quality assurance and quality control
Solvents used were HPLC grade and were tested prior to each analysis. Two field blanks, two laboratory blanks, and two spiked samples were analyzed for every campaign. Field blanks were taken at the 10:00 a.m. to 2:00 p.m. interval on the third and sixth days of each campaign. Laboratory blanks were opened and sealed upon sample arrival to the laboratory. Samples were spiked prior to sample elution. The instrument was calibrated using five standard solutions (from 0.05 µg/mL up to 1.25 µg/mL). A kit of derivatives containing acetaldehyde, acetone, propenal, benzaldehyde, 2-butanone, crotonaldehyde, formaldehyde, hexaldehyde, isobutyraldehyde, propionaldehyde, o-toluenaldehyde, m-toluenaldehyde, p- toluenaldehyde, and valeraldehyde was used (Chem Service, Inc., ~99% purity).
Cartridge collection efficiency was determined using two cartridges connected in series; more than 95% of carbonyls were found on the first cartridge.
Statistical analysis
Compound concentrations over the method detection limits were classified as general, comprising all the information, by season of the year (spring and fall), by sampling campaign (in chronological order: 1, 2, 3, and 4), and by time interval (6:00 a. m., 10:00 a.m., 2:00 p.m., and 6:00 p.m.). Using XL-Stat 2013 software, descriptive statistics (mean, variance, standard deviation) were calculated, and discriminant analysis was performed to observe possible significant differences (α = 0.05) between the groups. The parameters used were tolerance of 0.0001 and significance level of 5%, no validation or prediction models were used, and observations with missing data were eliminated. Dependent variables (y) were defined as the groups and classes (e.g., sampling number), and independent variables (x) as the data obtained from the sampling for each compound. Once the results were obtained, the p-value of Fisher’s square distance was used as a criterion to accept the significant differences among the groups.
Results and Discussion
Carbonyl seasonal variation
In total, 112 samples were collected in this study: 28 for each campaign. Concentrations over the method minimum detection limits of 10 carbonyls (eight aldehydes and two ketones) were found in the first three sampling campaigns. In fall 2012, five aldehydes and two ketones were found. The average carbonyl concentrations for each monitoring campaign appear in . Missing data were eliminated and o,m,p-toluenaldehyde was reported as a single compound.
Table 2. Carbonyl concentrationsa (ppbv)
presents the meteorological conditions observed during the campaigns. Spring monitoring was carried out just prior to the start of summer of each year.
Table 3. Meteorological conditions in Monterrey during the sampling periods
Formaldehyde was the most abundant carbonyl (16–42 ppbv) in each sampling period. Acetaldehyde (5–16 ppbv) and acetone (7–15 ppbv) also had high concentrations. The highest average concentrations occurred in fall 2011, except for 2-butanone, which reached its maximum average (5 ppbv) in spring 2012, and valeraldehyde, for which the maximum (1.2 ppbv) was reached in fall 2012. Benzaledhyde, toluenaldehydes, and hexaldehyde were below the method’s detection limits in over 75% of the samples in fall 2012; wet deposition caused by the rainy conditions during the monitoring is the most probable cause (Finlayson-Pitts and Pitts, Citation2000; Christensen, 2000).
The concentrations of formaldehyde, in all the samplings, and propionaldehyde, in three of the monitoring campaigns, were higher than those obtained in Mexico City by Báez et al. (Citation2002) (~15 ppbv and 1 ppbv, respectively), while acetaldehyde and acetone presented concentrations similar to those reported in the same study. In comparison with the results of Herrera-Murillo et al. (Citation2012) in San José, Costa Rica, Dai et al. (Citation2012) in Xi’an China, and Morknoy et al. (Citation2011) in Bangkok, Thailand, aldehydes presented higher concentrations, while ketones had a similar behavior in Monterrey.
In general, the sampling campaigns were significantly different from each other in accordance with the discriminant analysis for the general class (pvalue < 0.0001). The differences between the spring and fall concentrations were expected due to the effects of the differences in temperature and rain between both seasons. The highest pollutant concentrations were reached in fall 2011, followed by spring 2012 and spring 2011. Except for 2-butanone, compounds had at least one case with no significant differences between the sampling campaigns. For example, formaldehyde had similar average concentrations in both spring programs, while acetaldehyde had the same averages in the first and fourth campaigns.
During fall 2011, the concentrations of the carbonyls were between 38% and 700% higher than those obtained in the spring. Báez et al. (Citation2000) had a similar result, with increased concentrations in the fall (November 1993 vs. June 1995), although with lower percentages, between 288% and 480%, in Mexico City. The compression of the inversion layer, as an effect of the decrease in temperature (4°C), could be the cause of the result found. This is evidenced with an increased correlation between CO and temperature during fall seasons (0.6–0.8) with respect to spring (0–0.6). A similar situation was expected for fall 2012. However, the last monitoring campaign had an average probability of rainfall of 60%. The sky was cloudy most of the time, and solar radiation decreased by 30% (from 0.672 to 0.475 kW/m2), diminishing secondary production of carbonyls, in comparison with fall 2011. Additionally, the wet deposition of carbonyls is an important sink, so rain during the sampling period could be a second cause of lower concentrations with respect to fall 2011 (Yu et al., Citation2008).
Formaldehyde/acetaldehyde (FA/AA) ratios and acetaldehyde/propionaldehyde (AA/PA) ratios have been used to describe the atmosphere as urban or rural areas. Urban areas are characterized by FA/AA values between 1 and 2 (Possanzini et al., Citation1996), and values close to 10 characterize rural areas, since photo-oxidation of isoprene produces a higher quantity of formaldehyde in comparison with acetaldehyde. The AA/PA ratio with values of 1.0 to 8.4 is characteristic of urban areas (Ho et al., Citation2002; Lu et al., Citation2010). The FA/AA ratios obtained in this investigation were 5.9, 2.7, 2.8, and 3.9 for the campaigns, in chronological order. The value obtained for spring 2011 is intermediate between urban and rural areas; therefore, it is possible that the variation in the concentrations of formaldehyde, and other carbonyls, is due to pruning and burning plants (Possanzini et al., Citation2007; Villanueva et al., Citation2004) observed during the sampling days near the monitoring station. The rest of the ratios are characteristic of an urban area. In contrast, Facundo-Torres et al. (Citation2012) found an FA/AA ratio of 1.9 during spring 2011 in Monterrey. The AA/PA values were 8.9, 6.5, 8.6, and 7.9, in chronological order of the samplings, which are in the range of values typical of urban areas.
In general, correlation analysis between carbonyls noted a lower correlation between the pollutants during fall campaigns. A specific analysis of carbonyl-propionaldehyde (PA) gave evidence of higher correlation values with FA, acetone, and benzaldehyde (0.8–0.9) during fall than in spring (0.5–0.8). Most emissions of PA are produced by anthropogenic sources; thus, during fall campaigns anthropogenic sources have greater importance for the three carbonyls. The sampling location is surrounded by main streets (); thus, mobile sources are among the main anthropogenic emitters of carbonyls. Additionally, when observing the general FA/AA ratios, during spring season (4.4, general averaged ratio) there is more carbonyl production by photooxidation than in fall (3.4). Pang and Mu (Citation2006) found a similar result when studying secondary production in Beijing, China. Further source analysis will be assessed in future work.
Diurnal variation and correlation with meteorological variables
shows the average diurnal variation of the three most abundant carbonyls in the Monterrey atmosphere: formaldehyde, acetaldehyde, and acetone. Averages include data of the four campaigns. Propionaldehyde is included because it describes urban atmospheres as it is primarily emitted by anthropogenic sources (Possanzini et al., Citation1996). Variations of the aldehydes, except crotonaldehyde, were similar to those presented in the figure. Ketones did not present similar variations.
Figure 4. Average diurnal variation of (a) formaldehyde, (b) acetaldehyde, (c) acetone, and (d) propionaldehyde. Concentration (ppbv) is read from the left of the graph, time interval (hours) from the bottom. Figure presents mean (⌖), median (line), and outliers (*).
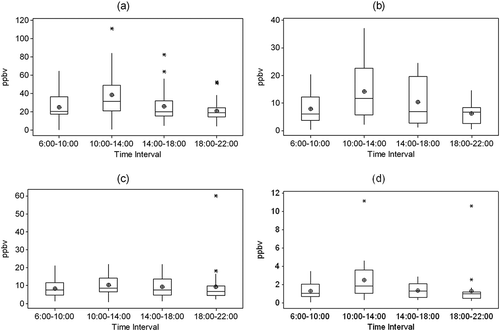
Volatile organic compound (VOC) chains break in the presence of ultraviolet energy, producing secondary aldehydes (Cerón et al., Citation2007). The concentrations of formaldehyde, acetaldehyde, and propionaldehyde were significantly higher between 10:00 a. m. and 2:00 p. m. than in the rest of the intervals (). These results agree with those found by Facundo-Torres et al. (Citation2012), who found higher concentrations of carbonyls during morning (8:00 a. m. to 12:00 p. m.) than in the afternoon (3:00 p.m. to 7:00 p.m.) at one location in the MMA. The concentration increase occurred during the interval with the largest amount of solar radiation () and increased production of O3 (), while CO () concentration remained constant, evidence that a fraction of the carbonyl concentration is caused by the secondary production of the compounds. – show correlations by time periods. The medium correlation (~0.7) of these pollutants with ozone () during this interval confirms that part of the concentration of the pollutants in the environment was due to secondary production. Furthermore, a medium correlation (~0.6) occurred between carbonyls and solar radiation in the 6:00 a.m. to 10:00 a.m. interval (), which then decreased to a low correlation (~0.4) in between 10:00 a.m. and 2:00 p.m. The carbonyl-solar radiation correlation values evidence that, in the first hours of the day as solar radiation increases, the concentration of the three carbonyls increased. At noon, solar radiation remains constant while the concentrations of the carbonyls continue to increase, thus confirming that part of the carbonyls come from secondary production by the photo-oxidation of VOCs. Finally, the low inverse correlation (–0.6 to –0.5) in the last sampling interval () between formaldehyde, acetone, and propionaldehyde with solar radiation confirms the secondary production in the rest of the intervals.
Table 4. Correlationsa for the 6:00 a.m. to 10:00 a.m. sampling interval
Table 5. Correlationsa for the 10:00 a.m. to 2:00 p.m. sampling interval
Table 6. Correlationsa for the 2:00 p.m. to 6:00 p.m. sampling interval
Table 7. Correlationsa for the 6:00 p.m. to 10:00 p.m. sampling interval
Figure 5. Average diurnal variation of (a) solar radiation, (b) temperature, (c) ozone, (d) carbon monoxide, and (e) NOx.
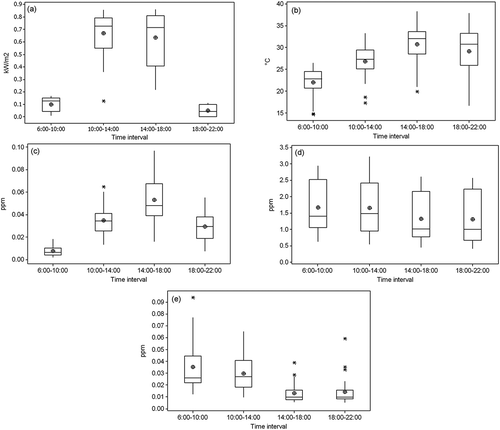
The mid correlation (0.6–0.7) of crotonaldehyde, 2-butanone, and hexaldehyde with solar radiation, and low correlations (<0.5) with propionaldehyde, suggest fractions of these compounds are emitted by biogenic sources during the 6:00 a.m.–10:00 a.m. interval.
Mid-range inverse correlations were found between wind speed and 2-butanone, benzaledhyde, and valeraldehyde (–0.7 to –0.5) in the different sampling intervals. The correlations show that part of the concentration of the pollutants depends on wind-mediated transport.
PM10 had low to medium correlations (0.4-0.6) with formaldehyde, acetaldehyde, and propionaldehyde from 10:00 a.m. to 2:00 p.m., and a correlation of 0.7 with propionaldehyde between 2:00 p.m. and 6:00 p.m. This information is insufficient to reach a conclusion. There were no important correlations between the carbonyls and the rest of the criteria pollutants.
The rest of the carbonyls had no significant variations during the different sampling times and didn’t correlate with O3 or solar radiation. Thus, emission sources are the same throughout the day, and have low or no secondary production.
The high correlations (>0.8) between carbonyls suggest common sources. For propionaldehyde, this indicates that the pollutants are produced in anthropogenic processes in larger measure when the correlation is high and there is more than one emission source when mid correlations (0.5–0.7) were found. Source identification and attribution will be addressed in later work.
Carbonyls–NOx–O3
The control of O3 concentration, a secondary pollutant, represents a challenge due to the complexity of the chemical atmosphere. Ozone’s many precursors and the nonlinearity of the reactions that produce it require an in-depth analysis that provides the government enough information to generate official regulations that address the multiple variables involved in tropospheric O3 concentration. Carbonyls are precursors of this pollutant, as they react with the NOx (NOx = NO + NO2) in the atmosphere in the presence of solar radiation. Therefore, the interrelation of the three pollutants is of interest. shows isopleths of maximum concentration for O3 and average concentration of NOx and carbonyls for the sampling intervals. O3 and NOx averages were calculated from SIMA information in the Obispado station, while total carbonyls represent the sum of carbonyls characterized for each sampling period.
Changes in O3 concentration are observed throughout the day as presented in and . Concentration variability is dependent upon solar radiation intensity () and precursor availability. The highest ozone concentrations ( and ) occurred during the third sampling interval, in the period with the least NOx concentration (), and just after carbonyl compounds reached their maximum concentration between 10:00 and 14:00 hr ( and ).
A sensitivity analysis was performed to assess the effects of carbonyl and NOx variation on O3 concentration. The analysis used the isopleths as a graphic tool. Tests were made by observation. A decrease in carbonyls while having constant NOx concentrations would cause a decrease of O3. However, the inverse process does not have important effects. These overall results are more evident in the mid to high carbonyl concentrations of and . Therefore, O3 is more sensitive to the changes in the concentration of carbonyls. Results found with the graphical analysis made during this research agree with those found by Sierra et al. (Citation2013), who modeled O3 sensitivity in relation to the presence of VOCs in the atmosphere of the MMA. The control of the carbonyls is of interest, especially formaldehyde, which accounts for 50% of the total carbonyls. The control of carbonyl concentrations should include its precursors.
Conclusions
The concentrations of carbonyls analyzed show marked diurnal trends characterized with the highest levels in the period of the most sunlight due to its secondary production. Seasonal variations were observed in the three first samplings. The fourth sampling presented a unique behavior in the concentrations of the compounds due to the rain present throughout the sampling period. The concentrations match those found in other urban areas, although they were higher in this study.
The bivariate statistical analysis showed that the concentrations of carbonyls are caused primarily by emissions from anthropogenic sources (mobile source among them) and secondary production. This shows that the source identification requires more specialized analysis. The heavier aldehydes, > C5, did not correlate with O3 denoting primary emission only.
The interrelation carbonyls–NOx–O3 showed that the atmosphere of Monterrey is sensitive to changes in the concentration of carbonyls. Therefore, it is concluded that a control over their emissions as well as those of their precursors is vital to improve the city’s air quality.
Funding
Acknowledgment is made to the Consejo Nacional de Ciencia y Tecnología (CONACYT) for the grants that made this project possible; to Tecnológico de Monterrey for the support given to this research through CAT-186; and to the Sistema Integral de Monitoreo Ambiental (SIMA) for its support and sharing its sampling facilities with us.
Additional information
Funding
Notes on contributors
H. Lizette Menchaca-Torre
H. Lizette Menchaca-Torre and Alberto Mendoza-Domínguez are professors with the Department of Chemical Engineering at Tecnológico de Monterrey, Campus Monterrey.
Roberto Mercado-Hernández
Roberto Mercado-Hernández is a professor in the Statistics Department of the Biology School at the Universidad Autónoma de Nuevo Leon (UANL).
José Rodríguez-Rodríguez
José Rodríguez-Rodríguez is the Chromatography Supervisor of the Environmental Quality Center at Tecnológico de Monterrey, Campus Monterrey.
Alberto Mendoza-Domínguez
H. Lizette Menchaca-Torre and Alberto Mendoza-Domínguez are professors with the Department of Chemical Engineering at Tecnológico de Monterrey, Campus Monterrey.
References
- Altshuller, A. 1993. Production of aldehydes as primary emissions and from secondary atmospheric reactions of alkenes and alkanes during the night and early morning hours. Atmos. Environ. 27A:21–32. doi:10.1016/0960-1686(93)90067-9
- Atkinson, R. 2000. Atmospheric Chemistry of VOCs and NOx. Atmos. Environ. 34:2063–2101. doi:10.1016/S1352-2310
- Atkinson, R., D.L. Bauluch, R.A. Cox, R.F. Hampson, J.A. Kerr, M.J. Rossi, and J. Troe. 1999. Evaluated kinetic and photochemical data for atmospheric chemistry, Organic species: Supplement VII. J. Phys. Chem. Ref. Data. 28:391–393. doi:10.1063/1.556048
- Báez, A.P., H. Padilla, M.C. Torres, and R. Belmont. 2000. Ambient levels of carbonyls in Mexico City. Atmósfera 13:121–131.
- Báez, A.P., H. Padilla, J. Cervantes, D. Pereyra, M.C. Tores, R. García, and R. Belmont. 2001. Preliminary study of the determination of ambient carbonyls in Xalapa City, Veracruz, Mexico. Atmos. Environ. 35:1813–1819. doi:10.1016/S1352-2310(00)00475-1
- Báez, A.P., M.C. Torres, R.M. García, and H.G. Padilla. 2002. Carbonyls in metropolitan area of Mexico City. Environ. Sci. Pollut. Res. 9:230–233. doi:10.1007/BF02987496
- Carter, W.P. 1994. Development of ozone reactivity scales for volatile organic compounds. J. Air Waste Manage. Assoc. 44:881–899. doi:10.1080/1073161X.1994.10467290
- Cerón, R.M., J.G. Cerón, and M. Muriel. 2007. Diurnal and seasonal trends in carbonyl levels in a semiurban coastal site in the Gulf of Campeche, Mexico. Atmos. Environ. 41:63–71. doi:10.1016/j.atmosenv.2006.08.008
- Cerón-Bretón, J.B., H. Padilla, M.C. Torres, M. Moya, and A.P. Báez. 2005. Measurements of C1–C4 carbonyls at forested regions in Mexico. Atmósfera 18(2): 103–125.
- Cojocariu, C., P. Escher, K.H. Häberle, R, Matyssek, H. Rennenberg, and J. Kreuzwieser. 2005. The effect of ozone on the emission of carbonyls from leaves of adult Fagus sylvatica. Plant Cell Environ. 28:503–611. doi:10.1111/j.1365-3040.2005.01305.x
- Dai, W.T., S.S.H. Ho, K.F. Ho, W.D. Liu, J.J. Cao, and S.C. Lee. 2012. Seasonal and diurnal variations of mono- and di-carbonyls in Xi’an, China. Atmos. Res. 113:102–112. doi:10.1016/j.atmosres.2012.05.001
- Duan, J., S. Guo, J. Tan, S. Wang, and F. Chai. 2012. Characteristics of atmospheric carbonyls during haze days in Beijing, China. Atmos. Res. 114–115:17–27. doi:10.1016/j.atmosres.2012.05.010
- Finlayson-Pitts, B.J., and J.N.J. Pitts. 2000. Chemistry of the Upper and Lower Atmosphere. San Diego, CA: Academic Press.
- Facundo-Torres, D.M., E. Ramírez-Lara, J.G. Cerón-Bretón, R.M. Cerón-Bretón, Y. García-Vasquez, R. Miranda-Guardiola, and J. Rivera de la Rosa. 2012. Measurement of Carbonyls and its relation with Criteria Pollutants (O3, NO, NO2, NOx, CO and SO2) in an Urban site within the metropolitan area of Monterrey, in Nuevo León, México. Int. J. Energy Environ. 6:524–531.
- García-Reynoso, J.A., M. Grutter, and D. Cintora-Juárez. 2007. Evaluación del riesgo por contaminantes criterio y formaldehído en la Ciudad de México. Rev. Int. Contam. Ambie. 23(4): 169–175.
- González-Santiago, O., C.T. Badillo-Castañeda, J.D.W. Kahl, E. Ramírez-Lara, and I. Balderas-Rentería. 2011. Temporal analysis of PM10 in metropolitan Monterrey, Mexico. J. Air Waste Manage Assoc. 61:573–579. doi:10.3155/1047-3289.61.5.573
- Grutter, M., E. Flores, G. Andraca-Ayala, and A. Báez. 2005. Formaldehyde levels in downtown Mexico City during 2003. Atmos. Environ. 39(6): 1027–1034. doi:10.1016/j.atmosenv.2004.10.031
- Grutter, M., E. Flores, R. Basaldud, and L.G. Ruiz-Suárez. 2003. Open-path FTIR spectroscopic studies of the trace gases over Mexico City. Atmos. Oceanic Optics 16:232–236.
- Herrera-Murillo, J., J.F. Rojas-Marín, and S. Rodríguez-Román. 2012. Determination of carbonyls and their sources in three sites of the metropolitan area of Costa Rica, Central America. Environ. Monit. Assess. 184: 53–61. doi:10.1007/s10661-011-1946-5
- Ho, K.F., S.C. Lee, K.K. Louie, and S.C. Zou. 2002. Seasonal variation of carbonyl compound concentrations in urban area of Hong Kong. Atmos. Environ. 35:1235–1252. doi:10.1016/S1352-2310(01)00570-2
- Ho, S.S.H., K.F. Ho, S.C. Lee, Y. Cheng, J.Z. Yu, K.M. Lam, N.S.Y. Feng, and Y. Huang. 2012. Carbonyl emissions from vehicular exhausts sources in Hong Kong. J. Air Waste Manage. Assoc. 62:221–234. doi:10.1080/10473289.2011.642952
- Lu, H., O.Y. Cai, S. Wen, Y. Chi, S. Guo, G. Sheng, and J. Fu. 2010. Seasonal and diurnal variations of carbonyl compounds in the urban atmosphere of Guangzhou, China. Sci. Total Environ. 408:3523–3529. doi:10.1016/j.scitotenv.2010.05.013
- Morknoy, D., P. Khummongkol, and T. Prueaksasit. 2011. Seasonal and diurnal concentrations of ambient formaldehyde and acetaldehyde in Bangkok. Water Air Soil Pollut. 216:696–702. doi:10.1007/s11270-010-0588-5
- Pang, X., and Y. Mu. 2006. Seasonal and diurnal variations of carbonyl compounds in Beijing ambient air. Atmos. Environ. 40:6313–6320. doi:10.1016/j.atmosenv.2006.05.044
- Pinto, J.P., A.S. Lefohn, and D.S. Shadwick. 2004. Spatial variability of PM2.5 in urban areas in the United States. J. Air Waste Manage. Assoc. 54: 440–449. doi:10.1080/10473289.2004.10470919
- Possanzini, M., V. Di Palo, M. Petricca, R. Fratarcangeli, and D. Brocco. 1996. Measurements of lower carbonyls in Rome ambient air. Atmos. Environ. 30:3757–3764. doi:10.1016/1352-2310(96)00110-0
- Possanzini, M., V. Di Paolo, and A. Cecinato. 2002. Sources and photodecomposition of formaldehyde and acetaldehyde in Rome ambient air. Atmos. Environ. 36:3195–3201. doi:10.1016/S1352-2310(02)00192-9
- Possanzini, M., G. Tagliacozzo, and A. Cecinato. 2007. Ambient levels and sources of lower carbonyls at Montelibretti, Rome (Italy). Water Air Soil Pollut. 183:447–454. doi:10.1007/s11270-007-9393-1
- SDU, Secretaría de Desarrollo Urbano, Mexico. 2014. Par Vial Constitución-Morones Prieto. http://www.nl.gob.mx/?P=parvial_2011 (accessed October 20, 2014).
- SEMARNAT. 2012. Capítulo 5. Atmósfera, Compendio de Estadísticas Ambientales. México, D.F.
- Sierra, A., A. Vanoye, and A. Mendoza. 2013. Ozone sensitivity to its precursor emissions in Northeastern Mexico for a summer air pollution episode. J. Air Waste Manage. Assoc. 63:1221–1233. doi:10.1080/10962247.2013.813875
- SIMA. (2014a). Reporte del Estado de la Calidad del Aire en el Área Metropolitana de Monterrey: reportes mensuales enero-diciembre 2013. Monterrey, México.
- SIMA. (2014b). Reporte del Estado de la Calidad del Aire en el Área Metropolitana de Monterrey: reportes mensuales enero-septiembre 2014. Monterrey, México.
- U.S. Environmental Protection Agency. 1999. Determination of formaldehyde in ambient air using adsorbent cartridge followed by high performance liquid chromatography (HPLC). Compendium of Methods of Toxic Organic Compounds in Ambient Air. http://www.awma.org/docs/default-source/JA-WMA-Documents/journal-guidelines-1-20-14.pdf?sfvrsn=0 (accessed May 2010).
- U.S. Environmental Protection Agency. 1998. Objectives of the PM “Supersites” Monitoring Program. Executive summary. http://www.epa.gov/ttnamti1/files/ambient/pm25/suprsite/epaobj2.pdf (accessed March 2010).
- Villanueva, F., A. Notario, J.A. Adame, M.C. Millán, R. Mabilia, and J. Albaladejo. 2013. A preliminary study on ambient levels of carbonyls, benzene, toluene and xylene in the south-west of the Iberian Peninsula (Huelva coast), Spain. Environ. Technol. 34:289–299. doi:10.1080/09593330.2012.692719
- Villanueva-Fierro, I., C.J. Popp, and R.S. Martin. 2004. Biogenic emissions and ambient concentrations of hydrocarbons, carbonyl compounds and organic acids from Ponderosa pine and cottonwood trees at rural forested sites in central New Mexico. Atmos. Environ. 38:249–260. doi:10.1111/j.1600-0889.1989.tb00134.x
- Villasáez, J. 2014. Traban lateral de Gonzalitos. El Norte, July 13. http://www.elnorte.com/aplicaciones/articulo/default.aspx?id=286167
- Viskari, E.L., M. Varrtiainen, and P. Pasanen. 2000. Seasonal and diurnal variation in formaldehyde and acetaldehyde concentrations along a highway in eastern Finland. Atmos. Environ. 34:917–923. doi:10.1016/S1352-2310(99)00307-6
- Wang, B., S.C. Lee, and K.F. Ho. 2007. Characteristics of carbonyls: concentrations and source strengths for indoor and outdoor residential microenvironments in China. Atmos. Environ. 41:2851–2861. doi:10.1016/j.atmosenv.2006.11.039
- World Health Organization. 2000. Guidelines for Air Quality. Geneva, Switerland:WHO.
- Yu, Y., S. Wen, H. Lü, Y. Feng, X. Wang, G. Sheng, and J. Fu. 2008. Characteristics of atmospheric carbonyls and VOCs in Forest Park in South China. Environ. Monit. Assess. 137:275–285. doi:10.1007/s10661-007-9759-2