ABSTRACT
The characteristics and mechanisms of hydrogen sulfide (H2S) adsorption on three different biochars derived from agricultural/forestry wastes through pyrolysis at various temperatures (100 to 500 ºC) were investigated. In this study, the H2S breakthrough capacity was measured using a laboratory-characterized using pH and Fourier transform infrared spectroscopy analysis. The results obtained demonstrate that all biochars were effective in H2S sorption. The sorption capacity of the biochar for H2S removal is related to the pyrolysis temperature and pH of the surface. Certain threshold ranges of the pyrolysis temperature (from 100 to 500 ºC) and pH of the surface are presented. It also concluded that the sorption capacity (for removing H2S) of rice hull-derived biochar is the largest in three biochars (camphor-derived biochar, rice hull-derived biochar, and bamboo-derived biochar). These observations will be helpful in designing biochar as engineered sorbents for the removal of H2S.Implications: This paper focuses on the adsorption of hydrogen sulfide (H2S) by biochars derived from wastes. The characteristics and mechanisms of hydrogen sulfide (H2S) adsorption on three different boichars derived from agricultural/forestry wastes through pyrolysis at various temperatures were investigated. In this study, the H2S breakthrough capacity was measured using laboratory characterization with pH and Fourier-transform infrared spectroscopy analysis. The results obtained demonstrate that all biochars were effective in H2S sorption. The sorption capacity of the biochar for H2S removal is related to the pyrolysis temperature and pH of the surface.
Introduction
Hydrogen sulfide (H2S) is one of the most common compounds that can be found in petrochemical plants, coal gasification plants, wastewater treatment plants, human-made fiber paper, and other production processes (Latos et al., Citation2011; Lebrero et al., Citation2011). It is extremely toxic: It can cause injury to the central nervous system even at low doses, is toxic to microorganisms, and is corrosive to concrete and steel (Burgress et al., Citation2001; Lee et al., Citation2006). It is a major air pollutant when it is emitted to the atmosphere because H2S is not only malodorous but also a source of acidic rains. Therefore, it is imperative to treat the H2S gas stream before it is released into the environment.
Numerous studies on H2S adsorption using activated carbon (AC) have been recently conducted because of the increase in deodorization problems (Bagreev and Bandosz, Citation2000; Bashkova et al., Citation2009). AC can be one of the best options for H2S removal, but the preparation of this adsorbent requires high temperature, high pressure, and an activation process (Boehm, Citation1994). Traditionally, only ACs impregnated with caustics were considered suitable materials. Although caustic-impregnated and catalytic carbons have been proven to work well as H2S removers, certain disadvantages with the application of such carbons have been observed, including (i) low temperature for self-ignition, (ii) low capacity for physical adsorption attributable to the filling of the pore system with the impregnate, (iii) special precaution required for use with alkalis, and (iv) difficulty in regeneration after washing with water. All of the aforementioned factors directed the attention of numerous studies toward unmodified, as-received ACs.
Biochar is a fine-grained and porous substance, similar in appearance to charcoal, that is produced by pyrolysis of biomass under oxygen-limited conditions (Das et al., Citation2008; Lehmann et al., Citation2006; Van-Zwieten et al., Citation2010). In recent years, biochar has received considerable interest as a large-scale soil amendment to improve soil fertility, crop production, and nutrient retention and to serve as a recalcitrant carbon stock (Yang and Sheng, Citation2003; Woolf, Citation2008; Akio et al., Citation2012).
Biochar has a relatively structured carbon matrix with a high degree of porosity and extensive surface area, suggesting that it may act as a surface sorbent that is similar in some aspects to activated carbon and thereby may play an important role in controlling contaminants in the environment. Much work has been done on plant-residue-derived or agricultural wastes-derived biochar for sorbing pollutants (Chen et al., Citation2004; Inyang et al., Citation2012); however, only limited information is available on H2S sorption and the associated underlying mechanisms.
The objective of this paper is to demonstrate the superior performance of materials obtained by simple carbonization of the agricultural/forestry wastes, as adsorbents for removing hydrogen sulfide. These materials are expected to work well as hydrogen sulfide adsorbents, because of their surface texture. The results obtained show that the preparation of these adsorbents is simple, and the efficiency of removing hydrogen sulfide is high.
Experimental Section
Materials
Biochars used in this study were produced from agricultural/forestry wastes (camphor tree, rice hull, and bamboo). The basic properties of raw materials are shown in . The wastes were cut into small pieces, washed, and baked in the oven at 60ºC for 48 hr. The pieces were then ground into small particles using a crusher. The sizes of the particles were smaller than 0.3 mm as obtained after final screening. To prepare the biochars, the agricultural/forestry wastes particles were made to undergo pyrolysis (Gaskin et al., Citation2008; Wannapeera et al., Citation2012) at temperatures between 100 and 500ºC under an oxygen-poor atmosphere in a ceramic fiber muffle furnace. The heating rate was 10ºC/min up to the selected pyrolysis temperature, with a holding time of 5 hr at the final temperature. The samples were cooled by ventilation with nitrogen gas to 30ºC. The samples are referred to as S100P, S200P, S300R, S400B, and S500B (“P” stands for camphor tree samples, “R” stands for rice hull samples, and “B” stands for bamboo samples), where the numbers represent the pyrolysis temperatures of 100, 200, 300, 400, and 500ºC, respectively. shows a schematic diagram of the apparatus.
Table 1. The basic properties of raw materials.
H2S adsorption experiments
The dynamic tests (shown in ) were carried out at room temperature to evaluate the capacity of biochar for H2S removal. Biochar samples were packed into a quartz tube (length 30 mm, diameter 12 mm). The height of biochar bed is 15 cm. The quartz sand is packed above and below the biochar bed. The detailed process of the test is shown in . The source gas containing 0.005% (50 ppm) H2S was then passed through the column of adsorbent at 40 mL/min. The elution of H2S was collected by gas collection bag, then injected to the gas chromatograph (GC) by injection needle. The test was stopped at the breakthrough concentration of 50 ppm. The camphor biochar mass of each test is about 1.0 g. The excess of hydrogen sulfide was absorbed with sodium hydroxide solution each test.
Biochar properties
The concentration of hydrogen sulfide in the exhaust gases from the biochar bed was monitored using Shimadzu gas chromatography (GC; model GC-2010). The separation was conducted on a CNW (a Germany company) CD-1 column (bonded 100% dimethyl siloxane, 30 m long, 0.32 mm internal diameter, and 5.00 µm df). The GC oven heating program was as follows: (1) 80ºC for 2 min, (2) heating from 80ºC to 150ºC at 20ºC/min, and (3) holding at 150ºC for 18 min. The injector temperature was 80ºC. An FPD detector, with a sulfur filter and having a temperature of 220ºC, was used.
The pH of the biochar suspension provides information on the average acidity/basicity of sorbents and was thus measured. A 1.0-g sample of dry biochar was added to 50 mL deionized water. The suspension was then stirred to reach equilibrium using a magnetic stirrer for 30 min, and was then filtered. Finally, the pH of the solution (Cantrell et al., Citation2012; Hina et al., Citation2010) was measured using a pH meter (TWT, 7400).
The Fourier-transform infrared (FTIR) spectra of biochar samples were obtained using diffuse reflectance infrared Fourier-transform spectroscopy. Biohcars were ground to 0.1 mm, and 0.5 mg of each sample was placed onto the Ge window of a Nicolet 5700 FTIR with an ATR attachment (OMNI Sampler Nexus). Spectra were obtained over 256 scans with a KBr beam splitter. It was set at a resolution of 4 cm−1, covering the range of 4500–650 cm−1 and with an aperture size of 34 cm. The reflectance was measured and analyzed using OMNIC v7.1 with Happ–Genzel apodization and Mertz phase correction.
Results and Discussion
Breakthrough curves
Breakthrough curves represent the time profile for saturation of a given amount of an adsorbent. Such adsorbent is structured as a fixed bed with a given solution of an adsorbate, forced through this bed at a constant rate and fixed temperature. Despite being fully empirical and dependent on almost all variables involved, breakthrough curves serve two purposes: (a) to validate if the adsorbent is efficient for the required separation and (b) to establish the breakthrough time (process interruption), based on a criterion that is either technical, economic, or legal (Knaebel, Citation1999). , , and show the breakthrough curves of H2S on different biochars with different pyrolysis temperatures.
Figure 2. Breakthrough curves of H2S on bamboo-derived biochars pyrolyzed at different temperatures.
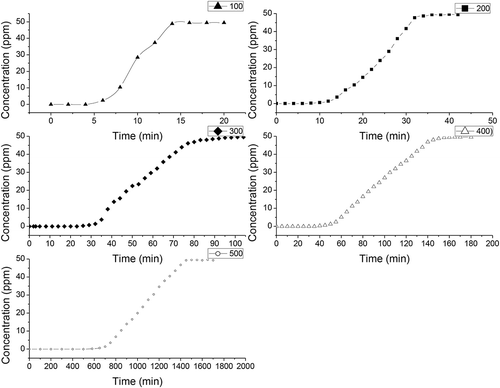
Figure 3. Breakthrough curves of H2S on rice-hull-derived biochars pyrolyzed at different temperatures.
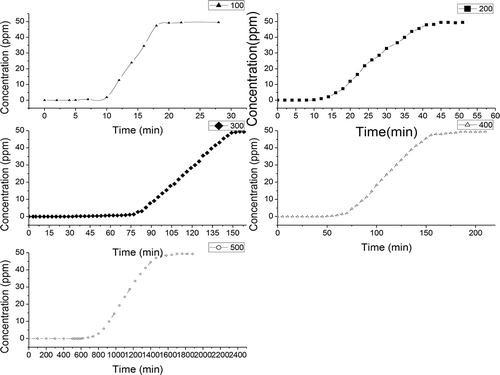
Figure 4. Breakthrough curves of H2S on camphor-derived biochars pyrolyzed at different temperatures.
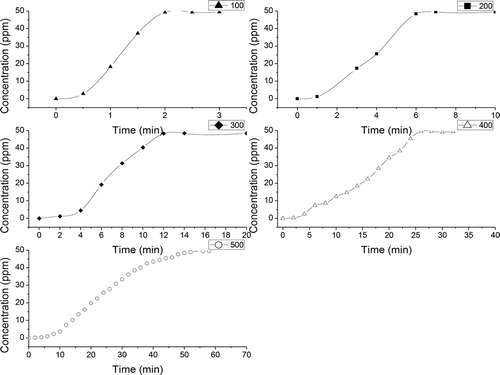
Various factors affect the breakthrough curves, such as the nature of the adsorbate and adsorbent, the column geometry, and operating conditions. Favorable adsorption isotherms and extremely high adsorption rates would result in a virtually coinciding breakthrough time and exhaustion point. (Breakthrough time was defined as the time when the outlet concentration was just greater than 0.05 ppm. The saturation time was defined as the time when the outlet concentration was just greater than 48.5 ppm.) Thus, the breakthrough curves become sharp. The breakthrough time is the point on the breakthrough curve where the effluent adsorbate reaches its maximum allowable concentration, which often corresponds to the treatment goal. , , and show that the breakthrough time is dependent on pyrolysis temperature and different raw materials. The breakthrough time for different biochars increased with increasing pyrolysis temperature. When the pyrolysis temperature increased from 100ºC to 500ºC, the breakthrough time for different biochars gradually increased. For camphor-derived biochars, the breakthrough time increased from 50 min to 360 min; for rice hull-derived biochars, the breakthrough time increased from 5 min to 620 min; and for bamboo-derived biochars, the breakthrough time increased from 5 min to 580 min (). Hossain et al. (Citation2011) and Peng et al. (Citation2011) reported that pyrolysis temperature has a significant effect on the chemical, physical, morphological, and spectral properties of biochars. The scanning electron microscope image presents evidence that the surface area of biochar can be increased with pyrolysis temperature (Brodowski et al., Citation2005; Keiluweit et al., Citation2011). The increase in surface area provides a greater number of adsorption sites and space for H2S.
Table 2. The physicochemical characteristics of the biochars, and H2S breakthrough time, saturation time, and H2S breakthrough capacity for the different biochars.
The breakthrough time was also dependent on the raw materials of biochar. For camphor-derived biochars, the minimum breakthrough time was 50 min, and the maximum breakthrough time was 360 min; for rice-hull-derived biochars, the minimum breakthrough time was 5 min, and the maximum breakthrough time was 620 min; for bamboo-derived biochars, the minimum breakthrough time was 5 min, and the maximum breakthrough time was 580 min. The raw materials for biochars differ in terms of elemental composition; the presence of soil and dust particles; moisture content; and lignin, cellulose, and hemicelluloses content, which, in turn, affect the properties of the respective biochars after pyrolysis (Ubbelhde and Lewis, Citation1960; Boehm, Citation1994; Alexis et al., Citation2007; Yip et al., Citation2007), and finally affect the adsorption of the H2S (shown in ).
pH of biochar
The degree of acid dissociation is known to be dependent on the pH of the system. Dissociation is feasible when the pH value is greater than the pKa of the acid under study. H2S is a weak diprotic acid with first and second dissociation constants equal to 7.2 and 13.9, respectively. To further study the mechanism of adsorption of H2S on the different biochars, the pH values of the surface of the biochar samples were determined. The results are shown in . The pH of the surface of the camphor-derived biochar samples ranged from 5.45 to 9.55, for the rice hull-derived biochar samples it ranged from 6.45 to 10.56, and for the bamboo-derived biochar samples it ranged from 6.25 to 10.21. With equal pyrolysis temperature, the pH of the surface of the samples was different; the rice hull-derived biochar’s was the highest, and the camphor-derived biochar’s was lowest among the three biochars. The pH of surface of the samples increased with increasing pyrolysis temperature (). The source of alkalinity might be divided into organic and inorganic materials, and the organic ones might have been decomposed by higher temperature. The pH changes of the samples might be due to the composition (organic and inorganic materials) of the samples decomposed under different pyrolysis temperatures. Some composition, such as hemicelluloses and cellulose (Cantrell et al., Citation2012) decomposed below 400ºC. Thus, the surfaces of these samples are acidic. According to Bagreev et al. (Citation2001), acidity, expressed as pH, should have a value greater than 5. Such a value ensures the effectiveness of H2S removal from the gas phase.
Table 3. The surface pH of the different biochars.
H2S is an acidic gas. When H2S comes in contact with the sample’s alkaline surface, it is adsorbed easily. The surface pH of the S500R sample is 10.56, which is the highest among those of all samples. Moreover, the S500R sample has the strongest capacity for H2S adsorption. This inference is consistent with the result of the H2S adsorption curves.
Adsorption capacity
Breakthrough capacity is defined as the mass of the absorbate removed by the adsorbent at breakthrough concentration or the maximum acceptable (desired) concentration. When the effluent concentration reaches this value, the adsorbent requires replacement. For each adsorption experiment, the reactor was operated until the H2S outlet concentration matched the inlet concentration (exhaustion). Breakthrough curves were thus obtained by plotting the H2S concentration in the outlet gas vs. time. The adsorption capacity (x/M, mg H2S g−1 of adsorbent) for each adsorbent system was calculated as follows (Anfrums et al., Citation2011; Ros et al., Citation2006):
where x is the mass of the adsorbed H2S (mg), M is the mass of the adsorbent (g), Q is the inlet flow(m3 s−1), ω is the adsorbent weight (g), Mw is the H2S molecular weight (mg mmol−1), VM is the ideal gas molar volume(mL mmol−1), ce is the H2S inlet concentration (ppm, v/v), ts is the bed exhaustion time (s) and c(t) is the H2S outlet concentration (ppm, v/v) at a given time. also summarizes the capacity measured for a breakthrough ranges from 1.78 mg/g to 382.7 mg/g. Among all samples, S500R has the strongest capacity of H2S breakthrough. The S500R is remarkably superior to all other samples. Bagreev et al. (Citation2001a) reported that the capacity of removal H2S was 104.5 mg/g by sludge biochar. Xiao et al. (Citation2014) reported that the pH of the surface and the carbon content were important to removal H2S. They used sludge biochar and pig manure biochar to adsorb the H2S, for which the adsorption capacity was 43.9 mg/g and 59.6 mg/g, respectively. The samples (S500P, S500B, S500R) we tested included more carbon contents adsorption capacity so were superior to theirs. The change in the saturation time of samples is similar to the change in the breakthrough time.
The adsorption capacity was also dependent on surface area of biochars and the carbon content. For all biochars, the adsorption capacity increased with the increase of the surface area and carbon content (see ). The results were consistent with the results of Xiao et al., (Citation2014) and Bagreev et al. (Citation2001a).
On the basis of the surface chemistry and the performances of carbons, Adib et al. (Citation1999a, Citation1999b) suggested that the H2S breakthrough capacity is governed by local pH within the pore system. Acidic pH suppresses dissociation of H2S; consequently, its oxidation to sulfur is limited. The proposed mechanism involves (eq 2) H2S adsorption on the C surface, (eq 3) H2S dissolution in a water film, (eq 4) dissociation of H2S in an adsorbed state in the water film, (eq 5a) surface reaction of adsorbed O2 with the formation of elemental sulfur (eq 5b) or sulfur dioxide, and (eq 6) further oxidation of SO2 to H2SO4 in the presence of water (Adib et al., Citation1999a, Citation1999b, Citation2000):
where H2Sgas, H2Sads-liq, and H2Sads correspond to the H2S in the gas, liquid, and adsorbed phases, respectively; KH, KS, Ka, and KR1, KR2, and KR3 denote the equilibrium constants for related processes (adsorption, gas solubility, dissociation, and surface reaction constants); is dissociatively adsorbed O2; and Sads, SO2ads, and H2SO4ads represent sulfur, SO2, and H2SO4 as the end products of the surface oxidation reactions, respectively. The mechanism of H2S removal by biochars probably differs from that of the activated carbons. As proposed by Hedding and Rao (Citation1976), at the virgin carbon surface, dissociation of hydrogen sulfide occurs in the film of adsorbed water and then hydrogen sulfide ions or HS- is oxidized by oxygen radicals to elemental sulfur. On the other hand, when caustic is present, it catalyzes oxidation to elemental sulfur until all base is exhausted (Turk et al., Citation1992).
Infrared spectra
The FTIR spectra of all biochars are shown in , with band assignments and trends on pyrolysis indicated in . For camphor-derived biochars, a broad peak at 3420 cm−1 is associated with the hydroxide (OH) stretching vibration. However, as the temperature of the preparation of biochar rises, the intensity of the peak becomes weaker. Thus, the content of the OH is reduced. Thus, as the pyrolysis temperature rises, the OH groups are dehydrated. This phenomenon is similar to that observed in Huang’s research (Huang et al., Citation2011), where a peak at 2920 cm−1 corresponds to the CH2 stretching vibration. However, in the S300P, S400P, and S500P samples, the CH2 stretching vibration was not evident. This phenomenon may be attributed to the thermal decomposition process. A peak at 1730 cm−1 corresponds to the C=O stretching vibration. The new peak is mentioned later. For curves d and e, the sharp peak appearing at the wave number of 1433 cm−1 can be used to confirm the COO stretching vibration. The same FTIR spectroscopy also occurs in the other curves d and e. There is a similar phenomenon for the FTIR spectra of the bamboo-derived biochars and rice-hull-derived biochars at wave numbers from 3500 cm−1 to 1400 cm−1, but for rice hull-derived biochars of S400R and S500R, at 2800 cm−1 a weak peak appeared, and at 800 cm−1 a new peak also appeared. For bamboo-derived biochars of S400B and S500B, at 800 cm−1 a new peak appeared too (shown in ).
Table 4. The FTIR wave number of samples for the different biochar samples.
Figure 5. (a) FTIR spectra of the camphor-derived biochar samples; (b) FTIR spectra of the rice-hull-derived biochar samples; and (c) FTIR spectra of the bamboo-derived biochar samples.
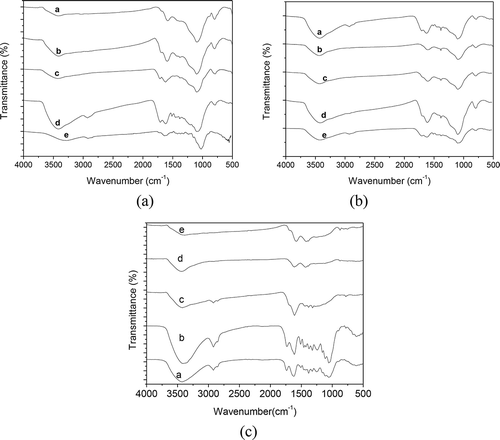
With increasing pyrolysis temperature, the bands assigned to the O–H stretching vibration and the aliphatic C–H stretching vibration decreased markedly and almost disappeared. This indicates that labile aliphatic compounds decreased continuously in biochars with increasing pyrolysis temperature and that demethoxylation, demethylation, and dehydration of lignin occurred (Kleen and Gellerstedt, Citation1995; Jakab et al., Citation1997; Shama et al., Citation2004). Bagreev et al. (Citation2001) and Rutherford et al. (Citation2004) found that pyrolysis temperatures above 400°C enhance dehydroxylation; the loss of OH and aliphatic groups gives rise to pore formation due to a concurrent development of fused-ring structures. The decomposition of hemicellulose during pyrolysis can be followed by the disappearance of the bands at 1740 to 1730 cm−1, which indicates the removal of acetyl ester groups (Schwanninger et al., Citation2004; Stefke et al., Citation2008). Hence, the result of the FTIR spectra of all biochar has an effect on the H2S breakthrough times.
Conclusion
This study indicated that biochars produced from agricultural/forestry wastes (camphor, rice hull, and bamboo) can effectively adsorb H2S from odor gas. The sorption capacity of the biochar for H2S removal is related to the pyrolysis temperature and pH of the surface. Certain threshold ranges of the pyrolysis temperature (from 100 to 500ºC) and pH of the surface are present. It also concluded that the sorption capacity (for removing H2S) of rice-hull-derived biochar is the largest of the three biochars (camphor-derived biochar, rice hull-derived biochar, and bamboo-derived biochar). These observations will be helpful in designing biochars as engineered sorbents for the removal of H2S.
Funding
This study was supported by the Postdoctoral Foundation of China (14Z102060010) and the Innovation of Science and Technology Special-Postdoctoral Research Fund (14X100030005).
Additional information
Funding
Notes on contributors
Guofeng Shang
Guofeng Shang is a postdoctor at the Department of Environment and Resource, School of Agriculture and Biology, Shanghai Jiao Tong University, Shanghai, China.
Qiwu Li
Qiwu Li is an engineer and the Hunan Environmental Monitoring Center, Changsha, Hunan Changsha, Hunan, China.
Liang Liu
Liang Liu is a doctor at the Department of Environment and Resource, School of Agriculture and Biology, Shanghai Jiao Tong University, Shanghai, China.
Ping Chen
Ping Chen is a doctor at the Department of Environment and Resource, School of Agriculture and Biology, Shanghai Jiao Tong University, Shanghai, China.
Xiamei Huang
Xiamei Huang is an engineer and the District of Hangzhou Environmental Protection Bureau, Hangzhou, Zhejiang, P.R. China
References
- Azargohar, R., and A.K. Dalai. 2011. The direct oxidation of hydrogen sulphide over activated carbons prepared from lignige coal and biochar. Can. J. Chem. Eng. 2011:1–10.
- Akio, E., H. Kelly, and W. Thea. 2012. Characterization of biochars to evaluate recalcitrance and agronomic performance. Bioresource Technol. 114:644–53.
- Alexis, M. A., D.P. Rasse, C. Rumpel, G. Bardoux, and N. Pechot. 2007. Fire impact on C and N losses and charcoal production in a scrub oak ecosystem. Biogeochemistry 82:201–16. doi:10.1007/s10533-006-9063-1
- Adib, F., V. Bagreev, and T.J. Bandosz. 1999a. Effect of surface characteristics of wood-based activated carbons on adsorption of hydrogen sulfide. J. Colloid Interface Sci. 214(2):407–15. doi:10.1006/jcis.1999.6200
- Adib, F., A. Bagreev, and T.J. Bandosz. 1999b. Effect of pH and surface chemistry on the mechanism of H2S removal by actibated carbons. J. Colloid Interface Sci. 216(2):360–69.
- Adib, F., A. Bagreev, and T.J. Bandosz. 2000. Analysis of the relationship between H2S removal capacity and surface properties of unimpregnated activated carbons. Environ. Sci. Technol. 34(4):686–92. doi:10.1021/es990341g
- Anfruns, A., M. Martin, and M. Montes-Moran. 2011. Removal of odourous VOCs using sludge-based adsorbents. Chem. Eng. J. 166:1022–31. doi:10.1016/j.cej.2010.11.095
- Bandosz, T.J. 2001. On the adsorption/oxidation of hydrogen sulfide on activated carbons at ambient temperatures. J. Colloid Interface Sci. 246:1–20. doi:10.1006/jcis.2001.7952
- Bashkova, S., T.R. Armstrong, and V. Schwartz. 2009. Selective catalytic oxidation of hydrogen sulfide on activated carbons impregnated with sodium hydroxide. Energy Fuels 23:1674–82. doi:10.1021/ef800711c
- Bagreev, A., T. Bandosz, and D. Locke. 2001. Pore structure and surface chemistry of adsorbents obtained by pyrolysis of sewage-derived fertilizer. Carbon 39:1971–79. doi:10.1016/S0008-6223(01)00026-4
- Burgess, J.E., S.A. Parsons, and R.M. Stuetz. 2001. Developments in odour control and waste gas treatment biotechnology: a review. Biotechnol. Adv. 19:35–63. doi:10.1016/S0734-9750(00)00058-6
- Brodowski, S., W. Amelung, L. Haumaier, C. Abetz, and W. Zech. 2005. Morphological and chemical properties of black carbon in physical soil fractions as revealed by scanning electron microscopy and energy-dispersive x-ray spectroscopy. Geoderma 128(1–2):116–29. doi:10.1016/j.geoderma.2004.12.019
- Bagreev, A., F. Adib, and T.J. Bandosz. 2001a. pH of activated carbon surface as an indication of its suitability for H2S removal from moist air streams. Carbon 39(12):1897–905. doi:10.1016/S0008-6223(00)00317-1
- Bagreev, A., and T.J. Bandosz. 2000. Study of hydrogen sulfide adsorption on activated carbons using inverse gas chromatography at infinite dilution. J. Chem. Phys. B 104(37):8841–47. doi:10.1021/jp0011037
- Boehm, H.P. 1994. Some aspects of the surface chemistry of carbon blacks and other carbons. Carbon 32:759–69. doi:10.1016/0008-6223(94)90031-0
- Chen, Y., G. Shen, C.T. Chiou, and B. Xing. 2004. Compositions and sorptive properties of crop residue-derived chars. Environ. Sci. Technol. 38(17):4649–55. doi:10.1021/es035034w
- Cantrell, K.B., P.G. Hunt, M. Uchimiya, and J.M. Novak. 2012. Impact of pyrolysis temperature and manure source on physicochemical characteristics of biochar. Bioresource Technol. 107:419–28. doi:10.1016/j.biortech.2011.11.084
- Das, K.C., M. Garcia-Perez, B. Bibens, and N. Melear. 2008. Slow pyrolysis of poultry litter and pine woody biomass: Impact of chars and bio-oils on microbial growth. J. Environ. Sci. Health, Part A 43(7):714–24. doi:10.1080/10934520801959864
- Gaskin, J.W., C. Steiner, K. Harris, K.C. Das, and B. Bibens. 2008. Effect of low-temperature pyrolysis conditions on biochar for agricultural use. Trans. ASABE 51(6):2061–69. doi:10.13031/2013.25409
- Hina, K., P. Bishop, M.C. Arbestain, J.A. Macia-Agullo, and J. Hindmarsh. 2010. Producing biochars with enhanced surface activity through alkaline pretreatment of feed stocks. Austr. J. Soil Res. 48(7):606–17. doi:10.1071/SR10015
- Hossain, M.K., V. Strezov, K.Y. Chan, A. Ziolkowski, and P.F. Nelson. 2011. Influence of pyrolysis temperature on production and nutrient properties of wastewater sludge biochar. J. Environ. Manage. 92(1):223–28. doi:10.1016/j.jenvman.2010.09.008
- Huang, Y., Z. Wei, Z. Qiu, X. Yin, and C. Wu. 2011. Study on structure pyrolysis behavior of lignin derived from corncob acid hydrolysis residue. J. Anal. Appl. Pyrol. 93:153–59. doi:10.1016/j.jaap.2011.10.011
- Hedding, K., and B.R. Rao. 1976. VDI-Ber. No. 235, S. 37/42.
- Inyang, M., B. Gao, Y. Yao, Y.W. Xue, and A. Zimmerman. 2012. Removal of heavy metals from aqueous solution by biochars derived from anaerobically digested biomass. Bioresource Technol. 110:50–56. doi:10.1016/j.biortech.2012.01.072
- Jakab, E., O. Faix, and F. Till. 1997. Thermal decomposition of milled wood lignins studied by thermogravimetry/mass spectrometry. J. Anal. Appl. Pyrol. 40–41:171–86. doi:10.1016/S0165-2370(97)00046-6
- Knaebel, K. 1999. The basics of adsorber design. Chem. Eng. 106(4):92–101.
- Kleen, M., and G. Gellerstedt. 1995. Influence of inorganic species on the formation of polysaccharide and lignin degradation products in the analytical pyrolysis of pulp. J. Anal. Appl. Pyrol. 35:15–41. doi:10.1016/0165-2370(95)00893-J
- Keiluweit, M., P.S. Nico, M.G. Johnson, and M. Kleber. 2011. Dynamic molecular structure of plant biomass-derived black carbon (biochar). Environ. Sci. Technol. 44(4):1247–53. doi:10.1021/es9031419
- Latos, M., P. Karageorgos, N. Kalogeraki, and M. Lazaridis. 2011. Dispersion of odorous gaseous compounds emitted from wastewater treatment plants. Water Air Soil Pollut. 215(4):667–77. doi:10.1007/s11270-010-0508-8
- Lebrero, R., L. Bouchy, R. Stuetz, and R. Murioz. 2011. Odor assessment and management in wastewater treatment plants: A review. Crit. Rev. Environ. Sci. Technol. 41(10):915–50. doi:10.1080/10643380903300000
- Lee, E.Y., N.Y. Lee, K.S. Cho, and H.W. Ryu. 2006. Removal of hydrogen sulfide by sulfate-resistant acidithiobacillus thiooxidans AZ11. J. Biosci. Bioeng. 101(4):309–14. doi:10.1263/jbb.101.309
- Lehmann, J., J. Gaunt, and M. Rondon. 2006. Bio-char sequestration in terrestrial ecosystems—A review. Mitigation Adaptat. Strat. Global Change 11:403–27. doi:10.1007/s11027-005-9006-5
- Peng, X., L. Ye, C. Wang, H. Zhou, and B. Sun. 2011. Temperature- and duration-dependent rice straw-derived biochar: Characteristics and its effects on soil properties of an ultisol in southern China. Soil Tillage Res. 112(2):159–66. doi:10.1016/j.still.2011.01.002
- Ros, A., M. Montes-Moran, E. Fuente, D.M. Neskaia, and M.J. Martin. 2006. Dried sludges and sludge-based chars for H2S removal at low temperature: influence of sewage sludge characteristics. Environ. Sci. Technol. 40:30–39. doi:10.1021/es050996j
- Rutherford, D.W., R. Wershaw, and L. Cox. 2004. Changes in composition and porosity occurring during the thermal degradation of wood and wood components. Sci. Invest. Rep. 8:5285–92.
- Sharma, R., J. Wooten, V. Baliga, X. Lin, and W. Chan. 2004. Characterization of chars from pyrolysis of lignin. Fuel 83:1469–82. doi:10.1016/j.fuel.2003.11.015
- Schwanninger, M., J.C. Rodrigues, H. Pereira, and B. Hinterstoisser. 2004. Effects of short-time vibratory milling on the shape of FT-IR spectra of wood and cellulose. Vibrational Spectrosc. 36:23–40. doi:10.1016/j.vibspec.2004.02.003
- Stefke, B., E. Windeisen, M. Schwanninger, and B. Hinterstoisser. 2008. Determination of the weight percentage gain and of the acetyl group content of acetylated wood by means of different infrared spectroscopic methods. Anal. Chem. 80:1272–79. doi:10.1021/ac7020823
- Turk, A., E. Sakalis, O. Rago, and H. Karamitsos. 1992. Activated carbon systems for removal of light gases. Ann. NY Acad. Sci. 661:221–28. doi:10.1111/j.1749-6632.1992.tb26043.x
- Ubbelohde, A.R., and F.A. Lewis. 1960. Graphite and Its Crystal Compounds. Oxford, UK: Clarendon Press.
- Van-Zwieten, L., S. Kimber, S. Morris, K.Y. Chan, and A. Downie. 2010. Effects of biochar from slow pyrolysis of papermill waste on agronomic performance and soil fertility. Plant Soil 327(1–2):235–46. doi:10.1007/s11104-009-0050-x
- Woolf, D. 2008. Biochar as a soil amendment: A review of the environmental implications. Swansea: Swansea University, 2008.
- Wannapeera, J., B. Fungtammasan, and N. Worasuwannarak. 2012. Effects of temperature and holding time during torrefaction on the pyrolysis behaviors of woody biomass. J. Anal. Appl. Pyrol. 92(1):99–105. doi:10.1016/j.jaap.2011.04.010
- Xu, X.Y., X.D. Cao, L. Zhao, and T.H. Sun. 2014. Comparison of sewage sludge-and pig manure-derived biochars for hydrogen sulfide removal. Chemosphere 111:296–303. doi:10.1016/j.chemosphere.2014.04.014
- Yip, K., H. Wu, and D.K. Zhang. 2007. Effect of inherent moisture in collie coal during pyrolysis due to in-situ steam gasification. Energy Fuels 21:2883–91. doi:10.1021/ef7002443
- Yang, Y.N., and G.Y. Sheng. 2003. Enhanced pesticide sorption by soils containing particulate matter from crop residue burns. Environ. Sci. Technol. 37:3635–39. doi:10.1021/es034006a