ABSTRACT
Landfill gas (LFG) generation is predicted by a first-order decay (FOD) equation that incorporates two parameters: a methane generation potential (L0) and a methane generation rate (k). Because non-hazardous waste landfills may accept many types of waste streams, multiphase models have been developed in an attempt to more accurately predict methane generation from heterogeneous waste streams. The ability of a single-phase FOD model to predict methane generation using weighted-average methane generation parameters and tonnages translated from multiphase models was assessed in two exercises. In the first exercise, waste composition from four Danish landfills represented by low-biodegradable waste streams was modeled in the Afvalzorg Multiphase Model and methane generation was compared to the single-phase Intergovernmental Panel on Climate Change (IPCC) Waste Model and LandGEM. In the second exercise, waste composition represented by IPCC waste components was modeled in the multiphase IPCC and compared to single-phase LandGEM and Australia’s Solid Waste Calculator (SWC). In both cases, weight-averaging of methane generation parameters from waste composition data in single-phase models was effective in predicting cumulative methane generation from -7% to +6% of the multiphase models. The results underscore the understanding that multiphase models will not necessarily improve LFG generation prediction because the uncertainty of the method rests largely within the input parameters. A unique method of calculating the methane generation rate constant by mass of anaerobically degradable carbon was presented (kc) and compared to existing methods, providing a better fit in 3 of 8 scenarios. Generally, single phase models with weighted-average inputs can accurately predict methane generation from multiple waste streams with varied characteristics; weighted averages should therefore be used instead of regional default values when comparing models.
Implications: Translating multiphase first-order decay model input parameters by weighted average shows that single-phase models can predict cumulative methane generation within the level of uncertainty of many of the input parameters as defined by the Intergovernmental Panel on Climate Change (IPCC), which indicates that decreasing the uncertainty of the input parameters will make the model more accurate rather than adding multiple phases or input parameters.
Introduction
To predict landfill gas (LFG) generation, a first-order decay (FOD) equation is employed. LFG is primarily composed of methane (CH4) and carbon dioxide (CO2), although many trace gases are present. Because methane is both a potent greenhouse gas (GHG) and a potential renewable fuel source, the FOD equation considers only methane-generating parameters: (1) the methane generation potential (L0) or the degradable organic carbon (DOC) content and (2) the methane generation rate (k). Many institutions and government agencies have developed LFG models for gas collection and control system (GCCS) design, regulatory purposes, or GHG accounting, and all models use the same fundamental FOD equation.
Methane generation parameters
L0 (m3 CH4/Mg waste) specifies the total amount of methane, the product of methanogenesis, which may be released from a material in an anaerobic landfill environment. The parameter is assigned on a wet-weight basis to allow for operators to directly input as-received landfill tonnage into FOD models. L0 values for waste streams and individual waste components (L0i) have been quantified stoichiometrically and experimentally (Cho et al., Citation2012). Component-specific L0i values have been used in combination with waste composition data to estimate L0 of an entire waste stream or landfill (Eleazer et al., Citation1997). A weighted-average L0 would represent an equivalent amount of potential methane per mass of waste as that resulting from the sum of all individual components. When accurate waste composition data are available, this process can be applied to determine L0 values for each year of disposal (Machado et al., Citation2009; Cho et al., Citation2012). This type of analysis may provide guidance when considering the impact of landfill diversion scenarios on future LFG generation (Pan and Voulvoulis, Citation2007; Thompson et al., Citation2009).
DOC (Mg carbon/Mg waste) enumerates the concentration of the biodegradable carbon (C) per mass of material. Like L0, DOC is a wet-weight parameter and it identifies the material that will generate LFG, namely, biodegradable C. However, not all DOC is anaerobically degradable (e.g., lignin and humic acids) and not all anaerobically degradable C is bioavailable (Stinson and Ham, Citation1995). Thus, the DOC within a waste stream or DOCi within waste component i do not alone indicate how much methane would be generated within a landfill. The Intergovernmental Panel on Climate Change (IPCC) has identified several other parameters that transform DOC to L0 (IPCC, Citation2006). The IPCC has identified a large degree of uncertainty within each of their default parameter values, recommending the use of site-specific information when available (IPCC, Citation2000). For example, the uncertainty range for IPCC’s default DOC is −50% to +20%, which directly influences the amount of predicted methane generation. The uncertainty within the DOC would then be integrated into the respective L0, such that a model using DOC and a model using the translated L0 would incorporate the same level of uncertainty. Many international FOD models use DOC parameters to characterize their waste streams instead of L0. The inputs to each FOD model can be translated to an appropriate, equivalent input to all other models, although this point is not often emphasized (Scharff and Jacobs, Citation2006; Mou et al., Citation2015). Successful translation of a parameter value to another model (e.g., DOC to L0) would yield similar FOD curves.
k (yr−1) describes the rate at which methane generation (or C decay) will occur. Whereas L0 and DOC are solely a function of the waste, k is also influenced by environmental conditions such as climate and engineered conditions such as liquids addition (Kim and Townsend, Citation2012; Sormunen et al., Citation2013; Tolaymat et al., Citation2010). As with L0, experimentally determined k values for individual waste components have been applied to field-scale waste composition data to determine a single, bulk k value (De la Cruz and Barlaz, Citation2010). A more common approach to identify field-scale k has been to determine L0 by waste composition as previously described and select k by best fit to measured LFG values (Amini et al., Citation2012). The impact of waste composition on k, particularly the bioavailability of C for landfill methane generation, is not well understood. Because LFG generation is modeled with an exponential function, the selection of k becomes important for predicting whether methane will be generated quickly or over a long period of time.
Use of FOD models
The FOD equation has historically been used to predict methane generation from a single, bulk municipal solid waste (MSW) stream with homogenous characteristics. LandGEM, developed by the U.S. Environmental Protection Agency (EPA), is widely utilized because it requires inputs for only a single waste stream, and thus is commonly referred to as a “single-phase” model. Although LandGEM is popular because of its simplicity, more accurate predictions may be possible for landfills with known waste composition and “multiphase” models have been developed for this purpose (Scharff and Jacobs, Citation2006). These models allow operators and engineers to predict LFG generation from multiple waste components with unique L0 and k values (Mou et al., Citation2015).
It is important to note that in the context of this research and in previous texts “phase” is synonymous to “waste stream” or “waste component” (Govindan and Agumuthu, Citation2014). Multiphase models, therefore, incorporate multiple waste streams by performing the calculations for each phase in parallel and summing the results to predict total annual generation. This terminology should not be confused with other modeling approaches that may, for example, predict LFG transport as a function of multiphase phenomena (i.e., gaseous and liquid interactions), which are not used in FOD modeling and are not further discussed.
Single-phase and multiphase FOD models
LandGEM, Afvalzorg, and IPCC Waste Model contain regioal default methane generation values for L0, DOC, k, and other parameters. These default values reflect economic, regulatory, and geographic characteristics, such as climate, of the locations in which the models were developed. However, the parameter inputs can be amended to any user-defined value. It has been suggested that single-phase models such as LandGEM may be unable to effectively predict methane generation from landfills with unique or heterogeneous wastes (Scharff and Jacobs, Citation2006; Mou et al., Citation2015). In such cases, multiphase models, such as Afvalzorg or the IPCC Waste Model, have been considered more capable.
The objective of this research was to compare the predictions of methane generation from multiple waste streams input into a multiphase model with a single, weighted-average waste stream translated for input into a single-phase model. Does a translated single-phase model predict similarly to a multiphase model? A successful translation of multiple waste components with respective DOC values to a single DOC value attributed to a bulk waste would yield the same amount of biodegradable C (i.e., multiphase to single-phase). Similarly, multiple rates of decay would be translated to a single rate of decay that would predict a similar trend of methane generation over the life of the landfill.
Oonk and Boom (Citation1995) noted that multiphase models may be able to fit to measured results better than a single-phase model because they utilize more parameters, but also indicated that the greatest source of error was within the selection of parameter values. If the uncertainty of the methane generation parameters and waste composition data is greater than the difference between modeled results of multi- and single-phase models, a single-phase model could be said to accurately predict methane generation from a landfill receiving heterogeneous waste streams. Therefore, the comparison between multiphase and weighted-average single-phase results would be valuable to more clearly identify the strengths and weaknesses of each approach. In reality, accuracy of a FOD model is quantified in relation to measured LFG collection and/or emissions because LFG generation cannot be measured directly. In this study, only waste composition data were used and accuracy describes the closeness-of-fit of single-phase models to multiphase models with the assumption that the multiphase models accurately predict LFG generation.
Models and methods
Five LFG generation models were used to predict methane generation from four MSW landfills located in Denmark in two exercises. Waste composition and annual landfilled tonnages were defined by both Afvalzorg waste streams and IPCC waste components reported in the supporting information (SI) of Mou et al. (Citation2015) for four landfills: AV Miljø, Odense, Audebo, and Glatved. These landfills were selected for this research because they had extensive waste composition and tonnage data for use in multiple models.
Model descriptions are given in the following sections. Using waste composition data, annual waste tonnages, and multiphase methane generation parameters, appropriate weighted-average single-phase inputs were translated. Calculation and translation of each of the methane generation input parameters is described in the section titled “Translating parameters.” The section “Exercise methodology” describes the process through which the application of the waste composition data reported by Mou et al. (Citation2015) were used to compare and contrast the FOD models. All weights were reported in metric tonnes, parameterized in Megagrams (1 Mg = 1000 kg = 1 t). To compare the modeled results, annual generation values were converted to m3 CH4/yr and compared cumulatively over 100 yr. Annual methane generation was compared by closeness-of-fit as described in Statistical Analyses subsection.
Previous research has validated FOD models for use in LFG modeling (Scharff and Jacobs, Citation2006; Mou et al., Citation2015). Validation typically would require gas collection data and/or emission data. Only methane generation was modeled in this research. Landfill emissions, which may require an oxidation factor, were not calculated and were not in the scope of this research. Instead, this study compares predicted methane generation based on similar input values translated from multiphase to single-phase models.
LandGEM
LandGEM was developed to allow engineers to predict LFG and methane generation from MSW landfills in the United States according to the EPA’s New Source Performance Standards (EPA, Citation1996). The model requires two methane generation parameter inputs: L0 and k. Although most MSW landfills in the United States may accept construction and demolition debris (C&DD) and other nonhazardous wastes subject to state and federal regulations (EPA, Citation1991), all landfilled waste is considered a single phase within the model. The equation employed in LandGEM version 3.02 is shown below:
where QCH4 is the annual methane generation (m3 CH4/yr); n is the number of years since waste was first accepted; k is the methane generation rate (yr−1); L0 is methane generation potential (m3 CH4/Mg waste); Mi is the mass of waste landfilled in the ith year (Mg); and tij is the age of the jth section of Mi accepted in the ith year (decimal years, e.g., 3.2 yr).
Two sets of default values exist within LandGEM: the Clean Air Act (CAA) values and the AP-42: values. The CAA defaults are required for use by engineers to predict if MSW landfills will require gas collection systems. The CAA values are conservative for regulatory purposes and are not suggested to predict actual generation within the landfill (EPA, Citation1998). The AP-42 default values are used for GHG emission inventories and to size a GCCS based on expected peak flow of LFG. Additionally, LandGEM allows for user-defined inputs for L0 and k. User-defined values may be useful for comparisons with defaults when site-specific conditions are better understood (Amini et al., Citation2012). LandGEM defaults, which include options for k based on annual precipitation, are shown in .LandGEM outputs results in terms of m3/yr for methane, LFG, and a number of contaminants of concern. As described in the following sections, L0 and k were identified for each of the following exercises by a weighted average of waste composition data.
Table 1. Default methane generation parameters for selected FOD models.
IPCC Waste Model
In an effort to quantify methane emissions from landfills worldwide, the IPCC developed the Waste Model for countries to report national inventories. It is equally capable of predicting methane generation from individual landfills because it only requires readily available data on methane generation inputs and annual tonnages. The Waste Model uses parameters of DOC and k, as shown in eqs 2 and 3, which incorporate several additional parameters to transform the C source to annual methane generation.
where QCH4 is the mass of methane generated per year; DDOCmi is the mass of degraded decomposable organic carbon of waste component i (Gg); and F is the fraction of methane in LFG.
where Mi is the mass of waste component i; DOCi is degradable organic carbon concentration of waste component i; DOCf is the fraction of anaerobically degradable carbon in landfill; and MCF is the methane correction factor.
The DOCf quantifies the percentage of DOC that will actually anaerobically decay (e.g., bioavailable cellulose, hemicellulose, fats, and proteins). The MCF is considered a landfill management factor that may be used to reduce the predicted methane generation of landfills that are partially aerobic. The Waste Model can predict methane generation from bulk MSW tonnages using regional defaults (single-phase) or apply component-specific DOCi values to user-defined waste composition data as a multiphase model (Govindan and Agamuthu, Citation2014). k values are identified for waste streams or waste components based on four selectable climates: wet and dry temperate and wet and dry tropical. The waste component values of DOC and k are identified in . Notice the k values for food waste range from 0.06 yr−1 (dry temperate) to 0.4 yr−1 (wet tropical), nearly a degree of magnitude difference based on climate.
IPCC reports methane generation from the DDOCm on a mass basis in gigagrams (1 Gg = 1000 Mg). To convert Gg CH4 to m3 CH4 per year, a unit conversion with methane density at standard temperature and pressure (STP) is required, as shown in eq 4.
Afvalzorg multiphase model
NV Afvalzorg developed the Afvalzorg multiphase model to predict methane emissions from several waste streams entering landfills in The Netherlands (Scharff and Jacobs, Citation2006). The European Union Landfill Directive prohibits untreated biodegradable waste from entering a landfill, and the model reflects those regulatory requirements (Mou et al., Citation2015). Therefore, many of the wastes typically disposed have low methane generation potentials (Mou et al., Citation2014). The waste streams are characterized by fractions of rapidly, moderately, and slowly decaying C; each fraction associated with a k value. The k values for rapid, moderate, and slow decay are calculated by half-lives of carbon, as shown in eq 5. This assumes the relative C bioavailability and type is equivalent for all waste fractions.
where k is the methane generation rate (yr−1) and t1/2 is the half-life of carbon (yr).
Similar to the IPCC’s MCF, Afvalzorg includes a dissimilation factor, Ϛ, to reflect nonideal conditions within landfills. The dissimilation factor has the effect of directly reducing the methane yield per tonne of waste. The default dissimilation factor is 0.7, indicating that only 70% of the methane potential is achieved. For all simulations presented in the following sections, Ϛ = 1. Equations 5–7 dictate the results of the Afvalzorg model.
where DOCjavailable is the available carbon in waste component j; Mi is the mass of waste component i; fDOCj is the fraction of DOC for waste component j; and DOCj is the waste component.
Afvalzorg reports annual methane generation in m3 CH4. Therefore, to report the annual methane generation, no additional calculations are required.
Australia’s multiphase Solid Waste Calculator
Australia’s Clean Energy Regulator developed the multiphase Solid Waste Calculator (SWC) to predict methane and GHG emissions from solid waste landfills in Australia, using DOC and k values identified by the IPCC (Clean Energy Regulator, Citation2014). k values are assigned by the location and climate of the state or territory of the landfill. Unlike other models, there is no option to enter user-defined values for these parameters. Like IPCC and to a lesser extent LandGEM, the k values may vary based on the climate where the landfill resides. The DOC and k values utilized by the SWC are identical to the IPCC values, although only three of the four are utilized, as shown in .
The percentage of each of three waste streams (MSW, Commercial and Industrial waste, and C&DD) relative to the annual landfilled mass is input to allow for annual characterization. Each of the three SWC waste streams is defined by default or user-defined percentages of the IPCC MSW components, including sludge. Therefore, the methane generation parameters DOC, DOCf, and k cannot be modified, but the amount of C disposed and methane generated per year can change according to the disposed percentages of food, wood, textiles, etc. For this reason, the SWC was not modeled with the Afvalzorg inputs but was modeled with the IPCC waste composition.
Results of the SWC were given tonnes CO2-equivalents (t CO2-eq). The Clean Energy Regulator identified a global warming potential of methane 21 times that of CO2 (Commonwealth of Australia, Citation2014). The equation to convert 2013–2014 SWC outputs into annual methane generation in cubic meters is given in eq 9.
Translating parameters
Each of the five FOD models outputs annual methane generation. In the case of multiphase models, the calculations are performed for each phase and summed for a single annual value. The predicted methane generation is related to the amount of anaerobically biodegradable C within the landfill. Each of the models uses similar parameters, and in some cases, translation of the parameters is necessary for input into other models. The international community has largely settled on syntax emphasizing DOC parameters rather than L0, which is used primarily in the United States and Canada (Thompson et al., Citation2009). As previously mentioned, the syntax is relatively unimportant because all parameters can be effectively translated between each model and all FOD models use the same fundamental equation that calculates methane generation on an annual basis from tonnes received in that year as well as tonnes of yet-undegraded waste in place. The IPCC published the translation of DOC to L0 using the other parameters incorporated in its Waste Model, as shown in eq 10 (IPCC, Citation2006). Each of the parameters directly impacts L0, and many researchers have varied these parameters to compare measured emissions from landfills, especially for unmanaged disposal sites where anaerobic conditions may not exist throughout (Irving et al., Citation1999; Jha et al., Citation2008; Jigar et al., Citation2014).
where DOC is degradable organic carbon (Mg C/Mg waste); DOCf is the fraction of DOC that will anaerobically decay (default = 0.5); MCF is the methane correction factor (default = 1); F is the fraction of methane in LFG (default = 0.5); 16/12 is the CH4/C mass ratio; 1000 is the conversion from Mg to kg; and 0.714 is the density of methane at STP (kg/m3).
In IPCC, methane generation can be modeled as a function of each waste component (e.g., food wastes, garden waste, etc.). In LandGEM, only a single waste stream can be input; therefore, the methane generation characteristics must reflect the entire waste stream. Although waste composition at many sites is unknown or incomplete, waste composition studies have been used to predict methane generation at landfills using component-specific parameters (Cho et al., Citation2012; Machado et al., Citation2009). Although IPCC provides a methodology for determining a bulk DOC based on waste composition (IPCC, Citation2000; Jensen and Pipatti, Citation2000), no guidance is given for a bulk DOCf or k calculation.
If waste composition is known in a single year, j, the weighted average of each of the MSW components with their respective methane-generating characteristics should describe the cumulative, bulk MSW stream for that year. Therefore, the multiphase characteristics can be translated to single-phase DOC, DOCf, and L0 by weighted-averaging each of the parameters as shown below.
where MSWi is the waste mass or percent mass of waste component i in year j; DOCf,i is the fraction of DOC that will anaerobically degrade in component i; j is the year in which waste is landfilled; and 1867 is the empirical unit conversion constant equivalent to 16/12 × 1000/0.714.
Equations 11 and 12 identify the weighted-average DOC and DOCf of a bulk waste stream in year j. Equation 13 adapts eq 10 to determine the proceeding L0. By this definition, the component sum of C, anaerobically degradable C, or potential methane will be identical to an identical mass of the weighted-average material, as shown in .
Figure 1. A single year’s waste composition for multiphase Afvalzorg waste streams (left) and IPCC waste components (right) can be weighted-averaged to a bulk waste stream (far right in each graph) that will contain equivalent methane generation parameters for use in a single-phase model. Afvalzorg waste composition data are from Glatved year 2004. IPCC waste composition data are from AV Miljø year 2000. Data reported by Mou et al. (Citation2015).
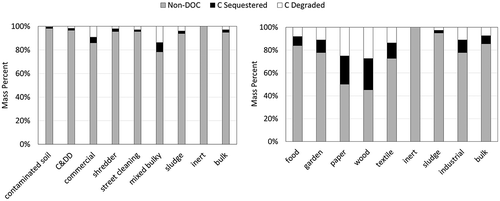
Unlike its three counterparts, Afvalzorg identifies multiple fractions of anaerobically degradable C within each waste stream as (1) rapidly, (2) moderately, or (3) slowly decomposing. These masses are attributed to rates (e.g., k1, k2, and k3), but more importantly here, quantify the total anaerobically degradable C that contributes to methane generation. These fDOC1,2,3 fractions can be summed to identify a DOCf applicable to the IPCC model, as shown in eq 14. Once the Afvalzorg fDOC is translated to DOCf, it and the DOC can be used to define L0 for LandGEM, as shown in eqs 11–13.
where fDOC1,2,3 is the fraction of DOC that is slowly, moderately, or rapidly degradable.
De la Cruz and Barlaz (Citation2010) previously reported a methodology for applying experimentally derived k values to field-scale waste composition data, shown in eq 15.
where f is the correction factor; klab,i is the laboratory-derived k value for waste component i; wt.fractioni is the mass percent of waste component i; and kfield,MSW is the field-scale k value for bulk waste mass.
If f is assumed to equal 1, the equation implies that the weighted average of all waste component k values equals the bulk MSW k. What is important to note is that this equation includes inert fractions of material because the mass percent is relative to the entire waste mass, not only the biodegradable fraction. k values of inert material would equal 0. Assuming f = 1, eq 15 can be rewritten to conform to the syntax used in eqs 11 and 12, as shown in eq 16.
where kwt,j is the methane generation rate calculated by bulk weight of waste in year j (yr−1) and ki is the methane generation rate of waste component i (yr−1).
The inclusion of inert material may not be appropriate because, unlike DOC, k should not be influenced by inert mass. As the multiphase models show, the rate of decay of one waste stream will not be impacted by another. Thus, a new method to calculate bulk k based on waste composition was developed to account for only the total mass of anaerobically degradable carbon, shown in eq 17.
where kC,j is the methane generation rate calculated by weight of carbon in waste in year j (yr−1).
As portrayed in , the fraction of carbon that will be degraded may be relatively small to the entire landfilled mass. In such a case, a simple weighted average (kwt) may skew the k value lower than would be expected in existing multiphase models. A simple example is presented in Supplemental Material to clarify the difference between eqs 16 and 17 and demonstrate the multiphase calculations.
As previously mentioned, Afvalzorg uses upwards of three k values per waste stream. A percentage of the waste mass is attributed to the corresponding rate (e.g., the mass of material MSWi × DOCi × fDOCi1 corresponds to k1 and mass of material MSWi × DOCi × fDOCi2 corresponds to k2). To calculate a single k value for each Afvalzorg waste stream that can then be used towards the weighted average of single-phase IPCC and LandGEM models, eq 14 is used where fDOC1,2,3 identifies the mass percent of each k value, as shown in eq 18.
Equations 11–14 and 16–18 identify waste characteristics for the bulk waste stream in year j defined by multiple MSW components. The weighted-averaging process is repeated for all j years to calculate characteristics that represent the waste stream across the life of the landfill (DOCa, DOCf,a, L0,a, and ka). In this way, waste characteristics of multiple waste streams from Afvalzorg or IPCC can be effectively translated to two parameters (L0 and k) suitable for input into LandGEM or three parameters (DOC, DOCf, and k) for a single-phase IPCC simulation, shown in eqs 19–23.
Exercise methodology
Annual waste composition data of four landfills located in Denmark were reported by Mou et al. (Citation2015). Composition was reported in terms of Afvalzorg waste streams as well as IPCC waste components. Weighted-average DOC, DOCf, L0, and k values were calculated based on the stream-specific (Afvalzorg) and component-specific (IPCC) methane generation parameters, creating two exercises with which to test the translations. Thus, two different simulations were modeled for each of the four landfills. Additionally, two k values were calculated and modeled with LandGEM: (1) a carbon weighted-average k (kC) introduced here and (2) a bulk weighted-average k (kwt) adopted from De la Cruz and Barlaz (Citation2010). The process flow diagram for the exercises of this research is shown in , which shows the use of each set of composition data in Exercise 1 with Afvalzorg waste streams and Exercise 2 with IPCC waste components. Because SWC methane generation parameters cannot be modified and the inputs are based on IPCC waste components, no modeling was performed comparing SWC with Afvalzorg and SWC was not used in Exercise 1. Because Afvalzorg waste streams do not conform to the IPCC component definitions, Afvalzorg was not modeled in Exercise 2. The primary objective was to compare predicted methane generation from multiphase and single-phase models, in order to answer the question: Are the predictions of cumulative and annual generation from single-phase models that use weighted-average and translated inputs similar to the predictions from multiphase models? A secondary objective was to compare the two methods previously described to calculate k by examining the year-to-year difference between the multi- and single-phase results.
Figure 2. Process flow diagram of the two exercises undertaken for each of four landfills, where (Exercise 1) Afvalzorg multiphase results were compared with single-phase LandGEM and IPCC results, and (Exercise 2) multiphase IPCC and SWC results were compared with single-phase LandGEM results.
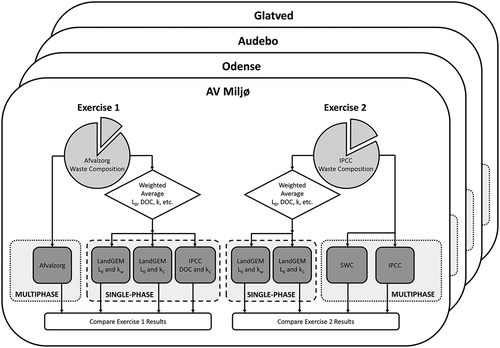
In Exercise 1, waste composition data based on Afvalzorg waste streams were used to develop weighted-average parameters suitable for input into single-phase LandGEM and IPCC models. The modeled results were compared with multiphase Afvalzorg predictions to examine the difference on a year-to-year basis as well as to compare cumulative methane generation predicted by each of the models. IPCC was modeled as a single-phase model using a single weighted-average DOC, DOCf, and kC. LandGEM was modeled with a weighted-average L0, kC, and kwt to examine the impact of the inert fraction on the generation rate. Weighted-average values were input with the total annual landfilled tonnages.
In Exercise 2, IPCC waste composition data presented by Mou et al. (Citation2015) were modeled in IPCC using the multiphase function including sludge and industrial waste and was compared with results of the single-phase LandGEM (using both k values) as well as SWC. MSW components “plastics” and “inert” identified by Mou et al. (Citation2015) were combined into an aggregated “inert” category. For all Exercise 2 simulations, DOCf = 0.5, F = 0.5, and MCF = 1 and the dry temperate climate was selected to establish baseline k values. In SWC, this corresponded to the wet temperate climate. For SWC modeling, the relative percentages of each MSW component per year were determined based on the IPCC MSW composition defined by Mou et al. (Citation2015). The industrial waste characterized by Mou et al. (Citation2015) (L0 = 92 m3/Mg and k = 0.09 yr−1) was modeled as 100% garden waste within the Commercial and Industrial waste stream. The IPCC default DOC for garden waste (0.2 g C/g waste) is similar to the Industrial waste stream identified by Mou et al. (Citation2015), although the k value is greater (wet temperate kgarden = 0.10 yr−1 compared with 0.09 yr−1). To model the sludge, the C&DD waste stream was characterized by 100% sludge. Weighted-average L0, kC, and kwt values were input into LandGEM with the total annual landfilled tonnages.
Statistical analyses
Annual methane generation of the models was compared by the sum of squared differences (SSD), as shown in eq 24. SSD compared the difference of modeled results on a year-to-year basis, with the lowest SSD identifying the best fit. An SSD of 0 would identify identical curves. SSD values are a quantification of differences of model predictions and were not evaluated at a specific confidence interval to qualify the goodness-of-fit. The curves with the lowest SSD are presented in Results and SSD calculations are included in Supplemental Material.
where Qj,multiphase is the methane generated in year j of the multiphase model (m3) and Qj,single-phase is the methane generated in year j of the single-phase model (m3).
Cumulative methane generation was compared at year 100 by percent difference. Each Microsoft Excel program calculated methane generation over a unique length of time: 80 yr (IPCC), 100 yr (Afvalzorg), 140 yr (LandGEM), and 269 yr (SWC). To assess cumulative methane generation comparatively, the IPCC model was replicated in a separate Excel file, the calculations were carried to 101 yr (years 0–100), and all results were evaluated after 100 yr (i.e., t = 100 yr) beginning the first year after waste placement (i.e., t = 1). This time span is consistent with solid waste life-cycle assessment (LCA) models (Manfredi and Christensen Citation2009) and is likely the upper limit of LFG generation modeling confidence.
Results
Exercise 1: Comparison with Afvalzorg waste composition
Methane generation parameters were combined according to waste composition and annual tonnages to identify suitable bulk values for input into single-phase FOD models LandGEM and IPCC. Based on the values shown in , cumulative methane generation from the single-phase models over 100 yr was calculated between −7% and +6% of the respective multiphase models. Annual methane generation results for Exercise 1 are reported in Tables S2–S5 (Supplemental Material).
Table 2. Bulk waste methane generation input parameters to the single-phase FOD models derived from Afvalzorg waste streams in Exercise 1.
AV Miljø waste was characterized by 26% shredder waste and 20% inert material, causing a low year-to-year DOC range from 0.012 to 0.065 Mg C/Mg waste and DOCf from 0.49 to 0.61. These corresponded to L0 values from 6 to 37 m3 CH4/Mg waste. kC values ranged from 0.057 to 0.075 yr−1 and kwt ranged from 0.023 to 0.053 yr−1. Cumulative methane generation was overpredicted by 2.5%, 1.2%, and 4.5% for LandGEM kC, LandGEM kwt, and IPCC, respectively. The IPCC and LandGEM kC models fit well until approximately 2010, at which point, overestimation occurred by a maximum of 20%. Overestimation continued (by a decreasing percentage) until 2040 when LandGEM kC and IPCC began underestimating generation compared with Afvalzorg. Although kwt caused underestimation before peak generation and overestimation thereafter, it was found to have the lowest SSD and therefore provided the best fit of the three.
Waste disposed at Audebo landfill had average DOC content ranging from 0.009 to 0.077 Mg C/Mg waste and DOCf ranging from 0.58 to 0.65. This corresponded to L0 values from 5 to 45 m3 CH4/Mg waste. kC ranged from 0.062 to 0.072 yr−1, whereas kwt ranged from 0.004 to 0.034 yr−1. IPCC and LandGEM kC overestimated initially by almost 40% but still fit better than the LandGEM kwt, which underestimated in the early years and overestimated for all later years.
Glatved waste was characterized by DOC content from 0.041 to 0.064 Mg C/Mg waste and DOCf from 0.52 to 0.62. As a result, L0 values ranged from 20 to 37 m3 CH4/Mg waste. kC values ranged from 0.062 to 0.069 yr−1, and kwt values were more varied, from 0.032 to 0.049 yr−1. Cumulative methane generation was greater than the Afvalzorg model by 5.8%, 3.5%, and 5.0% for the LandGEM kC, LandGEM kwt, and IPCC models, respectively. Modeling with the kwt parameter produced the lowest SSD, followed by the IPCC and LandGEM kC. This was despite underprediction from 1981 to 2010, followed by overprediction thereafter.
Odense waste contained 60% shredder waste. DOC content of the annual disposed waste stream was 0.032–0.057 Mg C/Mg waste, and DOCf ranged from 0.41 to 0.53. These corresponded to annual L0 values from 12 to 28 m3 CH4/Mg waste. kC ranged from 0.051 to 0.059 yr−1, and kwt from 0.045 to 0.053 yr−1. Odense landfill contained the least amount of inert waste stream but did not have the highest average L0. Cumulative methane generation was 5.8%, 3.5%, and 5.0% greater than Afvalzorg predictions for LandGEM kC, LandGEM kwt, and IPCC, respectively. LandGEM kwt had the lowest SSD, followed by IPCC, and finally LandGEM kC. displays the FOD curves from the multiphase and single-phase models from Exercise 1.
Figure 3. Exercise 1: Methane generation at four Danish landfills based on Afvalzorg waste composition data reported by Mou et al. (Citation2015).
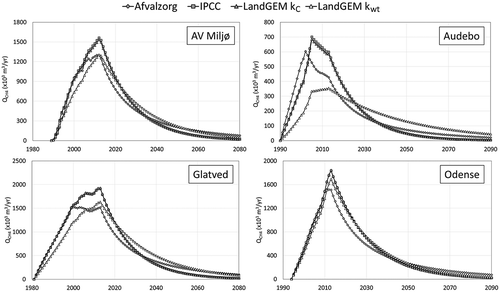
Exercise 2: Comparison with IPCC waste composition
The methane generation parameter values calculated using IPCC defaults for each of the four landfills, reported in , were greater than those defined by Afvalzorg waste streams. For AV Miljø, annual DOC values ranged from 0.113 to 0.183 Mg C/Mg waste. Annual kC values ranged from 0.077 to 0.088 yr−1, whereas kwt values ranged from 0.047 to 0.083 yr−1. kwt underestimated initially, and then overestimated, but provided the better fit for the single-phase approach. Using IPCC waste components to predict methane generation from AV Miljø landfill, cumulative methane generation was −1.1%, 0.2%, and 15.5% of the IPCC multiphase by LandGEM kC, LandGEM kwt, and SWC, respectively. The kC had the lowest SSD, indicating that it modeled the best fit to the IPCC multiphase.
Table 3. Bulk waste methane generation input parameters to the single-phase FOD models derived from IPCC waste components in Exercise 2.
Audebo waste was characterized by DOC from 0.104 to 0.159 Mg C/Mg waste. Annual kC values ranged from 0.059 to 0.086 yr−1, whereas kwt values ranged from 0.038 to 0.065 yr−1. At Audebo landfill, cumulative methane generation was 0.9%, −1.1%, and −14% from the IPCC multiphase by LandGEM kC, LandGEM kwt, and SWC, respectively. Modeling with the kC produced the lowest SSD, followed by SWC and kwt. The FOD curves in show the close fit of LandGEM kC to the IPCC multiphase.
Figure 4. Exercise 2: Methane generation at four Danish landfills based on IPCC waste composition data reported by Mou et al. (Citation2015).
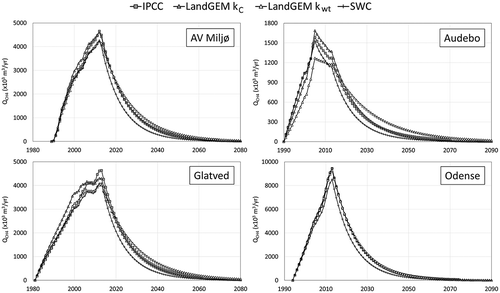
Annual Glatved DOC content ranged from 0.114 to 0.178 Mg C/Mg waste corresponding to L0 = 53–83 m3 CH4/Mg waste. kC ranged from 0.074 to 0.085 yr−1, and kwt ranged from 0.056 to 0.075 yr−1. Landfilled waste was 54% industrial and 20% inert material, with biodegradable MSW components constituting only 8% by mass. LandGEM kwt and SWC fit well to the IPCC multiphase initially, whereas LandGEM kC overpredicted. LandGEM kwt had the lowest SSD, followed by LandGEM kC, and then SWC. kwt fit the IPCC model slightly better than the SWC, and this is likely due to the higher garden waste k value that substituted for the industrial waste (equivalent DOC), which causes the methane generation to occur more quickly and results in continuous underestimation after landfill ceases.
Waste disposed at Odense landfill contained DOC concentrations from 0.181 to 0.198 Mg C/Mg waste, corresponding to L0 = 85–93 m3 CH4/Mg waste. kC ranged from 0.083 to 0.089 yr−1, and kwt ranged from 0.075 to 0.088 yr−1. Nearly 90% of the waste disposed was industrial, followed by inert (5%), biodegradable MSW components (4%), and sludge (3%). Similar to the Afvalzorg-defined composition, the inert fraction was the lowest among the landfills. LandGEM kC and LandGEM kwt fit well to the model throughout the 100 yr. The similar k values, reported in , made for a close comparison between the two methods; however, kwt had the lower SSD. SWC matched well for the first 10 yr but underpredicted for the remainder. Cumulative generation was 1.0%, 1.0%, and −18% of the IPCC multiphase model. The FOD curves using IPCC waste composition data for the four landfills are displayed in . Annual methane generation results for Exercise 2 are reported in Tables S6–S9 (Supplemental Material).
Discussion
Five Microsoft Excel–based landfill methane generation models were used to compare multiphase and single-phase predictions based on the FOD equation that has become universal for LFG modeling. Afvalzorg and SWC are considered multiphase models because they allow for simultaneous modeling of multiple waste streams with unique methane generation parameters, whereas LandGEM is only able to model a single waste stream. IPCC can model in both ways and is presented as two distinct models. Multiphase models employ the same FOD equation for each unique waste component and aggregate the calculated methane generation annually. The single-phase LandGEM calculates methane generation from only one waste stream annually.
Mou et al. (Citation2015) compared the ability of Afvalzorg, IPCC, and LandGEM to model low-methane-generating wastes using the default values of the respective models. The input waste tonnages for all four landfills were used in this research encompassing two exercises. In Exercise 1, default values from the Afvalzorg January 2015 multiphase model were used and eqs 19–23 were employed to calculate parameters suitable for input into single-phase FOD models IPCC and LandGEM.
Mou et al. (Citation2015) reported two waste compositions for each of the four landfills. One defined by Afvalorg waste streams and the second defined by IPCC waste components. In Exercise 2, the alternate IPCC waste composition was also modeled in multiphase IPCC and SWC and eqs 19–23 were used to calculate parameters for single-phase LandGEM. Thus, for each of the four landfills, two distinctive waste compositions resulted in two dissimilar methane generation curves.
Examining and , an inconsistency between the DOC and L0 values derived from the waste compositions exists. The DOC and L0 values used in the Afvalzorg model, shown in , are in many cases significantly lower than the IPCC waste components and partially account for the disparity. The lower DOC values calculated from the Afvalzorg waste streams can be further accounted for from a higher inert fraction compared with the defined IPCC composition (i.e., mass inertafvalzorg > mass inertIPCC, except Odense landfill). Mou et al. (Citation2015) noted the Afvalzorg model reflects the regulatory conditions in The Netherlands, where landfilling untreated biodegradable waste streams is restricted. Thus, it is not surprising that Mou et al. (Citation2015) reported significantly larger methane generation from LandGEM when using CAA default values compared with the Afvalzorg waste streams. However, that assessment is only valid for the regional default values, whereas translated parameters may more accurately compare model capabilities. Comparing the translated parameters here, the modeled results are much more similar than the order of magnitude differences reported by Mou et al. (Citation2015). It is also noteworthy that despite the low DOC values shown in , the DOCf remains greater than or equal to the IPCC default estimate of 0.5. This indicates that more than half of the landfilled C within the low-biodegradable wastes will generate methane.
In Exercise 1, although single-phase IPCC and LandGEM used the equivalent, translated input values, the percent differences and SSD values compared with the Afvalzorg multiphase model were similar but not identical. The truncation of significant digits from DOC (3 significant figures) and DOCf (2 significant figures) to L0 (2 significant figures) likely caused the minor discrepancy. The uncertainty inherent within the parameters suggests that precise values are not appropriate and should be limited to 2 or 3 significant figures at all times. Thus, the resultant L0, derived from the DOC and DOCf parameters as described in eq 21 and modeled in LandGEM, was rounded to the nearest single integer. Distinguishing L0 or any of the parameters between two similar values (e.g., L0 = 66 or 67 m3 CH4/Mg waste) inaccurately suggests that the confidence of the value is high and this precision may not be appropriate in all instances. In this research, because the waste composition is precisely defined by Mou et al. (Citation2015), there is higher confidence of the calculated values. However, when a single waste composition analysis is carried throughout a FOD model for the life of the landfill, the uncertainty of the values should be acknowledged.
To more accurately estimate methane generation from a landfill, previous studies have used waste composition to determine a site-specific L0 or DOC while selecting k based on local environmental conditions (Cho et al., Citation2012; Govindan and Agamuthu Citation2014; Sormunen et al., Citation2013). To compare modeled with measured values for several landfills, Amini et al. (Citation2012) calculated L0 and k was determined by best fit. Here, both L0 and k have been determined by waste composition to compare results of single-phase and multiphase models. Calculating k based solely on waste composition, similar to the calculation of DOC or L0, implies that L0 and k are directly related (Fei et al., Citation2015). However, research attempting to fit estimated gas generation to modeled results has shown that L0 and k are inversely related (Tolaymat et al., Citation2010). The suggestion that waste composition determines, at least partially, the k value would again identify a direct, rather than inverse, relationship with L0. What could be inferred from this logic is that the environmental conditions contributing to k would be the inverse relation and this factor would outweigh the composition-based calculation. This helps to explain why the kC provided the better fit for only three of the eight FOD curves compared with kwt. The incorporation of the inert fraction may reduce the direct influence of the waste composition calculation.
In many cases, the weighted-average values overpredicted and then underpredicted (or vice versa) compared with the multiphase values, so cumulative generation was still similar. Thus, the SSD analysis was necessary to compare results on an annual basis. DOC values for each year were calculated based on composition and then weighted-averaged based on tonnes disposed in that year to yield an overall DOC and L0 for the site (DOCa or L0a). The low percent difference of cumulative generation suggests that the DOCa and L0a methodology is appropriate. Because kC is not affected by inert waste streams, it was always greater than the kwt, although many times the kwt fit the multiphase curve better.
In Exercise 1, single-phase LandGEM and IPCC models were generally able to predict methane generation similarly to the Afvalzorg multiphase model. In Exercise 2, LandGEM and SWC predicted similar FOD curves to the multiphase IPCC model. The SWC does not allow for user-specified values, although the mass percentages work similarly to the IPCC model to create variability in the methane generation characteristics of the yearly landfilled waste. The region-specific DOC and k values identify the unique characteristics based on climate, regulatory, and economic considerations. As EPA’s AP-42 defaults may not accurately characterize waste from any other country, neither may the defaults established within the SWC, nor the IPCC bulk defaults for Africa, nor the waste streams in Afvalzorg. However, this does not diminish SWC’s importance for landfill operators and regulators in Australia. It only suggests that the model may not be suitable for predicting methane generation at landfills outside of Australia, whose waste may have different characteristics. Likewise, the initial conclusion drawn by Mou et al. (Citation2015), that the Afvalzorg model was most suitable for modeling low-methane-generating wastes, was an outcome of the comparison of regional default values, rather than the capabilities of the models. Afvalzorg performs several FOD calculations simultaneously, and the same results could be found modeling LandGEM several times with the appropriate L0 and k for each DOC, fDOC, and k combination and summing the results, something that was not attempted here.
Conclusion
Results from single-phase FOD models with weighted-average input parameters were compared with those from multiphase FOD models of heterogeneous waste streams. Mou et al. (Citation2015) reported two sets of waste composition data for four landfills in Denmark. In the first exercise, waste composition data based on Afvalzorg waste streams were used to determine weighted-average bulk DOC, DOCf, L0, and k values applicable to single-phase IPCC and LandGEM. In the second exercise, waste composition data based on IPCC waste components were used to determine bulk L0 and k values applicable to LandGEM. In both exercises, two k values were calculated for LandGEM models: the first, introduced here as a C-weighted k (kC), the other, based on the bulk weight of waste components, including the inert fraction (kwt).
Did the single-phase models predict similarly to the multiphase models? Single-phase IPCC and LandGEM were able to effectively model methane generation from heterogeneous wastes similar to the predictions of the multiphase models, generally ±7% of the predicted cumulative generation of a landfill over 100 yr. Did the kC improve single-phase predictions? The kC value was found to provide a better fit for only three of the eight landfill scenarios by SSD analysis. The inclusion of the inert material, which causes kwt to be less than kC, may frequently provide the better fit because of the inverse relationship that exists between L0 and k. In both exercises, it was shown that a single-phase FOD model is capable of predicting similarly to multiphase models when using the methodology described here to calculate weighted-average input parameters based on waste composition instead of comparing different regional default values. In the authors’ opinion, reducing the uncertainty of the input parameters, rather than incorporating more parameters or phases, will achieve greater accuracy to actual LFG generation.
Supplemental Material
Download Zip (156.4 KB)Acknowledgment
The authors recognize the valuable input of the reviewers, who gave excellent feedback to clarify the methods and message of the paper.
Funding
The authors would like to thank the New River Solid Waste Association and the University of Florida Engineering School of Sustainable Infrastructure and Environment for funding this research.
Supplemental Material
Supplemental data for this article can be accessed on the publisher’s website.
Additional information
Funding
Notes on contributors
Max J. Krause
Max J. Krause and Giles W. Chickering are graduate research assistants at the University of Florida in Gainesville, FL.
Timothy G. Townsend
Timothy G. Townsend is a professor of environmental engineering at the University of Florida, in Gainesville, FL.
References
- Amini, H.R., D.R. Reinhart, and K.R. Mackie. 2012. Determination of first-order landfill gas modeling parameters and uncertainties. Waste Manage. 32:305–316. doi: 10.1016/j.wasman.2011.09.021
- Cho, H.S., H.S. Moon, and J.Y. Kim. 2012. Effect of quantity and composition of waste on the prediction of annual methane potential from landfills. Bioresour. Technol. 109:86–92. doi: 10.1016/j.biortech.2012.01.026
- Clean Energy Regulator. 2014. Guideline: Solid Waste Calculator. Canberra, Australia. http://www.cleanenergyregulator.gov.au/NGER/Forms-and-resources/Calculators ( accessed July 8, 2015).
- Commonwealth of Australia. 2014. National Greenhouse and Energy Reporting Regulations 2008 (Superseded) F2014C01069. Canberra, Australia. www.comlaw.gov.au/ ( accessed July 8, 2015).
- De la Cruz, F.B., and M.A. Barlaz. 2010. Estimation of waste component-specific landfill decay rates using laboratory-scale decomposition data. Environ. Sci. Technol. 44:4722–4728. doi: 10.1021/es100240r
- Eleazer, W.E., W.S. Odle, Y.-S. Wang, and M.A. Barlaz. 1997. Biodegradability of municipal solid waste components in laboratory-scale landfills. Environ. Sci. Technol. 31:911–917. doi: 10.1021/es9606788
- Fei, X., D. Zekkos, and L. Raskin. 2015. Quantification of parameters influencing methane generation due to biodegradation of municipal solid waste in landfills and laboratory experiments. Waste Manage. doi: 10.1016/j.wasman.2015.10.015
- Govindan, S.S., and P. Agamuthu. 2014. Quantification of landfill methane using modified Intergovernmental Panel on Climate Change’s Waste Model and error function analysis. Waste Manage. Res. 32:1005–1014. doi: 10.1177/0734242X14552551
- Intergovernmental Panel on Climate Change. 2000. Waste. Good Practice Guidance and Uncertainty Management in National Greenhouse Gas Inventories. Geneva, Switzerland: Intergovernmental Panel on Climate Change.
- Intergovernmental Panel on Climate Change. 2006. Solid waste disposal. In 2006 IPCC Guidelines for National Greenhouse Gas Inventories, Vol. 5, Waste, ed. R. Pipatti and P. Svardal, 1–40. Geneva, Switzerland: Intergovernmental Panel on Climate Change.
- Irving, W., J. Woodbury, M. Gibbs, D. Pape, and V. Bakshi. 1999. Applying a correction factor to the IPCC default methodology for estimating national methane emissions from solid waste disposal sites. Waste Manage. Res. 17:459–464. doi: 10.1177/0734242X9901700609
- Jensen, J.E.F., and R. Pipatti. 2000. Background Paper: CH4 emissions from solid waste disposal. In Good Practice Guidance and Uncertainty Management in National Greenhouse Gas Inventories, ed. J.E.F. Jensen and R. Pipatti, 419–439. Geneva, Switzerland: Intergovernmental Panel on Climate Change.
- Jha, A.K., C. Sharma, N. Singh, R. Ramesh, R. Purvaja, and P.K. Gupta. 2008. Greenhouse gas emissions from municipal solid waste management in Indian mega-cities: A case study of Chennai landfill sites. Chemosphere 71:750–758. doi: 10.1016/j.chemosphere.2007.10.024
- Jigar, E., A. Bairu, and A. Gesessew. 2014. Application of IPCC model for estimation of methane from municipal solid waste landfill. J. Environ. Sci. Water Resour. 3:52–58.
- Karanjekar, R.V., A. Bhatt, S. Altouqui, N. Jangikhatoonabad, V. Durai, M.L. Sattler, M.D.S. Hossain, and V. Chen. 2015. Estimating methane emissions from landfills based on rainfall, ambient temperature, and waste composition: The CLEEN model. Waste Manage. doi: 10.1016/j.wasman.2015.07.03
- Kim, H., and T.G. Townsend. 2012. Wet landfill decomposition rate determination using methane yield results for excavated waste samples. Waste Manage. 32:1427–1433. doi: 10.1016/j.wasman.2012.03.017
- Machado, S.L., M.F. Carvalho, J.-P. Gourc, O.M. Vilar, and J.C.F. do Nascimento. 2009. Methane generation in tropical landfills: Simplified methods and field results. Waste Manage. 29:153–161. doi: 10.1016/j.wasman.2008.02.017
- Manfredi, S., and T.H. Christensen. 2009. Environmental assessment of solid waste landfilling technologies by means of LCA-modeling. Waste Manage. 29:32–43. doi: 10.1016/j.wasman.2008.02.021
- Mou, Z., C. Scheutz, and P. Kjeldsen. 2014. Evaluating the biochemical methane potential (BMP) of low-organic waste at Danish landfills. Waste Manage. 34:2251–2259. doi: 10.1016/j.wasman.2014.06.025
- Mou, Z., C. Scheutz, and P. Kjeldsen. 2015. Evaluation and application of site-specific data to revise the first-order decay model for estimating landfill gas generation and emissions at Danish landfills. J. Air Waste Manage. Assoc. 65:686–698. doi: 10.1080/10962247.2015.1008653
- Oonk, J., and A. Boom. 1995. Landfill Gas Formation, Recovery, and Emissions. Apeldoorn, The Netherlands. TNO Institute of Environmental and Energy Technology.
- Pan, J., and N. Voulvoulis. 2007. The role of mechanical and biological treatment in reducing methane emissions from landfill disposal of municipal solid waste in the United Kingdom. J. Air Waste Manage. Assoc. 57:155–163. doi:10.1080/10473289.2007.10465317
- Scharff, H., and J. Jacobs. 2006. Applying guidance for methane emission estimation for landfills. Waste Manage. 26:417–429. doi: 10.1016/j.wasman.2005.11.015
- Sormunen, K., T. Laurila, and J. Rintala. 2013. Determination of waste decay rate for a large Finnish landfill by calibrating methane generation models on the basis of methane recovery and emissions. Waste Manage. Res. 31:979–985. doi: 10.1177/0734242X13490980
- Stinson, J.A., and R.K. Ham. 1995. Effect of lignin on the anaerobic decomposition of cellulose as determined through the use of a biochemical methane potential method. Environ. Sci. Technol. 29:2305–2310. doi: 10.1021/es00009a023
- Thompson, S., J. Sawyer, R. Bonam, and J.E. Valdivia. 2009. Building a better methane generation model: Validating models with methane recovery rates from 35 Canadian landfills. Waste Manage. 29:2085–2091. doi: 10.1016/j.wasman.2009.02.004
- Tolaymat, T.M., R.B. Green, G.R. Hater, M.A. Barlaz, P. Black, D. Bronson, and J. Powell. 2010. Evaluation of landfill gas decay constant for municipal solid waste landfills operated as bioreactors. J. Air Waste Manage. Assoc. 60:91–97. doi: 10.3155/1047-3289.60.1.91
- U.S. Environmental Protection Agency. 1991. Code of Federal Regulations. Title 40 Part 258—Criteria for Municipal Solid Waste Landfills. Washington, DC. www.ecfr.gov ( accessed July 8, 2015).
- U.S. Environmental Protection Agency. 1996. Code of Federal Regulations. Title 40 Part 60 Subpart WWW—Standards of Performance for Municipal Solid Waste Landfills. Washington, DC. www.ecfr.gov ( accessed July 8, 2015).
- U.S. Environmental Protection Agency. 1998. Municipal solid waste landfills. In Compilation of Air Pollutant Emission Factors, Volume I: Stationary Point and Area Sources (AP-42), 1–19. Research Triangle Park, NC: U.S. Environmental Protection Agency.