ABSTRACT
To determine the impact of fireworks (FW) and firecrackers (FC) on particulate matter (PM) in ambient air, we reviewed evidence related to ambient PM during FW/FC periods; specifically, PM concentration, size, morphology, chemical components, including water-soluble ions and trace metals, and associated human health risks caused by exposure to FW/FC PM were reviewed. A large body of research suggests that outdoor ambient PM levels increase significantly during FW/FC displays. Furthermore, FW/FC PM remains suspended in the air, contributing to high PM concentrations for a long period. Increased PM from burning FW and FC mainly comprises fine and ultrafine spherical particles. Elevated levels of various trace metals, ions, elemental carbon (EC), organic carbon (OC), and organics in PM are present during FW/FC periods.Implications: Unique physical and chemical properties of ambient PM during short-term FW/FC burning can lead to a substantial increase in adverse health effects compared with during non-FW/FC periods. Further epidemiological and toxicological research into the potential health effects resulting from exposure to various pollutants from FW/FC is vital. Geographical distributions of PM concentrations during FW displays highlight the importance of implementing PM controls at the regional level and formulating stricter protective environmental legislation, particularly in Asian (e.g., India, China, or Taiwan) where festivals are not the only periods celebrated with FW/FC.
Introduction
Festivals worldwide are often accompanied by loud and brightly colored displays of fireworks (FW). Nationwide celebrations include Independence Day in the United States, the revolutionary overthrow of the monarchy in France, Las Fallas in Spain, November 5 in the United Kingdom, Lantern Festival and Spring Festival in China and Taiwan, Diwali Festival during October/November in India, and New Year’s Eve celebrations worldwide. In the United States, several thousand injuries, many of which are fatal, occur each year because of blasts in factories and stores as well as when consumers use FW (Health and Safety Executive, Citation2011). FW displays are high-intensity anthropogenic activities that cause short-term air-quality degradation, which can have significant public health implications. FW displays commonly generate dense smoke clouds that are full of particles (e.g., total suspended particulate matter [TSP], and particulate matter [PM]: PM10 and PM2.5) with water-soluble ions and trace metals (Kulshrestha et al., Citation2004; Drewnick et al., Citation2006; Moreno et al., Citation2007; Wang et al., Citation2007; Vecchi et al., Citation2008; Camilleri and Vella, Citation2010; Perrino et al., Citation2011; Chang et al., Citation2011; Tsai et al., 2012; Crespo et al., Citation2012).
Bach et al. (Citation1975) observed that FW activities on New Year’s Eve in Oahu, HI (United States), were responsible for a threefold increase in TSP (on average) at 14 locations and a sevenfold increase in lung-penetrating TSP at one location. Ravindra et al. (Citation2003) reported that FW during the Diwali festival led to a two- to threefold increase in PM10 and TSP concentration in Hisar City, India. Yang et al. (Citation2014) demonstrated that the widespread burning of firecrackers (FC) clearly contributed to the number concentration of particles in the small accumulation mode (100–500 nm) and PM2.5 mass concentration, with a 14-fold maximum PM2.5 concentration increase in Jinan (China) during the 2008 Chinese New Year. The extremely high PM concentrations may be due to meteorological factors such as low night temperature, low mixing height, and low wind speed (Thakur et al., Citation2010).
In addition to PM concentrations changing during FW display periods, the composition of PM varies considerably. Vecchi et al. (Citation2008) found that FW displays during the FIFA World Cup 2006 celebration increased the concentration of metals, such as Sr (120-fold), Mg (22-fold), K (12-fold), Ba (11-fold), and Cu (6-fold), in ambient PM10 in Milan, Italy. Yang et al. (Citation2014) reported higher ion concentrations in PM2.5, including K+ (33-fold), Mg2+ (21-fold), Cl− (12-fold), SO42 − (fourfold), F− (threefold), and Na+ (threefold), during FC periods than those during non-FC periods. Nevertheless, little is known of their acute cardiorespiratory toxicity. An online analysis of individual aerosol particles by Carranza et al. (Citation2001) showed that order-of-magnitude increases in Mg and Al mass concentrations were observed during the holiday period and were attributed directly to the discharge of FW.
Few studies have addressed the potential adverse health effects directly related to inhalation exposure to FW/FC emissions. Smith et al. (Citation1975) noted that the pulmonary function monitored in nine patients (seven healthy and two with chronic respiratory disease) exposed to a sixfold increase in PM10 (an average of 110 μg m−3 over a 5-hour experiment, which included a 15-min peak attaining concentrations in excess of 3.8 mg m−3) from FW events showed a significant decrease in the maximal mid-expiratory flow rate of susceptible individuals. Hirai et al. (Citation2000) found that inhaling smoke from FW causes cough, fever, and dyspnea, leading to acute eosinophilic pneumonia. Burning of FC and sparklers engendered increased loading effects in human health, which were particularly severe in infants, women, and elderly people (Kannan et al., Citation2004). Yang et al. (Citation2014) reported that pollutants from burning FC—even over a brief period—poses a high noncarcinogenic risk to human health. In addition to the direct effects of FW/FC on the composition of particulate matter (PM), other indirect effects may require special attention. One of the indirect effects from FW is groundwater and surface-water contamination by perchlorate, which can have an adverse ecological impact and inhibit the iodide uptake of the thyroid gland by PM deposition through precipitation (Backus et al., Citation2005; Kosaka et al., Citation2007; Wilkin et al., Citation2007; Munster et al., Citation2009; Sijimol and Mohan, Citation2014). During festivals, an increase in the cooking of meat at homes, restaurants, and night markets coincides with the burning of FW/FC, particularly in Asia. In Taiwan, Zhao et al. (Citation2011) observed higher concentrations of polycyclic aromatic hydrocarbons (PAHs) at night markets where cooking is frequent, particularly during festivals. Li et al. (Citation2009) reported markedly higher concentrations of PAHs in the nighttime than those in the daytime during the Spring Festival in China, mostly caused by an increase in coal combustion (i.e., from cooking) at night when the relative humidity (RH) is high and wind speed is low.
Local reviews of ambient air and noise quality have been conducted, such as in India during the Diwali festival FW period (Verma and Deshmukh, Citation2014). However, we found no global review of the impact of FW/FC on ambient PM. Accordingly, the primary objective of this paper is to review and summarize current scientific evidence on the effects of FW on ambient PM—specifically, PM concentration, size, morphology, and chemical components including water-soluble ions and trace metals—and associated human health risks attributable to exposure to FW PM. The present review evaluates the strength and consistency of current research about the effects of FW/FC on the concentration and composition of ambient PM and related human health problems. The review also highlights key knowledge gaps regarding the increased risks caused by FW/FC PM and suggests priority topics and strategies for future research.
Results of previous research
This review includes information on 49 references of primary relevance from over 200 articles and reports. lists the key features of these 49 studies, including the sampling design pattern, PM types and compositions, and health risks. Sampling at or near FW display sites or in urban areas (41 with patterns A, B, and C) was performed in 49 reviewed studies. Sampling far away (>10 km) from display sites or urban areas (five with Pattern D) renders quantifying the impacts of FW on ambient PM difficult. Although indoor laboratory sampling (two with Pattern E) can be used to characterize chemical properties, the results of such characterizations do not indicate the actual ambient conditions and indirect impacts.
Table 1. Key features of 49 studies of primary relevance to the effect of fireworks on ambient PM.
Tables S-1 and S-2 (supplemental file) provide additional details on these 49 studies including the sample quantity and frequency, sampling locations (distance from the source), and measured particle components.
Concentrations, sizes, and morphology
A large body of evidence suggests that FW displays have a significant impact on ambient PM levels. Among the 49 references of primary relevance, 41 have reported PM concentrations during the FW period.
lists PM concentrations and their elevation times during FW periods relative to pre-FW or normal ambient levels. Numerous studies have suggested that outdoor ambient PM levels increase by several to many times during FW displays compared with normal or non-FW PM levels. Nineteen of 20 PM2.5-related studies have reported average PM2.5 levels that exceed the 24-hr U.S. National Ambient Air Quality Standards (NAAQS) of 35 μg m−3, and 19 of 25 PM10-related studies have reported average levels of PM10 that exceeded the 24-hr U.S. NAAQS of 150 μg m−3.
Table 2. Ambient PM concentrations (μg m−3) reported during fireworks and their elevation times relative to nonfirework period.
PM during FW/FC appears to be strongly influenced by local conditions (i.e., proximity of the monitoring sites to the display), cultural diversity (which determines the quantity and intensity of FW), local air pollution, and meteorological factors (Perry, Citation1999; Drewnick et al., Citation2006; Vecchi et al., Citation2008; Walsh et al., Citation2009; Joly et al., Citation2010). For example, when samples were measured within a predicted plume location in Canada, peak PM2.5 levels reached nearly 10,000 μg m−3, approximately 1000-fold higher than background levels (Joly et al., Citation2010). Additionally, there are evident differences among celebrations around the world. and present the median PM concentrations during FW displays and ambient PM concentrations during normal days in three geographical areas (i.e., India, China, and Taiwan; Europe; and North America). Ambient concentrations of both PM2.5 and PM10 are apparently the highest (101 μg m−3 and 119 μg m−3) in India, followed by China and Taiwan (54 μg m−3 and 83 μg m−3), and the lowest in Western countries (12 and 14 μg m−3). Although the elevation times relative to ambient background levels in India are lower (4.4 μg m−3 for PM2.5 and 4.6 μg m−3 for PM10) than those in Western countries (9.1 μg m−3 for PM2.5 and 5.4 μg m−3 for PM10), the absolute increase in both PM2.5 and PM10 concentrations in India attributable to celebrations was substantial compared with that in Western countries, indicating the importance of implementing PM controls at the regional level in India.
Figure 1. Geographical distribution of PM2.5 concentrations during firework period. “Median” refers to median concentrations during firework period. “Background” refers to ambient concentrations during normal nonfirework period. Numbers above each column group are elevation times of PM concentrations relative to ambient background levels.
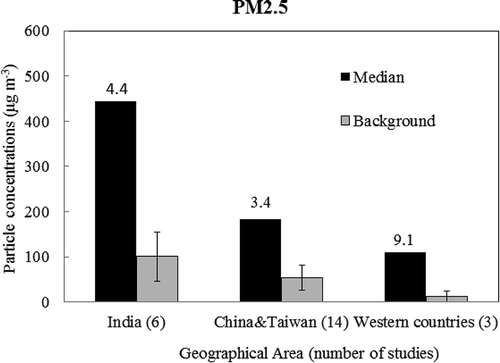
Figure 2. Geographical distribution of PM10 concentrations during firework period. “Median” refers to median concentrations during firework period. “Background” refers to ambient concentrations during normal nonfirework period. Numbers above each column group are elevation times of PM concentrations relative to ambient background levels.
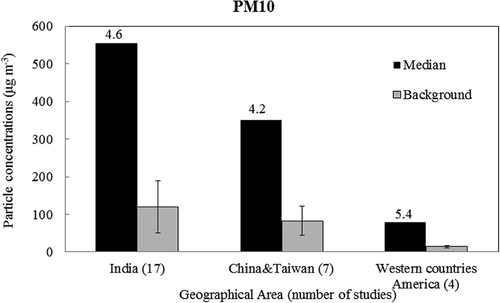
Strong evidence suggests that FW PM remains suspended in the air for a week or even up to 1 month after festival displays, causing high PM concentrations for a prolonged period (Perry, Citation1999; Ravindra et al., Citation2003; Sarkar et al., Citation2010; Agrawal et al., Citation2011; Kong et al., Citation2015).
Numerous studies have suggested that PM2.5/PM10 ratios increase during FW periods compared with pre- and post-FW periods, and primary aerosols from burning FW mainly release fine and ultrafine particles (100–500 nm) (Moreno et al., Citation2007; Wang et al., Citation2007; Vecchi et al., Citation2008; Godri et al., Citation2010; Zhang et al., Citation2010; Chang et al., Citation2011; Majumdar and Nema, Citation2011; Huang et al., Citation2012; Tsai et al., 2012; Beig et al., Citation2013; Nirmalkar et al., Citation2013; Baranyai et al., 2014; Jiang et al., Citation2014; Lin et al., Citation2014; Tian et al., Citation2014; Yang et al., Citation2014; Kong et al., Citation2015; Pathak et al., Citation2015), which can lead to a substantial increase in toxicity and short-term public health concerns (Dockery et al., Citation1993; Pope et al., Citation1995; Schwartz et al., Citation1996; Do et al., Citation2012; Tiwari et al., Citation2012; Beig et al., Citation2013).
Only three studies have addressed the morphology of FW PM (Wang et al., Citation2007; Agrawal et al., Citation2011; Li et al., Citation2013). Evidence shows that particles from burning FW are mostly spherical (Wang et al., Citation2007; Agrawal et al., Citation2011). Further detailed analysis by energy-dispersive x-ray spectroscopy suggests that the shape of various metal-bearing particles actually varies. The shape of K-rich particles is chain-like during FW periods, and they become round particles during post-FW periods because of aging processes during atmospheric transport (Li et al., Citation2013). Other metal-bearing particles, such as Al, Mg, and Fe, are mostly round in shape.
Metals
Among the 49 reviewed articles, 25 reported increased metal levels in PM during FW periods. Reactive metals (i.e., Mg, Al, Fe, and Zn), which function as fuels in FW, are used to make the FC brighter and more colorful (Shimizu, Citation2004; Steinhauser and Klapotke, Citation2008). Oxidants (i.e., nitrate or perchlorate salt) are usually included because atmospheric oxygen cannot support rapid combustion. Electron excitation of metal ions (i.e., Ba, Sr, Na, and Cu) at high temperatures during combustion results in colored displays. For example, Sr, Ba, and Cu compounds are used to give red, green, and blue fireworks, respectively (Kulshrestha et al., Citation2004; Wang et al., Citation2007; Moreno et al., Citation2007). Additional compounds, such as organic dyes (for smoke generation), metal salicylates (for noise effects), and chloride salts or chlorinated organics (for color enhancement), can be added for specialized functions (Shimizu, Citation2004). Fe facilitates the production of sparks, whereas Ca deepens the colors produced by other compounds (Kulshrestha et al., Citation2004).
presents metal concentrations during FW period and their increased ratios over normal days. It is shown that concentrations of K, Mn, Ba, Al, Mg, and Sr increased relative to normal days based on all peer-reviewed literature, and especially increased ratios of K, Ba, and Sr during FW displays were consistently remarkable (i.e., 167, 350, and 466 at the highest, respectively), suggesting that they can be used as FW tracers. Other metals that also increased during FW displays in most of literature include Cu, Zn, Pb, Cd, Cr, and Fe, although they were sometimes reported to decrease or be comparable to normal days. Other seldom-reported metals that also increased when burning FW include Co (Barman et al., Citation2008; Chatterjee et al., Citation2013; Crespo et al., 2014), V (Dutcher et al., Citation1999; Perry, Citation1999; Perrino et al., Citation2011; Chatterjee et al., Citation2013), Ti (Dutcher et al., Citation1999; Perry, Citation1999; Joly et al., Citation2010; Perrino et al., Citation2011), Ni (Barman et al., Citation2008), Hg (Thakur et al., Citation2010), Bi and Ga (Perrino et al., Citation2011), Sb (Camilleri and Vella, Citation2010; Croteau et al., Citation2010), Na (Tandon et al., Citation2008), and Ca (Dutcher et al., Citation1999), among which Sb is a potential carcinogen that is prohibited in consumer FW in the United States (Croteau et al., Citation2010).
Table 3. Metal concentrations (μg m−3) during FW and their increased ratios over normal days.
Water-soluble ions
Among the 49 reviewed articles, 20 studies noted elevated concentrations of water-soluble ions in ambient aerosol particles during and shortly after FW displays. Water-soluble ions are critical parts of PM chemical compositions that facilitate understanding the impact of FE burning and potential effects on human health. Sufficient evidence demonstrates that water-soluble ions contribute to more than 50% of PM2.5 mass (Yang et al., Citation2014).
The relative abundance of water-soluble ions, such as sulfate, nitrate, ammonium, and other species, has a critical influence on the hygroscopicity of atmospheric aerosols (Tang et al., Citation1995). The ability of water-soluble ions to absorb water at a high relative humidity (RH) can exacerbate visibility impairment (Dougle et al., Citation1996; Lee et al., Citation2005; Yuan et al., Citation2006).
shows the concentrations of water-soluble ions during FW and their increased ratios over normal days. High concentrations of various water-soluble ions are common during FW displays; especially K+, Mg2+, Cl−, and SO42− increased the most during FW periods relative to normal days. Feng et al. (Citation2012) reported that the K+ concentration reached 81.2 μg m−3, followed by SO42− (59.6 μg m−3) and Cl− (51.7 μg m−3). The increased ratios of K+, Cl−, Mg2+, and SO42− during fireworks over normal days were 71.4, 56.6, 23.9, and 12.5, respectively, while for NO3−, NH4+, and Ca2+ were only 2.3, 2.1, and 0.9. Among all detected water-soluble ions, K+ was considered a reliable indicator of PM generated by FW burning (Drewnick et al., Citation2006; Wang et al., Citation2007; Shen et al., Citation2009; Chang et al., Citation2011; Feng et al., Citation2012; Rao et al., Citation2012; Tsai et al., 2012; Ambade and Ghosh, Citation2013; Chatterjee et al., Citation2013; Pachauri et al., Citation2013; Cheng et al., Citation2014; Lin et al., Citation2014; Tian et al., Citation2014; Yang et al., Citation2014). High concentrations of other ions, such as Mg2+, Ca2+, and Na+, are often detected during FW periods (Wang et al., Citation2007; Feng et al., Citation2012; Rao et al., Citation2012; Tsai et al., 2012,Chatterjee et al., Citation2013; Ambade and Ghosh, Citation2013; Cheng et al., Citation2014; Crespo et al., 2014; Lin et al., Citation2014). Increased Cl−/Na+ ratios and decreased Cl−/K+ ratios of ambient fine particles were sometimes suggested to be indicators of FW burning (Tsai et al., 2012; Pachauri et al., Citation2013).
Table 4. Concentrations of water-soluble ions (μg m−3) during FW and their increased ratios over normal days.
During post-FW periods, Cl− is replaced by SO42- and NO3−, and secondary inorganic ions (SO42-, NO3−, and NH4+) increase gradually, becoming major components (Li et al., Citation2013; Yang et al., Citation2014; Kong et al., Citation2015; Huang et al., Citation2012). Huang et al. (Citation2012) reported that SO42-, NO3−, and NH4+ were 5.3, 7.2, and 5.5 μgm−3 during the preholiday pollution episode, but increased to 19.2, 10.2, and 13.4 μg m−3 during the postholiday episode. Therefore, selecting the appropriate sampling time and site is critical. In certain scenarios, when the sampling site is far from the display site, high levels of SO42-, NO3−, and NH4+ and increased NO3−/SO42- ratios may be indicators of PM from FW (Shen et al., Citation2009; Feng et al., Citation2012; Rao et al., Citation2012; Chatterjee et al., Citation2013; Ambade and Ghosh, Citation2013; Pachauri et al., Citation2013; Cheng et al., Citation2014; Yang et al., Citation2014; Kong et al., Citation2015). In other scenarios, increased Cl− levels and no change in SO42-, NO3−, or NH4+ are also credible indicators when sampling fresh FW particles (Drewnick et al., Citation2006; Wang et al., Citation2007; Chang et al., Citation2011; Feng et al., Citation2012; Huang et al., Citation2012; Tsai et al., 2012; Lin et al., Citation2014).
Others: Organic carbon (OC), elemental carbon (EC), PAHs, and organics
Among the 49 reviewed articles, 12 have reported EC and OC, 5 have reported PAHs, 6 have reported black carbon, and 10 have reported organics in PM during FW periods.
Carbonaceous species (i.e., OC and EC) are a crucial contributor to particle components. Numerous studies have suggested that OC increases significantly and EC increases marginally during FW burning compared with non-FW periods, and higher OC/EC ratios of PM can be an indicator of particles from FW burning because they are consistently higher than those observed in particles in urban areas (Vecchi et al., Citation2008; Feng et al., Citation2012; Huang et al., Citation2012; Tsai et al., 2012; Pachauri et al., Citation2013; Tian et al., Citation2014; Kong et al., Citation2015). Only one study showed a slight increase in OC and decrease in EC during FW burning (Cheng et al., Citation2014). The authors speculated that the FW contribution of EC was less crucial compared with the reduction in EC emissions from other sources, namely, vehicle exhausts. During post-FW periods, OC levels become even higher than those during FW periods, which could be due to the increased levels of secondary organic carbon (SOC) (Sarkar et al., Citation2010; Jiang et al., Citation2014; Yang et al., Citation2014).
Extant studies have suggested that PAHs are not directly from FW displays (Li et al., Citation2009; Sarkar et al., Citation2010; Feng et al., Citation2012; Shi et al., Citation2014). Only one indoor laboratory study (Croteau et al., Citation2010) revealed that during FW burning, formation of low-molecular-weight PAHs, such as naphthalene and acenaphthylene, were favored; however, this does not reflect actual ambient conditions. Biomass and coal burning and traffic are the two major sources of ambient PAHs; therefore, the direct contribution of FW to PAHs is negligible if any (Li et al., Citation2009; Sarkar et al., Citation2010; Feng et al., Citation2012; Shi et al., Citation2014). However, PAHs are indirectly influenced by biomass combustion, which is affiliated with FW displays.
Previous studies have consistently demonstrated that FW displays are a significant source of organics (Drewnick et al., Citation2006; Huang et al., Citation2012; Nishanth et al., Citation2012; Jiang et al., Citation2014; Kong et al., Citation2015). One study even found various hazardous organic compounds, including aliphatic compounds and derivatives, halogenated aliphatic, organic acids/ester, and alcohols/ketones, in PM during FW periods (Nishanth et al., Citation2012). However, the findings of several studies have suggested that the level of alkanes, aromatic organics, fatty acids, and dicarboxylic acids other than PAHs do not change during FW burning (Perry et al., Citation1999; Wang et al., Citation2007; Li et al., Citation2009; Agrawal et al., Citation2011; Feng et al., Citation2012).
Health risks
Respirable particulates, which have an aerodynamic diameter of less than 10 μm, are a crucial part of the atmosphere. When present at a level of over 50 μg m−3, PM10 has an adverse effect on human health (World Health Organization, Citation2006); an epidemiological study by Pope (2000) suggested that even a low level of exposure leads to an increased risk of cardiopulmonary disease, stressed respiratory physiology, mortality, and morbidity. Higher concentrations of PM10 in inhaled air increase the likelihood of these particles reaching deep into the lungs. Previous studies have suggested that these effects present as an increase in mortality with each incremental increase of PM10 by 10 μg m−3. People who are elderly or have past history of lung or heart disease may be more susceptible to the adverse effects of PM10 (Dockery et al., Citation1993; Schwartz et al., Citation1996).
Among the reviewed articles, only a few have reported health risk evaluations directly related to FW PM. Smith et al. (Citation1975) presents the first direct evidence that fireworks air pollution in Honolulu can alter pulmonary function in susceptible people. They also speculated that the 26% decrease in maximal midexpiratory flow rate (FEV 25-75%) in susceptible people was the result of an SO2–KCl aerosol interaction. Thakur et al. (Citation2010) estimated the probable health impact of short-lived deterioration of the ambient air quality by PM through Monte Carlo’s simulation in terms of increase in relative risk of mortality and morbidity in exposed individuals and found it to be extremely high.
Furthermore, two studies have suggested that fine and ultrafine particles generated by FW burning are more toxic and appear to have a more negative health effect compared with coarse particles from FW burning. In the study of Do et al. (Citation2012), the cytotoxicity response data for the size-fractionated airborne particulate matter collected from the pre- and postfireworks display periods was evaluated in Yanshui, Taiwan. The samples collected in the prefireworks display period were found to be less toxic than those in the postfireworks display period. Moreover, the levels of the elements collected in the submicrometer size range were less than half of those in the micrometer size range in the postfireworks display period, but the viability was 65.7% for the former compared with 73.3% for the latter. Another study by Beig et al. (Citation2013) shows increase in the numbers of excess cases of mortality and morbidity attributed to effective population exposure to PM2.5 and PM10 mass concentrations within areas of 2 km radii associated with the fireworks displays. It also indicates the excess number of cases for total, cardiovascular, and respiratory mortalities and hospital admissions may be related to inhalation of large number of smaller particles (PM2.5) rather than mass concentrations of coarser particles (PM10–2.5).
Accumulation of metals adversely affects human health and the ecosystem when present in high concentrations. High levels of metals can induce severe health effects, such as neurological and hematological effects, on exposed populations, particularly the effect of Pb on children, the carcinogenic effects of inhaled Cd and Ni on humans with chronic lung diseases, the toxic and carcinogenic effects of Cr on the bronchial tree, increased neurotoxic impairment from Mn, and respiratory irritation from increased Cu levels (Benoff et al., Citation2000; Santos-Burgoa et al., Citation2001; ATSDR, Citation2002; Hu, Citation2002; Manalis et al., Citation2005).
Little evidence is available on the links between exposure to metals in PM from FW and health risks. Only three robustly designed experimental studies have assessed carcinogenic and noncarcinogenic hazard health risks caused by exposure to heavy metals during FW burning. Sarkar et al. (Citation2010) estimated that chronic exposure to Diwali pollution is likely to cause at least a 2% increase in noncarcinogenic hazard index associated with Al, Mn, and Ba in the exposed population. Yang et al. (Citation2014) also indicated that exposure to metals found in PM2.5 may pose a serious public health risk in this study area and that the noncarcinogenic elemental inhalation risk is greatly increased due to the burning of firecrackers. In addition, among all analyzed metals, Cu and Pb increased the most. Their results of a comparison between children and adults showed that children were the most sensitive group to noncarcinogenic effects and should avoid possible exposure to these contaminants. However, Kong et al. (Citation2015) pointed out that the noncancer risks raised just by FW at Chinese New Year’s Eve indicated no adverse health effects, while the cancer risk suggested the FW particles were important carcinogens and should be controlled effectively, especially for the contained Cd and As.
It is crucial to note that only health risks directly associated with concentration and compositions of PM are reviewed in this paper; the actual health effects should be far more severe because of exposure to high levels of other pollutants generated by FW in addition to the acute effects of FW particles and associated metals.
Conclusions and research needs
The following conclusions were drawn from this review:
Outdoor ambient PM levels increase significantly during FW/FC burning.
FW PM remains suspended in the air for up to 1 month after festival displays, causing high PM concentrations for a long period.
Increased PMs from FW/FC burning are mainly fine and ultrafine spherical particles.
PM during FW/FC burning is strongly influenced by local conditions (i.e., distance between the monitoring sites and the display, cultural diversity, local air pollution, and meteorological factors).
Abnormally high levels of numerous trace metals, ions, EC, OC, and organics in PM during FW/FC periods are sufficiently documented.
Fine and ultrafine particles generated by FW burning are more toxic and appear to have a more negative health effect compared with coarse particles. However, there is a significant lack of information about health effects caused by PM from FM/FC.
In general, the information from this review is not sufficient to develop an emission inventory of the source because the ambient data is not comprehensive in the species, emission factors are not available, and there is incomplete information about the spatial/temporal occurrence of this emission source. Thus, the following observations and recommendations for future research are suggested:
Unique properties of ambient PM during short-term FW/FC burning relative to non-FW periods, such as increased PM levels, dominant fine and ultrafine particles, varied PM chemical components, and elevated levels may lead to a substantial increase in health risks. However, extant literature related to health risks associated with FW/FC is sparse and generally insufficient to draw compelling conclusions regarding specific health implications. Additional epidemiological and toxicological studies on the potential health effects from exposure to various pollutants from FW/FC burning are vital to ensure that at-risk populations take precautionary measures.
Geographical distributions of PM concentrations during FW displays highlight the importance of implementing PM controls at the regional level and formulating stricter protective environmental legislation, particularly in Asia (e.g., India, China, or Taiwan) where festivals are not the only periods celebrated with FW/FC.
Some studies show that fine and ultrafine particles generated by FW burning are more toxic, but confirming the effects of particle sizes on respiratory epithelial cells will require further studies. Besides, further estimations based on coarser and fine particles mass concentrations are warranted to develop exposure series of each of the pollutants emitted due to fireworks displays and effective population exposure as per population census.
UAWM_A_1219280_Supplementary_material.pdf
Download PDF (21 KB)Funding
The author thanks the Ministry of Science and Technology of the Republic of China for funding the corresponding author to visit Queensland University of Technology (NSC 99-2911-I-390-002-2), and Prof. Lidia Morawska for inspiring the corresponding author’s interest in particle-related research. Gratitude is also expressed to Dr. Ping Zhao and Miss Yu-Chia Lin for their assistance in collecting some of the literature.
Supplemental data
Supplemental data for this article can be accessed on the publisher’s website.
Additional information
Funding
Notes on contributors
Chi-Chi Lin
Chi-Chi Lin is an associate professor in the Department of Civil and Environmental Engineering at National University of Kaohsiung.
References
- Agency for Toxic Substances and Disease Registry. 2002. Toxicological profile of Copper. Atlanta, GA: ATSDR, Division of Toxicology.
- Agrawal, A., V.K. Upadhyayl, and K. Sachdeva. 2011. Study of aerosol behavior on the basis of morphological characteristics during festival events in India. Atmos. Environ. 45(21):3640–44. doi:10.1016/j.atmosenv.2011.04.006
- Ambade, B., and S. Ghosh. 2013. Characterization of PM10 in the ambient air during Deepawali festival of Rajnandgaon district, India. Nat. Hazards 69:589–98. doi:10.1007/s11069-013-0725-8
- Bach, W., A. Daniels, L. Dickinson, F. Hertlein, J. Morrow, S. Margolis, and V. Dinh. 1975. Fireworks pollution and health. Pol. J. Environ. Stud. 7:183–92. doi:10.1080/00207237508709692
- Backus, S.M., P. Klawuun, S. Brown, I. D’sa, S. Sharp, C. Surette, and D.J. Williams. 2005. Determination of perchlorate in selected surface waters in the Great Lakes Basin by HPLC/MS/MS. Chemosphere 61:834–43. doi:10.1016/j.chemosphere.2005.04.054
- Barman, S.C., R. Singh, M.P. Negi, and S.K. Bhargava. 2008. Ambient air quality of Lucknow City (India) during use of fireworks on Diwali festival. Environ. Monit. Assess. 137:495–504. doi:10.1007/s10661-007-9784-1
- Baranyai, E., E. Simon, M. Braun, B. Tóthmérész, J. Posta, and I. Fábián. 2015. The effect of a fireworks event on the amount and elemental concentration of deposited dust collected in the city of Debrecen, Hungary. Air. Qual. Atomos. Health. 8:359–65. doi:10.1007/s11869-014-0290-7
- Beig, G., D.M. Chate, S.D. Ghude, K. Ali, T. Satpute, S.K. Sahu, N. Parkhi, and H.K. Trimbake. 2013. Evaluating population exposure to environmental pollutants during Deepavali fireworks displays using Air Quality Measurements of the SAFAR network. Chemosphere 92:116–24. doi:10.1016/j.chemosphere.2013.02.043
- Benoff, S., A. Jacob, and I.R. Hurley. 2000. Male infertility and environmental exposure to lead and cadmium. Human Reprod. Update. 6:107–21. doi:10.1093/humupd/6.2.107
- Camilleri, R., and A.J. Vella. 2010. Effect of fireworks on ambient air quality in Malta. Atmos. Environ. 44:4521–27. doi:10.1016/j.atmosenv.2010.07.057
- Carranza, J.E., B.T. Fisher, G.D. Yoder, and D.W. Hahn. 2001. On-line analysis of ambient air aerosols using laser-induced breakdown spectroscopy. Spectrochim. Acta Part B Atom. Spectrosc. 56:851–64. doi:10.1016/S0584-8547(01)00183-5
- Chang, S.C., T.H. Lin, C.Y. Young, and C.T. Lee. 2011. The impact of ground-level fireworks (13 km long) display on the air quality during the traditional Yanshui Lantern Festival in Taiwan. Environ. Monit. Assess. 172:463–79. doi:10.1007/s10661-010-1347-1
- Chatterjee, A., C. Sarkar, A. Adak, U. Mukherjee, S.K. Ghosh, and S. Raha. 2013. Ambient air quality during Diwali festival over Kolkata- a mega-city in India. Aerosol Air. Qual. Res. 13:1133–44. doi:10.4209/aaqr.2012.03.0062
- Cheng, Y., G. Engling, K. He, F. Duan, Z. Du, Y. Ma, L. Liang, Z. Lu, J. Liu, M. Zheng, and R.J. Weber. 2014. The characteristics of Beijing aerosol during two distinct episodes: Impacts of biomass burning and fireworks. Environ. Pollut. 185:149–57. doi:10.1016/j.envpol.2013.10.037
- Crespo, J., E. Yubero, J.F. Nicolás, F. Lucarelli, S. Nava, M. Chiari, and G. Calzolai. 2012. High-time resolution and size-segregated elemental composition in high-intensity pyrotechnic exposures. J. Hazard. Mater. 241– 42:82–91. doi:10.1016/j.jhazmat.2012.09.017
- Croteau, G., R. Dills, M. Beaudreau, and M. Davis. 2010. Emission factors and exposures from ground-level pyrotechnics. Atmos. Environ. 44(27):3295–303. doi:10.1016/j.atmosenv.2010.05.048
- Deka, P., and R.R. Hoque. 2014. Incremental effect of festive biomass burning on wintertime PM10 in Brahmaputra Valley of northeast India. Atmos. Res. 143:380–91. doi:10.1016/j.atmosres.2014.03.003
- Do, T.M., C.F. Wang, Y.K. Hsieh, and H.F. Hsieh. 2012. Metals present in ambient air before and after a firework festival in Yanshui, Tainan, Taiwan. Aerosol. Air. Qual. Res. 12:981–93. doi:10.4209/aaqr.2012.03.0069
- Dockery, D., C.I. Pope, X. Xiping, J. Spengler, J. Ware, M. Fay, B. Ferris, and F. Speizer. 1993. An association between air pollution and mortality in six US cities. N. Engl. J. Med. 329(24):1753–59. doi:10.1056/NEJM199312093292401
- Dougle, P.G., A.L. Vlasenko, J.P. Veefkind, and B.H.M. Ten. 1996. Humidity dependence of the light scattering by mixtures of ammonium nitrate, ammonium sulfate and soot. J. Aerosol Sci. 27:S513–14. doi:10.1016/0021-8502(96)00329-1
- Dutcher, D.D., K.D. Perry, T.A. Cahill, and S.A. Copeland. 1999. Effects of indoor pyrotechnic displays on the air quality in the Houston Astrodome. J. Air Pollut. Control Assoc. 49:156–60. doi:10.1080/10473289.1999.10463790
- Drewnick, F., S.S. Hings, J. Curtius, G. Eerdekens, and J. Williams. 2006. Measurement of fine particulate and gas-phase species during the New Year’s fireworks 2005 in Mainz, Germany. Atmos. Environ. 40:4316–27. doi:10.1016/j.atmosenv.2006.03.040
- Feng, J.L., P. Sun, X.L. Hu, W. Zhao, M.H. Wu, and J.M. Fu. 2012. The chemical composition and sources of PM2.5 during the 2009 Chinese New Year’s holiday in Shanghai. Atmos. Res. 118:435–44. doi:10.1016/j.atmosres.2012.08.012
- Godri, K.J., D.C. Green, G.W. Fuller, M.D. OSTO, D.C. Beddows, F.J. Kelly, R.M. Harrison, and I.S. Mudway. 2010. Particulate oxidative burden associated with firework activity. Environ. Sci. Technol. 44:8295–301. doi:10.1021/es1016284
- Health & Safety Executive, 2011. Explosives incidents database advisory service. http://www.hse.gov.uk/explosives/eidas.htm (accessed January 19, 2016)
- Hirai, K., Y. Yamazaki, K. Okada, S. Furuta, and K. Kubo. 2000. Acute eosinophilic pneumonia associated with smoke from fireworks. Intern. Med. 39:401–403. doi:10.2169/internalmedicine.39.401
- Hu, H. 2002. Human health and heavy metals exposure. In: Life Support: The Environment and Human Health, ed. M. McCally, chap. 4. Cambridge, MA: MIT Press.
- Huang, K., G. Zhuang, Y. Lin, Q. Wang, J.S. Fu, R. Zhang, J. Li, C. Deng, and Q. Fu. 2012. Impact of anthropogenic emission on air quality over a megacity—Revealed from an intensive atmospheric campaign during the Chinese Spring Festival. Atmos. Chem. Phys. 12:11631–45. doi:10.5194/acp-12-11631-2012
- Jiang, Q., Y.L. Sun, Z. Wang, and Y. Yin. 2014. Aerosol composition and sources during the Chinese Spring Festival: fireworks, secondary aerosol, and holiday effects. Atmos. Chem. Phys. Discuss. 14:20617–46. doi:10.5194/acpd-14-20617-2014
- Joly, A., A. Smargiassi, T. Kosatsky, M. Fournier, E. Dabek-Zlotorzynska, V. Celo, D. Mathieu, R. Servranckx, R. D’Amours, A. Malo, and J. Brook. 2010. Characterization of particulate exposure during fireworks displays. Atmos. Environ. 44: 4325–29.doi10.1016/j.atmosenv.2009.12.010
- Kannan, G.K., M. Gupta, and J.C. Kapoor. 2004. Estimation of gaseous products and particulate matter emission from garden biomass combustion in a simulation fire test chamber. Atmos. Environ. 38:6701–10. doi:10.1016/j.atmosenv.2004.09.041
- Khaparde, V.V., P.P. Pipalatkar, T. Pustode, C.V.C. Rao, and D.G. Gajghate. 2012. Influence of burning of fireworks on particle size distribution of PM10 and associated barium at Nagpur. Environ. Monit. Assess. 184:903–11. doi:10.1007/s10661-011-2008-8
- Kong, S.F., L. Li, X.X. Li, Y. Yin, K. Chen, D.T. Liu, L. Yuan, Y.J. Zhang, Y.P. Shan, and Y.Q. Ji. 2015. The impacts of firework burning at the Chinese Spring Festival on air quality and human health: Insights of tracers, source evolution and aging processes. Atmos. Chem. Phys. 15:2167–84. doi:10.5194/acp-15-2167-2015
- Kosaka, K., M. Asami, Y. Matsuoka, M. Kamoshita, and S. Kunikane. 2007. Occurrence of perchlorate in drinking water sources of metropolitan area in Japan. Water. Res. 41:3474–82. doi:10.1016/j.watres.2007.05.011
- Kulshrestha, U.C., T.N. Rao, S. Azhaguvel, and M.J. Kulshrestha. 2004. Emissions and accumulation of metals in the atmosphere due to crackers and sparkles during Diwali festival in India. Atmos. Environ. 38:4421–25. doi:10.1016/j.atmosenv.2004.05.044
- Lee, C.G., C.S. Yuan, J.C. Chang, and C. Yuan. 2005. Effects of aerosol species on atmospheric visibility in Kaohsiung city, Taiwan. J. Air. Pollut. Control. Assoc. 55:1031–41. doi:10.1080/10473289.2005.10464683
- Li, W.J., Z.B. Shi, C. Yan, L.X. Yang, C. Dong, and W.X. Wang. 2013. Individual metal-bearing particles in a regional haze caused by firecracker and firework emissions. Sci. Total Environ. 443:464–69. doi:10.1016/j.scitotenv.2012.10.109
- Li, X.R., X.Q. Guo, X.R. Liu, C.S. Liu, S.S. Zhang, and Y.S. Wang. 2009. Distribution and sources of solvent extractable organic compounds in PM2.5 during 2007 Chinese Spring Festival in Beijing. J. Environ. Sci. 21(2):142–49. doi:10.1016/S1001-0742(08)62242-1
- Lin, C.C., K.L. Huang, H.L. Chen, J.H. Tsai, Y.P. Chiu, J.T. Lee, and S.J. Chen. 2014. Influences of beehive firework displays on ambient fine particles during the Lantern Festival in the YanShuei area of southern Taiwan. Aerosol. Air. Qual. Res. 14:1998–2009. doi:10.4209/aaqr.2014.09.0201
- Manalis, N., G. Grivas, V. Protonotarios, A. Moutsatsou, C. Samara, and A. Chaloulakou. 2005. Toxic metal content of particulate matter (PM10), within the greater area of Athens. Chemosphere 60(4):557–66. doi:10.1016/j.chemosphere.2005.01.003
- Majumdar, D., and P. Nema. 2011. Assessment of fine particle number profile in fugitive emissions from firecrackers. J. Sci. Ind. Res. 70:225–29.
- Mandal, R., B.K. Sen, and S. Sen. 1997. Impact of fireworks on our environment. Indian J. Environ. Protect. 17:850–53.
- Moreno, T., X. Querol, A. Alastuey, M.C. Minguillòn, J. Pey, S. Rodriguez, J.V. Mirò, C. Felis, and W. Gibbons. 2007. Recreational atmospheric pollution episodes: Inhalable metalliferous particles from firework displays. Atmos. Environ. 41:913–22. doi:10.1016/j.atmosenv.2006.09.019
- Munster, J., G. Hanson, and W. Jackson. 2009. The fallout from fireworks: Perchlorate in total deposition. Water. Air. Soil. Pollut. 198:149–53. doi:10.1007/s11270-008-9833-6
- Nirmalkar, J., M.K. Deb, D.K. Deshmukh, and S.K. Verma. 2013. Mass loading of size-segregated atmospheric aerosols in the ambient air during fireworks episodes in eastern central India. Bull. Environ. Contam. Toxicol. 90:434–39. doi:10.1007/s00128-012-0938-7
- Nishanth, T., K.M. Praseed, K. Rathnakaran, S.M.K. Kumar, R.R. Krishna, and K.T. Valsaraj. 2012. Atmospheric pollution in a semi-urban, coastal region in India following festival seasons. Atmos. Environ. 47:295–306. doi:10.1016/j.atmosenv.2011.10.062
- Pachauri, T., V. Singla, A. Satsangi, A. Lakhani, and K.M. Kumari. 2013. Characterization of major pollution events (dust, haze, and two festival events) at Agra, India. Environ. Sci. Pollut. Res. 20:5737–52. doi:10.1007/s11356-013-1584-2
- Pathak, B., J. Biswas, C. Bharali, and P.K. Bhuyan. 2015. Short term introduction of pollutants into the atmosphere at a location in the Brahmaputra Basin: A case study. Atmos. Pollut. Res. 6:220–29. doi:10.5094/APR.2015.026
- Perrino, C., S. Tiwari, M. Catrambone, S.D. Torre, E. Rantica, and S. Canepari. 2011. Chemical characterization of atmospheric PM in Delhi, India, during different periods of the year including Diwali festival. Atmos. Pollut. Res. 2:418–27. doi:10.5094/APR.2011.048
- Perry, K.D. 1999. Effects of outdoor pyrotechnic displays on the regional air quality of Western Washington State. J. Air Waste Manage. Assoc. 49:146–55. doi:10.1080/10473289.1999.10463791
- Pope, C.A., M.J. Thyb, and M.M. Namboodiri. 1995. Particulate air pollution as a predictor of mortality in a prospective study of US adults. Am. J. Respir. Crit. Care Med. 151:669–74. doi:10.1164/ajrccm.151.3.7881654
- Rao, P.S., D.G. Gajghate, A.G. Gavane, P. Suryawanshi, C. Chauhan, S. Mishra, N. Gupta, C.V.C. Rao, and S.R. Wate. 2012. Air quality status during Diwali Festival of India: A case study. Bull. Environ. Contam. Toxicol. 89:376–79. doi:10.1007/s00128-012-0669-9
- Ravindra, K., S. Mor, and C.P. Kaushik. 2003. Short-term variation in air quality associated with fireworks events: A case study. J. Environ. Monit. 5:260–64. doi:10.1039/b211943a
- Santos-Burgoa, C., C. Rios, L.A. Nercadi, R. Arecguga-Serrano, F. Cano-Vall, R.A. Eden-Wynter, J.L. Texcalac-Sangrador, J.P. Villa-Barragan, Y. Rodriguez-Agudelo, and S. Montes. 2001. Exposure to manganese; Health effects on the general population, a pilot study in central Mexico. Environ. Res. A 85:90–104. doi:10.1006/enrs.2000.4108
- Sarkar, S., P.S. Khillare, D.S. Jyethi, A. Hasan, and M. Parween. 2010. Chemical speciation of respirable suspended particulate matter during a major firework festival in India. J. Hazard. Mater. 184:321–30. doi:10.1016/j.jhazmat.2010.08.039
- Schwartz, J., D.W. Dockery, and L.M. Neas. 1996. Is daily mortality associated specifically with fine particles? J. Air. Pollut. Assoc. 46:927–39. doi:10.1080/10473289.1996.10467528
- Shen, Z.X., J.J. Cao, R. Arimoto, Z.W. Han, R.J. Zhang, Y.M. Han, S.X. Liu, T. Okuda, S. Nakao, and S. Tanaka. 2009. Ionic composition of TSP and PM2.5 during dust storms and air pollution episodes at Xi’an, China. Atmos. Environ. 43:2911–18. doi:10.1016/j.atmosenv.2009.03.005
- Shi, G.L., G.R. Liu, Y.Z. Tian, X.Y. Zhou, X. Peng, and Y.C. Feng. 2014. Chemical characteristic and toxicity assessment of particle associated PAHs for the short-term anthropogenic activity event: During the Chinese New Year’s Festival in 2013. Sci. Total. Environ. 482– 83:8–14. doi:10.1016/j.scitotenv.2014.02.107
- Shimizu, T. 2004. Chemical composition of fireworks compositions. In Pyrotechnic Chemistry, Pyrotechnic Reference Series No. 4, ed. K. Kosanke et al. J. Pyrotechnics (Whitewater, CO).
- Sijimol, M.R., and M. Mohan. 2014. Environmental impacts of perchlorate with special reference to fireworks—A review. Environ. Monit. Assess. 186:7203–10. doi:10.1007/s10661-014-3921-4
- Singh, D.P., R. Gadi, T.K. Mandal, C.K. Dixit, K. Singh, T. Saud, N. Singh, and P.K. Gupta. 2010. Study of temporal variation in ambient air quality during Diwali festival in India. Environ. Monit. Assess. 169:1–13. doi:10.1007/s10661-009-1145-9
- Smith, M.R., and V.D. Dinh. 1975. Changes in forced expiratory flow due to air pollution from fireworks. Preliminary report. Environ. Res. 9:321–31. doi:10.1016/0013-9351(75)90012-2
- Steinhauser, G., and T.M. Klapotke. 2008. “Green” pyrotechnics: A chemists’ challenge. Angew. Chem. Int. Ed. Engl. 47:3330–47. doi:10.1002/(ISSN)1521-3773
- Tandon, A., S. Yadav, and A.K. Attri. 2008. City-wide sweeping a source for respirable particulate matter in the atmosphere. Atmos. Environ. 42:1064–69. doi:10.1016/j.atmosenv.2007.12.006
- Tang, I.N., K.H. Fung, D.G. Imre, and H.R. Munkelwitz. 1995. Phase transformation and metastability of hygroscopic microparticles aerosol. Aerosol Sci. Technol. 23:443–53. doi:10.1080/02786829508965327
- Thakur, B., S. Chakraborty, A. Debsarkar, S. Chakraborty, and R.C. Srivastava. 2010. Air pollution from fireworks during festival of lights (Deepawali) in Howrah, India—A case study. Atmosfera 23:347–65.
- Tian, Y.Z., J. Wang, X. Peng, G.L. Shi, and Y.C. Feng. 2014. Estimation of the direct and indirect impacts of fireworks on the physicochemical characteristics of atmospheric PM10 and PM2.5, Atmos. Chem. Phys. 14:9469–79. doi:10.5194/acp-14-9469-2014
- Tiwari, S., D.M. Chate, M.K. Srivastava, P.D. Safai, A.K. Srivastava, D.S. Bisht, and B. Padmanabhamurty. 2012. Statistical evaluation of PM10 and distribution of PM1, PM2.5, and PM10 in ambient air due to extreme fireworks episodes (Deepawali festivals) in megacity Delhi. Nat. Hazards 61:521–31. doi:10.1007/s11069-011-9931-4
- Tsai, H.H., L.H. Chien, C.S. Yuan, Y.C. Lin, Y.H. Jen, and I.R. Ie. 2012a. Influences of fireworks on chemical characteristics of atmospheric fine and coarse particles during Taiwan’s Lantern Festival. Atmos. Environ. 62:256–64. doi:10.1016/j.atmosenv.2012.08.012
- Tsai, J.H., J.H. Lin, Y.C. Yao, and H.L. Chiang. 2012b. Size distribution and water soluble ions of ambient particulate matter on episode and non-episode days in southern Taiwan. Aerosol Air. Qual. Res. 12:263–74. doi:10.4209/aaqr.2011.10.0167
- Vecchi, R., V. Bernardoni, D. Cricchio, A. D’Alessandro, P. Fermo, F. Lucarelli, S. Nava, A. Piazzalunga, and G. Valli. 2008. The impact of fireworks on airborne particles. Atmos. Environ. 42:1121–32. doi:10.1016/j.atmosenv.2007.10.047
- Verma, C., and D.K. Deshmukh. 2014. The ambient air and noise quality in India during Diwali festival: A review. Recent Res. Sci. Technol. 6(1):203–10.
- Wang, Y., G. Zhuang, C. Xua, and Z. An. 2007. The air pollution caused by the burning of fireworks during the lantern festival in Beijing. Atmos. Environ. 41:417–31. doi:10.1016/j.atmosenv.2006.07.043
- Walsh, K.J., M. Milligan, and J. Sherwell. 2009. Synoptic evaluation of regional PM2.5 concentrations. Atmos. Environ. 43:594–603. doi:10.1016/j.atmosenv.2008.10.019
- World Health Organization. 2006. WHO air quality guidelines for particulate matter, ozone, nitrogen dioxide and sulfur dioxide. Geneva, Switzerland: WHO.
- Wilkin, R.T., D.D. Fine, and N.G. Burnett. 2007. Perchlorate behavior in a municipal lake following fireworks displays. Environ. Sci. Technol. 41:3966–71. doi:10.1021/es0700698
- Yang, L.X., X.M. Gao, X.F. Wang, W. Nie, J. Wang, R. Gao, P.J. Xu, Y.P. Shou, Q.Z. Zhang, and W.X. Wang. 2014. Impacts of firecracker burning on aerosol chemical characteristics and human health risk levels during the Chinese New Year Celebration in Jinan, China. Sci. Total Environ. 476– 77:57–64. doi:10.1016/j.scitotenv.2013.12.110
- Yuan, C.S., C.G. Lee, S.H. Liu, J.C. Chang, C. Yuan, and H.Y. Yang. 2006. Correlation of atmospheric visibility with chemical composition of Kaohsiung aerosols. Atmos. Res. 82:663–79. doi:10.1016/j.atmosres.2006.02.027
- Zhang, M., X. Wang, J. Chen, T. Cheng, T. Wang, X. Yang, and Y. Gong. 2010. Physical characterization of aerosol particles during the Chinese New Year’s firework events. Atmos. Environ. 44:5191–98. doi:10.1016/j.atmosenv.2010.08.048
- Zhao, P., K.P. Yu, and C.C. Lin. 2011. Risk assessment of inhalation exposure to polycyclic aromatic hydrocarbons in Taiwanese workers at night markets. Int. Arch. Occup. Environ. Health 84(3):231–37. doi:10.1007/s00420-010-0551-1