ABSTRACT
This study compares the performances of fern and plastic chips as packing media for the biofiltration of a styrene-laden waste gas stream emitted in a plant for the manufacture of plastic door plates. Fern chips (with a specific surface area of 1090 m2 m−3) and plastic chips (with a specific surface area of 610 m2 m−3) were packed into a pilot-scale biotrickling filter with a total medium volume of 50 L for the performance test. Field waste gas with styrene concentrations in the range of 161–2390 mg Am−3 at 28–30 °C) was introduced to the bed and a fixed empty-bed retention time (EBRT) of 21 sec, a volumetric gas flow rate of 8.57 m3 hr−1, and superficial gas velocity of 53.6 m hr−1 were maintained throughout the experimental period. Nutrients containing metal salts, nitrogen, phosphorus, and milk were supplemented to the filters for maintaining the microbial activities. Results reveal that the biotrickling filter developed in this study had the highest styrene monomer (SM) elimination capacities (170 g m−3 hr−1 for fern-chip packing and 300 g m−3 hr−1 for plastic-chip packing) among those cited in the literature. The plastic medium is a favorable substitute for endangered fern chips. The thermal-setting nature of plastic chips limits their recycle and reuse as raw materials, and the study provides an opportunity for the utilization of the materials.
Implications: Biotreatment of contaminants in air streams offers an inexpensive and efficient alternative to conventional technologies. Biofiltration has a great potential for the degradation of gas-borne styrene and total hydrocarbon (THC) removal efficiency of around 80%. The objective of this research was to compare the performances of fern chips and a kind of plastic chips as packing media for biofiltration of the styrene-laden waste gas stream emitted from cutting operations of stripes of premixed unsaturated polyester (UP) and styrene paste before hot-pressing operations for making plastic door plates. From a practical point of view, the plastic medium can be a good substitute medium for fern chips, which has been declared as a protected plant. This study provides an experimentally verified model for the design and operation of such biotreatment systems.
Introduction
Various unsaturated polyester (UP) resins are manufactured using maleic acid, phthalic acid, and 2–6-carbon glycols (ethylene, propylene, diethylene, and dipropylene glycols) as raw materials. The UP resin thus produced is then blended with styrene monomer (SM) as a solvent and as a cross-linking agent to form a paste. The paste is then coated on woven fiber-glass mats or blended with glass fibers and heated to form thermosetting fiber glass–reinforced plastic (FRP) materials or products. Collection systems should be provided to vent the SM-containing gas that is emitted during the cutting and curing operations and end-of-the-pipe treatment systems be used to eliminate SM in the vented gas. A plant located in southern Taiwan produces FRP door plates by using cut stripes of premixed UP and SM before hot-pressing operations. Waste gas that contains SM, emitted from the stripe-cutting operation, should be treated to prevent air pollution.
The biofiltration or biotrickling treatment of waste gases that contain SM at a concentration of <1000 ppm (around 4200 mg/m3 at 25 °C and 1 atm) has been shown to be both technically and economically feasible. Many papers concerning the biotreatment of SM have been published, but in most, compost, peat, perlite (an amorphous expanded volcanic glass with a typical bulk density of about 30–150 kg m−3), porous ceramic, and biomass pellets are used as packing media for nutrient storage or biofilm attachment. Williams and Miller (Citation1992) and Lee, Lau, and Pinder (Citation2001) showed that for effective biofiltration performance, the medium must provide an excellent environment for bacterial growth while maintaining large reactive surface, high absorption capacity, high pH buffering capacity, a low pressure drop, a good pore structure, and a very low compaction over time. The selection of media should therefore take into account all of these parameters, but such high-performing media are not easily prepared. The development of media that have a simple composition, are economical and effective, and have long-term effectiveness is important.
Fern chips have been tested and found to be effective as a single packing medium for the biotrickling filtration of ammonia, PGMEA (propylene glycol methyl ether acetate), MEK (methyl ethyl ketone), odorous fumes emitted from recycled nylon-melting operation, and mixed volatile organic compounds (VOCs) emitted from semiconductor industries. Experiences show that these chips do not rot for 3–5 yr under either moist or dry conditions. Fern chips are used because of their simple composition containing only one material, lower pressure drop of gas that flows through them, minimal tendency to clog and become compact, and ease of use in controlling moisture, nutrient, pH, and metabolites (Chen, Chang, and Chou Citation2013; Chou, Chang, and Perng Citation2008; Chou and Li Citation2009, Citation2010; Chou and Wang Citation2007). However, this material has not yet been tested for use in the biofiltration of styrene-laden air. Although fern chips exhibit good performance as a packing material for removing VOCs, their supply has become limited in recent years because some countries limit their harvest and export. An alternative material with similar performance should be developed and tested. Plastic chips that are obtained from the drilling and shaving operations that are involved in the finishing of FRP door plates have been considered as such.
This study compares the performances of fern chips and plastic chips as packing media in the biofiltration of the SM-laden waste gas stream that is emitted from stripe-cutting operations.
Materials and methods
Experimental setup
schematically depicts the experimental setup. A pilot-scale six-tray biotrickling filter (in which each tray is 40 cm [width] × 40 cm [depth] × 8 cm [height], packed with 5.2 cm height medium to yield a medium volume of 8.33 L) with a total medium volume of 50 L was constructed for use in the performance test. The waste gas from the plant was induced by a blower, and nutrient solution was sprayed on the surface of the medium in the top compartment. Gas inlet and exit ports of the reactor were used for gas sampling for SM analysis.
Materials
Filters of two kinds were compared in terms of performance in SM removal. The medium in the first filter was a blend of fern chips, and its characteristics are provided in (Chou and Li Citation2009). It was imported from Indonesia and obtained from a local shop. shows the filter and the fern chips. The second medium was a blend of equal volumes of plastic chips and turnings that were wastes from the processing of FRP door plates. presents the characteristics of the chips and turnings, and shows their appearance.
Table 1. Characteristics of the fern chips and their packed bed (Chou and Li Citation2009).
Table 2. Characteristics of the plastic chips and turnings, and the mixed medium.
For obtaining physical properties of the bed packed with cylinder-type plastic chips, the following measurements were made. Packing density of the plastic chips was obtained by measuring the mass of 1 L of the packed chips in a 1.5-L beaker. Water replacement was then used to measure the void volume of the 1 L of chips. Chip diameters were obtained by measuring 10 representative chips by using a screw-type micrometer, and lengths of the 10 chips were also obtained by using a common straight edge. From the obtained data, a = total chip surface area/(total packing volume) for the bed packed with cylinder-type chips was calculated by the following equation:
where V is the total packing volume (m3), set as 4 in this study; ε is the void fraction of the packed space (dimensionless); x is the average length of the 10 chips (m); and d is the average diameter of the 10 chips (m). a = 4 (1 − ε)/d = 420 m2 m−3. The term “V(1 − ε)/(xπd2/4)” accounts for the total chip numbers in the volume V and “xπd” for the surface area of one chip with x >> d.
Similar methods were used for obtaining a for the turnings. The equation is as follows:
where w is the average width of the 10 turnings (m), and t is the average thickness of the 10 turnings (m). a = 2 (1 − ε)/t = 800 m2 m−3. The term “V(1 − ε)/(xwt)” accounts for the total turning numbers in the volume V and “2xw” for the surface area of one turning with x and w >> t.
Operation
Before packing, both media were immersed for 2 days in the mixed liquor of an activated sludge pond used for the treatment of wastewater effluent from an ABS (acrylonitrile-butadiene-styrene) resin plant in the same industrial park as the FRP-door manufacturing plant. According to Bina et al. (Citation2004), Dion St-Pierre, Ramirez, and Heitz (Citation2009), Novak et al. (Citation2008), Chou, Chang, and Perng (Citation2008), Chou and Li (Citation2009, Citation2010), Chen et al. (Citation2013), and Delmoménie et al. (Citation2001), the activated sludge is rich in microorganisms that degrade SM. The bed was first packed with the fern chips and operated for 93 days, followed by packing with the mixed plastic chips and operated for the following 60 days.
shows nutrition rates of both media, taken from related studies. Milk was added to the media as a source of natural nutrients throughout the experimental period. Milk contains nitrogen (N), phosphorus (P), and many other essential natural nutrients for microorganisms. The milk addition yields much higher VOC removal in the treatment by fern-chip biofilters, a mixture of gas-borne PGMEA (propylene glycol monomethyl ether acetate), MEK (methyl ethyl ketone), and VOCs (volatile organic compounds) that are emitted from recycled nylon-melting operations than does the addition of inorganic N and P (Chou, Chang, and Perng Citation2008; Chou and Li Citation2009, Citation2010; Chen, Chang, and Chou Citation2013). All inorganic nutrients are reagent grades. Milk was obtained from a local supermarket, and the high-fructose corn syrup is a product of Fonenfonher Enterprise Co., Tainan, Taiwan.
Table 3. Nutrition rates.
A fixed empty-bed retention time (EBRT) of 21 sec, a volumetric gas flow rate of 8.57 m3 hr−1, and superficial gas velocity of 53.6 m hr−1 for the influent gas that flows through the empty bed space were maintained throughout the experimental period. The SM concentration in the influent gas varied with the varying field operational conditions. Gas samples were taken from the influent and effluent points of the reactor and measured for their total hydrocarbon (THC) contents for 5–30 min each time every 2–4 days. Average influent and effluent THC concentrations were then obtained to give a data set for evaluation of the bed performance for SM removal.
During the operation, nutrient solution was sprayed at a rate of 2 L min−1 for 15 min each time every 2 hr. According to Ottengraf (Citation1986), a biofiltration system consists mainly of a reactor packed with solid materials on which a biofilm with a proper microbial population is formed. The solid materials consist of at least a base organic medium (e.g., peat and compost) to support microbial growth and an amendment (e.g., perlite and bark) to provide structural strength for the bed or to reduce the operational pressure drop across the packed bed. To keep the packed solid materials from being dried during the operation, humidified gas or sprinkling water is usually applied to the solids. In the present study, inert fern or plastic chips were used as the medium and the required nutrients and moisture were supplied by the sprinkled nutrient solution. It had been tested and shown that the nutrient solution spraying mode could keep the medium in a moist condition suitable for the styrene degradation and keep the biomass from being flushed away from the medium surface. Strictly, the operation was like a biotrickling biofilter. No biomass was observed in the nutrient circulation tank, and no apparent biodegradation of styrene occurred in the tank.
Analytical
Gas samples with known styrene contents were used for preparing the calibration curve. THC concentrations in the influent and effluent gas samples were measured at the same time by two on-line flame ionization detectors (toxic vapor analyzer, TVA-1000B; Thermo Environmental Instruments Inc., xx, xx, USA). Correlation curves were also made to obtain SM concentration data from the measured THC data. SM concentrations in gas samples were measured using a gas chromatograph (GC-14B; Shimadzu Co., xx, Japan) with a capillary column (with an inner diameter [i.d.] of 0.53 mm and a length of 30 m, coated with 5-mm-thick polydimethylsiloxane) (Alltech Co., no. 16843; xx, xx) and a flame ionization detector (FID). The detection limit was 1 mg m−3 for an injected gas sample of 1 mL. Temperatures of 150 and 100 °C, respectively, were kept for the injection well and the column oven of the gas chromatograph. Nitrogen gas with a purity of 99.9% was used at a constant flow rate of 20 mL min−1 to carry the injected sample gas. pH of the nutrient solution was measured by a pH meter (model 761; Knick Co., xx, Italy).
Results and discussion
Operation data
shows two typical sets of the THC data of the measured THC for the influent and effluent gases to and from the fern-chip bed. Average influent and effluent THC concentrations were then obtained to give a data set for the bed performance evaluation. shows two typical sets of the THC data for the bed packed with the mixed plastic medium for the bed performance evaluation. As can be seen from the data, THC in the influent gases varied greatly with time due to the intermittent cutting operation of the UP strips containing SM monomers. Before the measurements, pHs of the nutrient solution were in the range of 6.2–6.5 and the pHs were adjusted to the range of 7.0–7.6.
Performance of fern-chip medium in removing SM
To evaluate the performances of the biofilter media, the volumetric loading L (g m−3 hr−1) and elimination capacity K (g m−3 hr−1) of the medium for a specific pollutant are defined as
where Q is the influent gas flow rate to the biofilter (m3 hr−1); Ci and Co represent the target pollutant concentrations (g m−3) in the influent and effluent gas streams, respectively; V is the total volume of the medium (m3), and η = (Ci − Co)/Ci is the total removal efficacy of the influent target pollutant(s).
plots time variations of SM concentrations in the influent gas to and effluent gas from the fern-chip medium, and variation of the volumetric SM elimination capacity (K) with its loading (L) to the fern-chip medium. SM concentrations of the influent gas were in the range of 161–1190 mg Am−3 (average ± standard deviation = 486 ± 280 mg Am−3 at 28–30 °C), and those of the effluent gas were 5.7–338 mg Am−3 (average ± standard deviation = 67 ± 73 mg Am−3 at 30–32 °C). The large SM concentration fluctuations of the influent gas arose from the intermittent cutting operation of the premixed UP-SM strips before the hot-pressing operation. The effluent gas temperature (30–32 °C) exceeded slightly that of the influent gas (28–30 °C) because some energy generated by the ring blower was converted into heat and delivered into the gas.
Figure 6. (a) Time variations of SM concentrations in the gases influent to and effluent from the fern-chip medium. (b) Variation of the volumetric SM elimination capacity (K) with its loading (L) to the fern-chip medium. K/L = 0.86 indicates an average SM removal efficiency of 86% with L < 200 g m−3 hr−1.
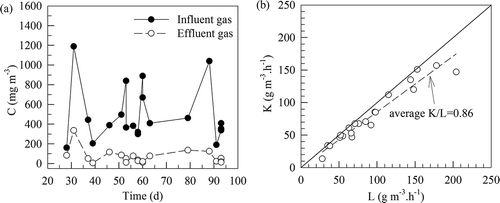
plots the variation of the volumetric SM elimination capacity (K) with its loading (L) to the fern-chip medium. K/L = 0.86 indicates an average SM removal efficacy of 86% with L < 200 g m−3 hr−1. On an average, the fern-chip had an elimination capacity of K = 170 g m−3 hr−1.
Performance of plastic medium in removing SM
plots the variations over time of SM concentrations in the influent gas to and the effluent gas from the mixed plastic medium. The influent SM concentration was in the range of 361–2390 mg Am−3 (average ± standard deviation = 1140 ± 652 mg Am−3 at 28–30 °C), and the effluent SM concentration was in the range of 6.4–620 mg Am−3 (average ± standard deviation = 180 ± 171 mg Am−3 at 30–32 °C). Large fluctuations of the influent SM concentration were also observed owing to the intermittent strip-cutting operation. plots variation of K with L to the plastic medium; the average K/L of 0.85 indicates an average SM removal efficacy of 85% with L < 350 g m−3 hr−1. On an average, the plastic medium had an elimination capacity of K = 300 g m−3 hr−1.
Figure 7. (a) Time variations of SM concentrations in the gases influent to and effluent from the plastic medium. (b) Variation of the volumetric SM elimination capacity (K) with its loading (L) to the plastic medium. K/L = 0.85 indicates an average SM removal efficiency of 86% with L < 350 g m−3 hr−1.
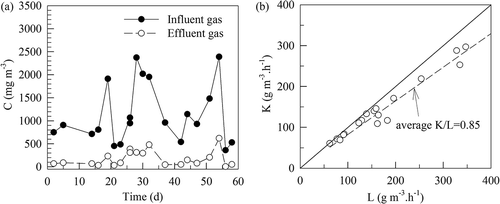
Comparisons of media in removing SM
compares laboratory biofilters and biotrickling filters, including the ones herein, in terms of their effectiveness in removing SM.
Table 4. Removal of SM in laboratory biotrickling filters or biofilters.
According to the data listed in , it can be seen that perlite (an amorphous volcanic glass, usually thermal-expanded to give a bulk density of about 30–150 kg m−3) had a relatively higher K of 62–145 g m−3 hr−1 (Cox, Doddema, and Harder Citation1993; Cox et al. Citation1997; Paca et al. Citation2001; Weigner et al. Citation2001) among the media listed in the table. Possible reasons could be the small particle sizes (<10 mm), hydrophilic surface, and porous structure that led to high biomass densities in the packed space. Celite pellets (size 6 mm) and ceramics had smaller K (56 and 81 g m−3 hr−1) compared with that of perlite, possibly because of no porous structure in these materials (George et al. Citation1998; Jang, Hirai, and Shoda Citation2004). Biofilters packed with compost or peat as a nutrient supplemental material and inert filling materials (plastics, ceramics, and wood bark) exhibited reasonable and practical K in the range of 50–170 g m−3 hr−1 (Bina et al. Citation2004; Jang, Hirai, and Shoda Citation2004, Citation2005; Juneson, Ward, and Signh Citation2001). However, further nutrient supplementation could be difficult upon their depletion.
The biotrickling filter that was developed in this study had the highest SM elimination capacities (maximum K of 170 g m−3 hr for fern-chip packing and 300 g m−3 hr−1 for plastic-chip packing), probably because it had a high specific surface area (fern chips 1090 ± 270 m2 m−3 and plastic chips 610 ± 50 m2 m−3) and because the chip bed supported channel-free flow. In addition to the characteristics, the thermal-setting nature of plastic chips and turnings limits their recycle and reuse as raw materials. The study provides an opportunity for the utilization of the materials.
Conclusion
This study used a pilot-scale biotrickling filter packed with either fern chips or plastic chips to test the removal of SM (styrene) in an air stream. The influent SM concentrations were in the range of 161–2390 mg Am−3 (at 28–30 °C), and EBRT was fixed at 21 sec throughout the experimental period. The following conclusions can be drawn:
The biotrickling filter developed in this study has the highest SM elimination capacities (170 g m−3 hr for fern-chip packing and 300 g m−3 hr−1 for plastic-chip packing) among those cited in the literature.
Nutrients containing metal salts, nitrogen, phosphorus, and milk supplemented to the present filters are suitable for maintaining the microbial activities and sustaining the SM elimination capacities.
The plastic medium is a favorable substitute for endangered fern chips. The thermal-setting nature of plastic chips limits their recycle and reuse as raw materials, and the study provides an opportunity for the utilization of the materials.
Additional information
Notes on contributors
Fu-Jen Chuang
Fu-Jen Chuang is a Ph.D. candidate at the Institute of Environmental Engineering, National Sun Yat-sen University, Kaohsiung, Taiwan, Republic of China.
Ming-Shean Chou
Ming-Shean Chou is a professor at the Institute of Environmental Engineering, National Sun Yat-sen University, Kaohsiung, Taiwan, Republic of China.
Hsiao-Yu Chang
Hsiao-Yu Chang is a postdoctoral fellow at the Institute of Environmental Engineering, National Sun Yat-sen University, Kaohsiung, Taiwan, Republic of China.
References
- Arnold, M., A. Reittu, A. Von Wright, P. J. Martikainen, and M.-L. Suihko. 1997. Bacterial degradation of styrene in waste gases using a peat filter. Applied Microbiology and Biotechnology 48:738–44. doi:10.1007/s002530051126.
- Bina, B., R. Dehghanzadeh, H. Pourmoghadas, A. Kalantary, and A. Torkian. 2004. Removal of styrene from waste gas stream using a biofilter. Journal of Research in Medical Sciences 6:280–88.
- Chen, K. B., H. Y. Chang, and M. S. Chou. 2013. Biofiltrtion of odorous fume emitted from recycled nylon melting operation. Journal Air and Waste Manage Association 63 (11):1–9.
- Chou, M. S., Y. F. Chang, and H. T. Perng. 2008. Treatment of PGMEA in air stream by a biofilter packed with fern chips. Journal Air and Waste Manage Association 58:1590–97. doi:10.3155/1047-3289.58.12.1590.
- Chou, M. S., and C. C. Hsiao. 1998. Treatment of styrene-contaminated airstream by biotrickling filter packed with slags. Journal Environmental Engineering, ASCE 124 (9):844–50. doi:10.1061/(ASCE)0733-9372(1998)124:9(844).
- Chou, M. S., and S. C. Li. 2009. Treatment VOC mixtures in air streams by a biofilter packed with fern chips. Journal Environment Engineering Manage 19 (4):203–11.
- Chou, M. S., and S. C. Li. 2010. Elimination of MEK in air streams by a biofilter packed with fern chips. Environmental Engineering Science 27 (8):679–87. doi:10.1089/ees.2010.0068.
- Chou, M. S., and C. H. Wang. 2007. Elimination of ammonia in air stream by a fern-chip biofilter. Environmental Engineering Science 24 (10):1423–30. doi:10.1089/ees.2007.0006.
- Cox, H. H. J., H. J. Doddema, and W. Harder. 1993. Purification of styrene-containing waste gases in biofilters. Mededelingen, Faculteit Landbouwkundige En Toegepaste Biologische Wetenschappen (Universiteit Gent) 58:1741–48.
- Cox, H. H. J., R. E. Moerman, S. van Baalen, W. N. M. van Heiningen, H. J. Doddema, and W. Harder. 1997. Performance of styrene-degrading biofilter containing the yeast exophiala jeanseilmei. Biotechnology Bioeng 53:259–66. doi:10.1002/(SICI)1097-0290(19970205)53:3<259::AID-BIT3>3.0.CO;2-H.
- Dehghanzadeh, R., A. Torkian, B. Bina, H. Poormoghaddas, and A. Kalantary. 2005. Biodegradation of styrene laden waste gas stream using a compost-based biofilter. Chemsphere 60:434–39. doi:10.1016/j.chemosphere.2004.12.003.
- Delmoménie, M.-C., L. Bibeau, J. Gendron, R. Brzezinski, and M. Heitz. 2001. Toluene removal by biofiltration: Influence of the nitrogen concentration on operational parameters. Industrial `Engineering Chemical Researcher 40:5405–14. doi:10.1021/ie0011270.
- Dion St-Pierre, M.-C., A. A. Ramirez, and M. Heitz. 2009. Biofiltration of air contaminated by styrene vapors on inorganic filtering media: An experimental study. Journal Air & Waste Manage Association 59:568–78. doi:10.3155/1047-3289.59.5.568.
- George, A. S., F. L. Smith, M. T. Suidan, P. Amit, B. Pratim, and C. B. Richard. 1998. Evaluation of trickle-bed air biofilter performance for styrene removal. Water Research 32:1593–603. doi:10.1016/S0043-1354(97)00355-2.
- Jang, J. H., M. Hirai, and M. Shoda. 2004. Styrene degrading by Pseudomonas sp. SR-5 in biofilters with organic and inorganic packing materials. Applications Microbiologic Biotechnology 65:349–55. doi:10.1007/s00253-004-1628-0.
- Jang, J. H., M. Hirai, and M. Shoda. 2005. Performance of a styrene-degrading biofiter innoculated with Pseudomonas sp. SR-5. Journal of Bioscience and Bioengineering 100:297–302. doi:10.1263/jbb.100.297.
- Jang, J. H., M. Hirai, and M. Shoda. 2006. Effect of shutdown on styrene removal in a biofiter innoculated with Pseudomonas sp. SR-5. Journal Hazardous Mat 129:233–227. doi:10.1016/j.jhazmat.2005.08.042.
- Jerio, H., L. Bibeau, and M. Heitz. 2000. Biofiltration of air contaminated by styrene: Effect of nitrogen supply, gas flow rate, and inlet concentration. Environment Sciences Technological 34:1764–71. doi:10.1021/es990911c.
- Juneson, C., O. P. Ward, and A. Signh. 2001. Microbial treatment of a styrene-contaminated air stream in a biofilter with high elimination capacities. Journal of Industrial Microbiology & Biotechnology 26:196–202. doi:10.1038/sj.jim.7000109.
- Lee, D. H., A. K. Lau, and K. L. Pinder. 2001. Development and performance of an alternative biofilter system. Journal Air and Waste Manage Association 51:78–85. doi:10.1080/10473289.2001.10464255.
- Novak, V., J. Paca, M. Halecky, and C. R. Soccol. 2008. Styrene biofiltration in a trickle-bed reactor. Brazilian Achieves of Biology and Technology 51 (2):385–90. doi:10.1590/S1516-89132008000200019.
- Ottengraf, S. P. P. 1986. Exhaust gas purification. In: Biotechnology, eds. H. J. Rehm and G. Reed, Vol. 8, 425–452. Weinheim, Germany: VCH Verlagsgesellsch.
- Paca, J., B. Koutsky, M. Maryska, and M. Halecky. 2001. Styrene degradation along the bed height of perlite biofilter. Journal Chemical Technological Biotechnology 76:873–78. doi:10.1002/(ISSN)1097-4660.
- Weigner, P., J. Paca, P. Loskot, B. Koutsky, and M. Sobotka. 2001. The start-up period of styrene degrading biofilters. Folia Microbiol 46:211–16. doi:10.1007/BF02818535.
- Williams, T. O., and F. C. Miller. 1992. Odor control using biofilters: Part 1. Biocycle (October):73–77.
- Zilli, M., E. Palazzi, L. Sene, A. Converti, and M. Del Borghi. 2001. Toluene and styrene removal from air in biofilters. Process Biochemistry (Barking, London, England) 37:423–29. doi:10.1016/S0032-9592(01)00228-X.