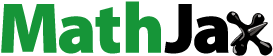
ABSTRACT
To achieve successful composting, all the biological, chemical, and physical characteristics need to be considered. The investigation of our study was based on various physicochemical properties, i.e., temperature, ammonia concentration, carbon dioxide concentration, pH, electrical conductivity (EC), carbon/nitrogen (C/N) ratio, organic matter (OM) content, moisture content, bacterial population, and seed germination index (GI), during the composting of poultry manure and sawdust for different aeration rates and reactor shapes. Three cylindrical-shaped and three rectangular-shaped pilot-scale 60-L composting reactors were used in this study, with aeration rates of 0.3 (low), 0.6 (medium), and 0.9 (high) L min−1 kg−1 DM (dry matter). All parameters were monitored over 21 days of composting. Results showed that the low aeration rate (0.3 L min−1 kg−1 DM) corresponded to a higher and longer thermophilic phase than did the high aeration rate (0.9 L min−1 kg−1 DM). Ammonia and carbon dioxide volatilization were directly related to the temperature profile of the substrate, with significant differences between the low and high aeration rates during weeks 2 and 3 of composting but no significant difference observed during week 1. At the end of our study, the final values of pH, EC, moisture content, C/N ratio, and organic matter in all compost reactors were lower than those at the start. The growth rates of mesophilic and thermophilic bacteria were directly correlated with mesophilic and thermophilic conditions of the compost. The final GI of the cylindrical reactor with an airflow rate of 0.3 L min−1 kg−1 DM was 82.3%, whereas the GIs of the other compost reactors were below 80%. In this study, compost of a cylindrical reactor with a low aeration rate (0.3 L min−1 kg−1 DM) was more stable and mature than the other reactors.
Implications: The poultry industry is growing in South Korea, but there are problems associated with the management of poultry manure, and composting is one solution that could be valuable for crops and forage if managed properly. For high-quality composting, the aeration rate in different reactor shapes must be considered. The objective of this study was to investigate various physicochemical properties with different aeration rates and rector shapes. Results showed that aeration rate of 0.3 L min−1 kg−1 DM in a cylindrical reactor provides better condition for maturation of compost.
Introduction
The poultry industry of South Korea has continued to expand, with significant increases in production. Korea’s chicken production is projected to increase 6.5% to 905,000 metric tons (MT) in 2018 from 850,000 MT in 2017 (United States Department of Agriculture [USDA] Citation2017). As a result, a large amount of manure is produced, which can cause serious environmental pollution. Composting of the manure is a widely used technique for dealing with this problem. Composting has been recognized as a natural process for stabilizing organic wastes. Proper composting effectively reduces odor emission and nitrogen (N) loss, and the organic waste becomes a relatively stable product for use as fertilizer. After composting, handling and transport of waste become easier and safer. To achieve good compost, factors such as the moisture content, carbon/nitrogen (C/N) ratio, aeration rate, temperature, and pH of the compost, as well the selection of the composting materials and the applied methodology, should be appropriately controlled.
In comparison with laboratory- or pilot-scale experimentation, which allows for a high degree of control and replication, full-scale or windrow composting experimentation can be both expensive and difficult to control (Mason and Milke Citation2005). Among the influencing factors, aeration rate and reactor shape are key for sustaining a thermophilic phase. The aeration rate affects microbial activity, degradation rate, and temperature variations during the composing process (Kuter, Hoitink, and Rossman Citation1985). Too much aeration can lead to cooling and the prevention of thermophilic conditions; however, too little aeration can lead to anaerobic conditions (Ahn, Richard, and Choi Citation2007). Several previous studies have investigated aeration methods and rates, with different aeration rates being recommended, e.g., 0.44 L min−1 kg−1 dry matter (DM) in the composting of maize stalks and cow feces (Nada Citation2015), 0.62 L min−1 kg−1 volatile solids (VS) in the composting of vegetable and fruit wastes (Arslan, Ünlü, and Topal Citation2011), 0.5 L min−1 kg−1 organic matter (OM) in the composting of chicken manure and sawdust (Gao et al. Citation2010), 0.25 L min−1 kg−1 OM in the composting of dairy manure with rice straw (Li, Zhang, and Pang Citation2008), 0.43−0.86 L min−1 kg−1 OM in the composting of food waste (Lu et al. Citation2001), 0.04–0.08 L min−1 kg−1 OM for swine manure composting (Lau et al. Citation1992), and 0.87–1.07 L min−1 kg−1 OM in the composting of dairy manure with crop and forest residues (Hong, Matsuda, and Ikeuchi Citation1983). The range of aeration rates varies according to the compost material and composting process.
To ensure that the thermophilic phase can be attained and sustained during composting, reactor shape and volume must be considered during reactor design (Phillip Citation2010). The amount of heat produced in a compost reactor is proportional to the volume (V) of the substrate, and the heat loss is proportional to the surface area (SA) of the reactor. Therefore, the SA/V ratio must be considered when building a compost reactor (Magalhaes et al. Citation1993). The volumes of compost reactors reported in the literature range from 0.4 to 1780 L. Large-volume reactors are able to achieve higher temperatures owing to a lower SA/V ratio (Phillip Citation2010). In previous studies, both cylindrical and rectangular shape reactors have been used to study various composting processes, such as a 30-L self-heating cylindrical reactor with a SA/V ratio of 18 m2 m−3 (VanderGheynst and Lei Citation2003), a 119-L self-heating cylindrical reactor with a SA/V ratio of 12.1 m2 m−3 (Barrington et al. Citation2003), a 33.3-L self-heating cylindrical reactor with a SA/V ratio of 17.6 m2 m−3 (Schloss, Chaves, and Walker Citation2000), a 985-L self-heating rectangular reactor with a SA/V ratio of 6 m2 m−3 (Pecchia, Beyer, and Wuest Citation2002), and a 840-L self-heating rectangular reactor with a SA/V ratio of 6.5 m2 m−3 (Leth, Jensen, and Iversen Citation2001).
For high-quality composting, the aeration rate in different reactor shapes must be considered. The objective of this study was to investigate various physical and physicochemical properties, i.e., temperature, ammonia concentration, carbon dioxide concentration, pH, electrical conductivity (EC), C/N ratio, organic matter content, moisture content, bacterial population, and seed germination index (GI), during the composting of poultry manure and sawdust at different aeration rates in cylindrical and rectangular reactors.
Materials and methods
Composting setup and design
Six pilot-scale composting reactors with two different shapes (three rectangular reactors and three cylindrical reactors) were used in this study. The reactors were made from high-density polyethylene. The cylindrical reactors each had a total volume of 60 L (0.66 m height and 0.34 m diameter) with a SA/V ratio of 14.8 m2 m−3, and the rectangular reactors each had a total volume of 60 L (height: 0.66 m; length: 0.3 m; width: 0.3 m) with a SA/V ratio of 16.3 m2 m−3 (). The reactors were insulated with a layer of polyurethane foam (2.54 cm thickness). A vertical rotating axis with blades ensured complete mixing of the composting mass prior to sampling. The reactors were equipped with a valve for removing the leachate.
Figure 1. Schematic of the cylindrical and rectangular reactors with experimental apparatus. 1, air compressor; 2, solenoid valve; 3, timer; 4, gas washing bottle (NaOH solution); 5, gas washing bottle (distilled water); 6, airflow meter; 7, reactor with insulation; 8, perforated plate; 9, digital thermometer; 10, ammonia sensor; 11, carbon dioxide sensor; 12, leachate outlet.
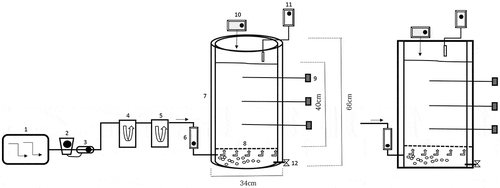
A plastic grid was installed 0.08 m above the bottom of the reactor to support the composting bed and ensure uniform air distribution. The air was input at the bottom using an air compressor (Air Land S45-40–4.5; Seoul, South Korea). Airflow inputs were intermittent, with 45 min of aeration followed by 15 min without aeration, as controlled by a timer and solenoid valve (Auto Sigma HPW2120; Gyeonggi-do, South Korea). The airflow rates in the reactors were set at 0.3, 0.6, and 0.9 L min−1 kg−1 DM. Before the start of the main experiment, we performed two tests in two reactors with aeration rates of 0.2 and 0.6 L min/kg DM for 1 week. The first test was performed with 30 min/hr aeration rate, and the second test was performed with 15 min/hr aeration rate. The temperatures with 0.2 L min−1 kg−1 DM reached maximum of up to 40 and 45 °C in the two tests, respectively. Therefore, aeration rates for the main experiment were selected to be 0.3, 0.6, and 0.9 L min/kg DM, with aeration time of 15 min/hr. An airflow meter (Valve Acrylic Flowmeter, Cole-Parmer) and pressure regulators were used to control the airflow rates. The compost temperature was monitored daily (10 a.m., 2 p.m., and 6 p.m.) at three different locations in the substrate using digital thermometers (Testo 905 T1). The ambient temperature was also recorded daily.
Before entering the reactors, the air was passed over a solution of sodium hydroxide in order to remove traces of carbon dioxide. The air was then moisturized through a gas washing bottle, i.e., a 500-mL bottle of distilled water, prior to entering the reactor, so as to maintain the humidity in the reactor.
Compost materials
Fresh poultry manure and sawdust were used as experimental materials. Poultry manure was collected from poultry farms in Sacheon, South Korea, and sawdust was purchased from a wood chipping mill. The initial moisture content, organic matter content, pH, EC, total organic carbon, total nitrogen (TN), and C/N ratio of the manure and sawdust were analyzed (). The manure and sawdust were mixed using a concrete mixer to achieve effective homogenization of the materials. The initial moisture content and C/N ratio of the homogenized material were adjusted to approximately 65% and 25, respectively, prior to placing the material into the reactors. The initial physicochemical properties of compost mixtures for all reactors are shown in .
Table 1. Initial characteristics of poultry manure, sawdust, and all composts in reactors.
Process monitoring and parameter analysis
For carbon dioxide determination, a Lutron MCH-383SD (Germany; accuracy ±40 ppm) electrochemical sensor was set above the composting material in each reactor. The Lutron MCH-383SD was able to record the date and time interval of the measurements, and it contained a memory card for storing these data. Carbon dioxide was measured at 10-min intervals, and the recorded data were averaged for further analysis and interpretation.
A ZDL800 ammonia meter (Environmental Sensors accuracy ±4 ppm) was used as an electrochemical sensor for ammonia determination above the compost material in each reactor. As the exposure of an electrochemical sensor to high concentrations of ammonia over long periods leads to errors in the readings, the ZDL800 sensor was used for 1-hr measurements (at the same time, daily) and the data were transferred to a computer (Qasim et al. Citation2017). This sensor was able to store the date, time, and the interval between each sampling point and actual exposure point.
After mixing by the bladed vertical rotating axis, the samples of compost material were taken from five different depths of compost and mixed in plastic bags. Approximately 50 g of the material was taken from plastic bags for further analysis. The moisture content of the experimental material was determined by oven drying at 105 °C for 24 hr (American Public Health Association [APHA] Citation1995). The C and N contents were determined using an automated LECO True Mac CNS elemental analyzer. The LECO True Mac CNS has overall measuring range from 20 ppm to 30% with high precision. EC and pH were measured in an aqueous extract, which was obtained by mechanically shaking the samples with distilled water at a solid/water ratio of 1:10 (w/v) for 1 hr. The suspension was centrifuged and filtered through Whatman no. 42 filter paper. The pH and EC measurements were carried out using digital meters (Oakton handheld pH meter, accuracy ±2 mV; CON 450 Eutech conductivity meter, accuracy ±1%). The organic matter content (volatile solids) of the material was measured by burning in an oven at 550 °C for 6 hr (APHA 1995).
The loss of organic matter (k) from the compost material was calculated from the initial and final organic matter contents, according to eq 1 (Diaz et al. Citation2002; Külcu and Yaldiz, Citation2004):
where OMi is the organic matter content at the beginning of the process, and OMf is the organic matter content at the end of the process.
Mesophilic and thermophilic bacterial populations
Colony-forming units (CFU) of mesophilic and thermophilic bacteria were determined by the spread plate technique (Prescot, Harley, and Kleon Citation1996). Nutrient agar was used as a growth medium. One gram of each sample was suspended in 99 mL of physiological solution and then homogenized using a magnetic stirrer for 30 min. Dilution series (from 10−3 to 10−8) were made from the prepared suspensions. One milliliter of each sample from the above-mentioned dilution was placed into a Petri dish and spread up and down using a “hockey stick.” The Petri dishes were incubated at 37 °C for 48 hr for the growth of mesophilic bacteria and at 55 °C for 48 hr for the growth of thermophilic bacteria. The CFU of mesophilic and thermophilic bacteria were counted using a digital colony meter, and the results were expressed as CFU of mesophilic and thermophilic bacteria per gram of compost.
Radish seed germination index (GI) test
Seed germination and root length tests were conducted on water extracts obtained by mechanically shaking fresh samples for 1 hr at a solid/double-distilled water ratio of 1:10 (w/v, dry weight basis). Approximately 5.0 mL of each extract was pipetted into a sterilized plastic Petri dish lined with a Whatman no. 2 filter paper. Ten radish seeds were evenly placed on the filter paper and incubated at 25 °C in the dark for 72 hr. Triplicate measurements were made for each composting mixture. Treatments were evaluated by counting the number of germinated seeds and by measuring the length of roots. The responses were calculated as the GI, which was determined according to eq 2 (Zucconi et al. Citation1981):
Statistical analysis
Statistical analysis was performed using the Prism 5 Graph Pad program. Data are presented as means (SEM) and analyzed by one-way analysis of variance (ANOVA), followed by Bonferroni’s multiple comparison test with a significance level of P < 0.05.
Results and discussion
Temperature profiles
Temperature variation during the composting process has been widely recognized as an important factor that directly correlates with microbial activity. According to the evolution of temperature, the composting process can be divided into three phases: mesophilic, thermophilic, and curing (Nada Citation2015). shows the variations in the composting temperature and ambient temperature of the cylindrical and rectangular reactors. The temperature of the middle layer of the compost was always the highest, and the temperature in the lower layer was always the lowest owing to the cooling effect of incoming air. The initial temperature in all reactors was 25 °C, but after a few hours this started to increase. In the reactors with aeration rates of 0.3 and 0.6 L min−1 kg−1 DM, temperatures of above 55 °C were recorded. In contrast, the reactors with aeration rates of 0.9 L min−1 kg−1 DM, temperatures were below 50 °C throughout the experiment due to the high aeration rate. The increase in temperature may have been due to the high availability of C, which provides favorable conditions for the growth of microorganisms (Novinscak, Surette, and Filion Citation2007). Troy et al. (Citation2012) reported that temperatures rise quickly in all reactors during the heating phase, indicating a rapid establishment of microbial activity. At day 1, the temperatures at the 0.3, 0.6, and 0.9 L min−1 kg−1 DM aeration rates were 40, 40, and 39 °C, respectively, for the cylindrical reactors, and 39, 39, and 38 °C, respectively, for the rectangular reactors. The thermophilic phase began in all reactors on day 2, with this phase lasting longer at the 0.3 and 0.6 L min−1 kg−1 DM aeration rates than it did at the highest aeration rate. The highest temperatures were recorded in all reactors on days 2–4. On day 3, the temperatures at the 0.3, 0.6, and 0.9 L min−1 kg−1 DM aeration rates were 55, 54, and 47 °C, respectively, for the cylindrical reactors, and 53, 52, and 47 °C, respectively, for the rectangular reactors. Due to microbial activity, smaller variation in temperature may occur throughout the composting process. The temperatures at the low aeration rate (0.3 L min−1 kg−1 DM) were above 55 °C for 2 days, which is considered sufficient to destroy pathogens (Strauch and Ballarini Citation1994). At the start, due to the rapid breakdown of the available OM and nitrogenous compounds by microbial activities, the temperature of all the compost mixtures rapidly increased. In both the cylindrical and rectangular reactors, the high temperatures lasted longer at the 0.3 L min−1 kg−1 DM aeration rate than at the 0.6 and 0.9 L min−1 kg−1 DM rates. The aeration system controls the temperature of a composting material, with a high aeration rate reducing thermophilic microbial activity in the substrate (Petric, Šestan, and Šestan Citation2009). The aeration rate might be considered an important factor affecting temperature patterns in the substrate. The temperature starts to decrease after the thermophilic phase due to a loss of substrate and a reduction in microbial activity (Ogunwande et al. Citation2008). The rise and fall in temperature have been found to strongly correlate with the microbial activity. Temperature is an excellent indicator of the microbial activity in a composting pile (Bernal, Alburquerque, and Moral Citation2009).
Figure 2. Variations in temperature of cylindrical and rectangular reactors during the composting process.
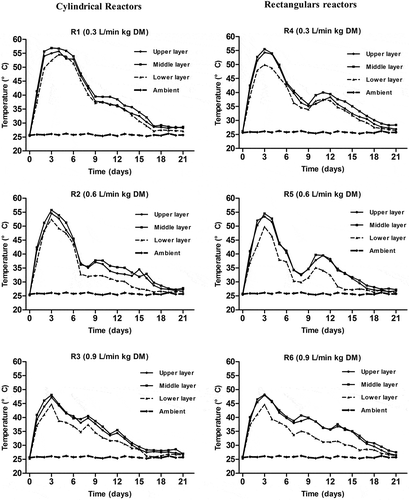
Comparing the cylindrical and rectangular reactors at an aeration rate of 0.3 L min−1 kg−1 DM, the temperature in reactors R1 and R4 reached in excess of 55 °C on day 3; however, the temperature in the rectangular reactor decreased more rapidly than that in the cylindrical reactor, perhaps due to the higher SA/V ratio. Conductive and radiative heat losses depend on the external surface of the reactor; as the SA/V increases, the potential for heat loss from the reactor walls increases (VanderGheynst, Gossett, and Walker Citation1997). The same phenomenon occurred for R2 and R5 with an aeration rate of 0.6 L min−1 kg−1 DM; however, there was little difference observed between R3 and R6 with an aeration rate of 0.9 L min−1 kg−1 DM.
Evaluation of ammonia and carbon dioxide
Ammonia volatilization increased in all reactors at the beginning of the experiment and then gradually decreased (). At the start of composting, ammonia volatilization in all reactors increased with increasing temperature, which comports with findings reported by Dewes (Citation1996). In all reactors, the highest ammonia volatilization rates were observed during the thermophilic phase. The highest ammonia volatilization rates in R1 were observed on days 2–4, but little ammonia was lost after the thermophilic phase, probably due to the presence of little digestible organic N. The lowest ammonia volatilization rates were observed in R3 and R6, although during the later stages of the process, the rates in R3 and R6 were higher than those of the other reactors, likely owing to the transformation of digestible organic N to ammonium. In the first week of composting, there were no significant differences in ammonia volatilization across the reactors (P > 0.05); however, in the second week, significant differences were observed between R1 and R3, R1 and R5, R1 and R6, and R2 and R6 (P < 0.05). In the third week, significant differences were observed between all reactors (except for R1 vs. R2, R3 vs. R4, R3 vs. R5, and R4 vs. R5; P < 0.05). This study confirms that increase in temperature and increase in aeration rate increase ammonia volatilization. Previous studies also reported that an increased aeration rate is responsible for an increase in ammonia (NH3) emissions (de Guardia et al. Citation2008). Zhang et al. (Citation2016) concluded after comparing three treatments in his study that a higher aeration rate resulted in more NH3 emission.
Volatilization of carbon dioxide closely followed the temperature profile of the substrate. The volatilization of carbon dioxide increased in all reactors at the beginning of the process and then started to decrease gradually (). Guo et al. (Citation2012) reported that CO2-C concentrations in the outlet air during composting were significantly correlated to their temperatures. Carbon dioxide volatilization was higher during the thermophilic stage, mainly due to the degradation of easily degradable C under healthy bacterial and fungal conditions (Nada Citation2015). During the maturation stage, the bacterial activity decreased; therefore, carbon dioxide volatilization was reduced. The mass of carbon dioxide was directly proportional to microorganism activity during composting. There were no significant differences in carbon dioxide volatilization across the reactors during the first week of composting. However, significant differences were observed during the maturation or curing stage (P < 0.05). The stability of compost can be assessed by the evaluation of carbon dioxide production. In the maturation or curing stage, the lowest carbon dioxide values were observed in R1 and R4 due to the higher compost stability in these reactors compared with the others. The highest carbon dioxide values were observed in R3, R5, and R6, which suggests the presence of unstable and immature compost in need of further decomposition.
Influence of temperature on ammonia volatilization
Temperature and pH are the main factors that contribute to ammonia volatilization. Ammonia emissions commence when both thermophilic temperatures (>45 °C) and high pH (approximately 9) are present in the compost environment (Beck, Smars, and Jonsson Citation2001). In , the ammonia volatilization observed in all reactors is presented in relation to temperature. It can be observed that the trend lines for all reactors are more likely exponential than linear. For all reactors, an exponential fit showed the positive correlation between temperature and ammonia volatilization. For all reactors, the R2 values of the exponential trend lines were higher than those of the linear trend lines. This demonstrates that the correlation between ammonia and temperature was indicative of exponential growth. Ammonia emission has been shown to be exponentially correlated with temperature during the first stage of composting (Pagans et al. Citation2006); however, the influence of compost temperature on ammonia emissions is not clearly understood (Beck, Smars, and Jonsson Citation2001).
Compost settlement and volume reduction
Settlement of compost can also affect the process to achieve successful composting; it starts as soon as the compost is put in the reactors. Settlement can lead to reduction in porosity and thus volume of compost reduces after few days; such occurrence can considerably affect the efficiency of oxygen supply and water evaporation and heat ventilation rates in a material (Barrington et al. Citation2003; Chang Citation2004).
In this study, although we didn’t focus on the influence of settlement on different parameters, we observed a reduction in volume of compost up to 10 cm, comparing the initial and final volumes of the compost. It might be due to taking samples from the compost every second day. Due to mixing of compost before sampling, the porosity remained the same during the whole experiment.
Changes in physiochemical characteristics during composting
pH
The pH level is an important characteristic of compost material. The optimum range for pH during composting is 5.5–8.0 (Venglovsky et al. Citation2005). The change in pH is also an indicator of the maturity of the compost. At the end of our study, the pH values in all reactors were found to be lower than the initial values. The initial pH in each reactor was 7.8, and it decreased to 6.89, 6.98, 6.83, 6.95, 7.12, and 7.07 in R1, R2, R3, R4, R5, and R6, respectively, as shown in (). The increase and decrease in pH are directly related to the ammonia content in the compost. This decrease is due to an increase in the production of organic acids and/or an increase in nitrification as a result of H+ released during microbial nitrification (Chen et al. Citation2015). Eklind and Kirchmann (Citation2000) reported that the decrease in pH with composting time is caused by a reduction in the volatilization of ammonical N and the release of carbon dioxide during the composting process. Some of this decrease may also have been caused by the production of organic acids in the compost (Sweeten and Auvermann Citation2008).
Table 2. Initial and final physiochemical properties of compost in all reactors.
Electrical conductivity (EC)
The EC of compost illustrates its degree of salinity, which is a potential indicator of its phytotoxic effect when used as a fertilizer (Lin Citation2008). An EC higher than 4 dS/m (Chen Citation1999) adversely influences plant growth, e.g., by producing low germination rates and withering. At the end of our study, the EC values in all reactors were found to be lower than initial values. The initial EC value in all reactors was 2.12 mS/cm, and this decreased to 1.0, 1.2, 1.5, 1.3, 1.5, and 1.6 mS/cm in R1, R2, R3, R4, R5, and R6, respectively, as shown in (). The initial EC may increase owing to the release of mineral salts such as phosphate and ammonium ions; however, during the maturation or curing stage, the EC starts to decrease owing to the evaporation of ammonium ions and the reduction of other basic groups (Wong, Li, and Wong Citation1995).
Moisture content
Moisture content should be maintained within an optimum range during composting to permit microbial growth and activity (Sesay, Lasaridi, and Stentiford Citation1998). At the end of our study, slight decreases in moisture content were observed in all reactors. The initial moisture content in all reactors was set at 65%, and the final moisture contents were recorded as 63.7%, 62.3%, 63.2%, 62.2%, 63.4%, and 62.3% in R1, R2, R3, R4, R5, and R6, respectively, as shown in . This decrease may have been caused by aeration and heat formation in the compost; at high aeration rates, the moisture content reductions were greater than those at low aeration rates. Arslan, Ünlü, and Topal (Citation2011) and Walker et al. (Citation1999) also reported that drying of the solid matrix increased with increases in aeration.
C/N ratio
The C/N ratio usually indicates the degree of maturity of compost (Bernal et al. Citation1998). The initial C/N ratio in all reactors was set to 25, and the final C/N ratios were 20.2, 22.5, 22.8, 21.5, 22.7, and 23.4 in R1, R2, R3, R4, R5, and R6, respectively, as shown in . The greatest reductions were observed in R1 and R4, which had an airflow rate of 0.3 L min−1 kg−1 DM. In the literature, the lower limit C/N ratio for mature compost is accepted to be >12 (Benito et al. Citation2003). In various previous studies, C/N ratios lower than those that we observed were reported; this is due to the longer periods of composting in those studies.
Organic matter degradation
The loss of organic matter from compost is related to microbial degradation/respiration (Paredes et al. Citation2002). The rate of organic matter loss is an indicator of the overall composting rate. The organic matter contents decreased in all reactors during the composting process due to microbial activity. The initial organic matter content in all reactors was 85%, and this decreased to 73.9%, 77.9%, 78.3%, 74.8%, 79.6%, and 78.8% in R1, R2, R3, R4, R5, and R6, respectively, as shown in . The greatest losses of organic matter were observed in R1 and R4, which had an airflow rate of 0.3 L min−1 kg−1 DM. This may be because R1 and R4 provided an adequate oxygen concentration for microorganisms, which enhanced compost material biodegradation. There were significant differences between R1 and the other reactors (except R4; P < 0.05). At the end of experiment, the volume and mass of composting materials were considerably reduced.
Table 3. Degradation of organic matter (dw) in all compost reactors.
Mesophilic and thermophilic bacterial populations
Composting can be an aerobic as well as anaerobic process, with organic waste degradation occurring due to a biological activity of microorganisms (Partanen et al. Citation2010). Both bacteria and fungi produce biological activity during the composting process (Gray, Sherman, and Biddlestone Citation1971). According to temperature, mesophilic and thermophilic bacteria play an important role during the composting process. shows the growth of mesophilic and thermophilic bacteria in all reactors at different stages of the process. In all reactors, as the temperature started to increase, mesophilic bacteria also increased until the start of the thermophilic phase. Thermophilic bacteria increased as the condition shifted from mesophilic to thermophilic. All the microbial populations depend on the early stages of the process. Ryckeboer et al. (Citation2003) also reported that one of the factors affecting microbial communities is the aerobic condition at start of the composting process, which directly affects the temperature of the substrate.
Phytotoxicity assay
The GI is an indicator of compost phytotoxicity (Gao et al. Citation2010). A low GI suggests high toxicity, which is also related to excessive salinity (Tiquia Citation2010). At end of the composting process, the GI% values were 82.3%, 71.4%, 61.7%, 68.8%, 63.6%, and 56.3% in R1, R2, R3, R4, R5, and R6, respectively (). This shows that R6 had the highest level of toxicity; this reactor also had the highest EC value compared with the other reactors. R1 had the GI% above 80%, which indicates the low level of toxicity. Cunha et al. (Citation2007) reported that a GI of more than 80% is an indication of a phytotoxic-free medium for plants. Significant differences were observed between R1 and all other reactors (P < 0.05). Moreover, significant differences were observed between R6 and all other reactors (P < 0.05). However, there were no significant differences between R2 and R5 and between R3 and R5 (P > 0.05).
Figure 6. Germination index (GI%) values of all compost reactors at the end of the composting process.
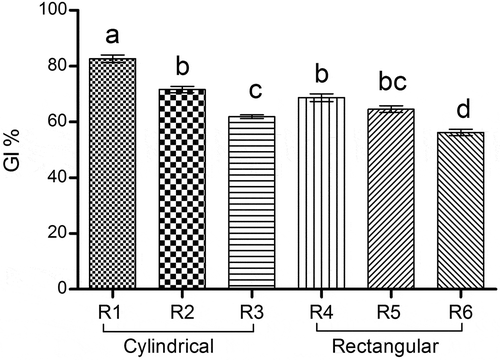
The differences in GI between the composts were due to microbial activity, which is affected by aeration rate (Chen et al. Citation2015). Owing to the longer periods of composting used, the GI values reported in several previous studies are higher than our results.
Conclusion
It can be concluded from these experiments that combination of rectangular or cylindrical reactors with high aeration rates is not suitable for the composting due to rapid heat loss. The heat loss from rectangular reactors was higher than that of cylindrical reactors owing to the higher SA/V ratio of the rectangular reactors. Aeration rate of 0.3 L min−1 kg−1 DM in a cylindrical reactor is recommended to maintain longest phase of thermophilic temperatures ≥55 °C. However, it is advised to keep the compost in reactors a bit longer than in this study to get more mature compost.
Additional information
Funding
Notes on contributors
Waqas Qasim
Waqas Qasim was a master's student of biosystems engineering in the College of Agriculture and Life Sciences, Gyeongsang National University, Jinju 52828, Korea.
Byeong Eun Moon
Byeong Eun Moon is a Ph.D. student of biosystems engineering in the College of Agriculture and Life Sciences, Gyeongsang National University, Jinju 52828, Korea.
Frank Gyan Okyere
Frank Gyan Okyere is a master's student of biosystems engineering in the College of Agriculture and Life Sciences, Gyeongsang National University, Jinju 52828, Korea.
Fawad Khan
Fawad Khan is a master's student of biosystems engineering in the College of Agriculture and Life Sciences, Gyeongsang National University, Jinju 52828, Korea.
Mohammad Nafees
Mohammad Nafees is a professor in the environmental science department, University of Peshawer, KPK, Pakistan.
Hyeon Tae Kim
Hyeon Tae Kim is a professor in the College of Agriculture and Life Sciences, Gyeongsang National University (Institute of Agriculture & Life Science), Jinju 52828, Korea.
References
- Ahn, H. K., T. L. Richard, and H. L. Choi. 2007. Mass and thermal balance during composting of a poultry manure—wood shavings mixture at different aeration rates. Process Biochem. 42 (2):215–23. doi:10.1016/j.procbio.2006.08.005.
- APHA (1995) Standard Methods for the Examination of Water and Wastewater. 19th Edition, American Public Health Association Inc., New York.
- Arslan, E. I., A. Ünlü, and M. Topal. 2011. Determination of the effect of aeration rate on composting of vegetable–Fruit wastes. CLEAN–Soil Air Water 39 (11):1014–21. doi:10.1002/clen.v39.11.
- Barrington, S., D. Choinière, M. Trigui, and W. Knight. 2003. Compost convective airflow under passive aeration. Bioresour. Technol. 86 (3):259–66. doi:10.1016/S0960-8524(02)00155-4.
- Beck, F. B., S. Smars, and H. Jonsson. 2001. Gaseous emissions of carbon dioxide, ammonia and nitrous oxide from organic household waste in a composting reactor under different temperature regimes. Agric. Eng. Res. 78 (4):423. doi:10.1006/jaer.2000.0662.
- Benito, M., A. Masaguer, A. Moliner, N. Arrigo, and R. M. Palma. 2003. Chemical and microbiological parameters for the characterisation of the stability and maturity of pruning waste compost. Biol. Fertil. Soils 37 (3):184–89. doi:10.1007/s00374-003-0584-7.
- Bernal, M. P., C. Paredes, M. A. Sanchez-Monedero, and J. Cegarra. 1998. Maturity and stability parameters of composts prepared with a wide range of organic wastes. Bioresour. Technol. 63 (1):91–99. doi:10.1016/S0960-8524(97)00084-9.
- Bernal, M. P., J. A. Alburquerque, and R. Moral. 2009. Composting of animal manures and chemical criteria for compost maturity assessment. Rev. Bioresour. Technol. 100:5444e5453.
- Chang, Z. 2004. Effects of temperature on the structure of a compost bed- compress, porosity and permeability. J. Agro-Environ. Sci. 23 (4):791–795.
- Chen, J. H. (1999). Characteristic and applications of domestic animal wastes. Animal waste compost products quality and treatment alternatives manual. Soil Survey and Testing Center, Department of Soil and Environmental Science, National Chung Hsing University, Taiwan, 15–22.
- Chen, Z., S. Zhang, Q. Wen, and J. Zheng. 2015. Effect of aeration rate on composting of penicillin mycelial dreg. J. Environ. Sci. 37:172–78. doi:10.1016/j.jes.2015.03.020.
- Cunha, Q. A. C., H. M. Ribeiro, A. Ramos, and F. Cabral. 2007. Study of biochemical and microbiological parameters during composting of pine and eucalyptus bark. Bioresour. Technol. 98:3213–20. doi:10.1016/j.biortech.2006.07.006.
- de Guardia, A., C. Petiot, D. Rogeau, and C. Druilhe. 2008. Influence of aeration rate on nitrogen dynamics during composting. Waste Manage. 28:575–87. doi:10.1016/j.wasman.2007.02.007.
- Dewes, T. 1996. Effect of pH, temperature, amount of litter and storage density on ammonia emissions from stable manure. J. Agri. Sci. 127 (4):501–09. doi:10.1017/S0021859600078722.
- Diaz, M. J., E. Madejón, F. López, R. López, and F. Cabrera. 2002. Optimization of the rate vinasse/grape marc for co-composting process. Process Biochem. 37 (10):1143–50. doi:10.1016/S0032-9592(01)00327-2.
- Eklind, Y., and H. Kirchmann. 2000. Composting and storage of organic household waste with different litter amendments. II: Nitrogen turnover and losses. Bioresour. Technol. 74 (2):125–33. doi:10.1016/S0960-8524(00)00005-5.
- Gao, M., B. Li, A. Yu, F. Liang, L. Yang, and Y. Sun. 2010. The effect of aeration rate on forced-aeration composting of chicken manure and sawdust. Bioresour. Technol. 101 (6):1899–903. doi:10.1016/j.biortech.2009.10.027.
- Gray, R. K., K. Sherman, and A. J. Biddlestone. 1971. Review of composting to the practical process. Process Biochem. 6 (10):44–49.
- Guo, R., G. Li, T. Jiang, F. Schuchardt, T. Chen, Y. Zhao, and Y. Shen. 2012. Effect of aeration rate, C/N ratio and moisture content on the stability and maturity of compost. Bioresour. Technol. 112:171–78. doi:10.1016/j.biortech.2012.02.099.
- Hong, J. H., J. Matsuda, and Y. Ikeuchi. 1983. High rapid composting of dairy cattle manure with crop and forest residues. Trans. ASAE 26 (2):533–41. doi:10.13031/2013.33974.
- Kulcu, R., and O. Yaldiz. 2004. Determination of aeration rate and kinetics of composting some agricultural wastes. Bioresource Technology 93 (1):49-57.
- Kuter, G. A., H. A. J. Hoitink, and L. A. Rossman. 1985. Effects of aeration and temperature on composting of municipal sludge in a full-scale vessel system. J. Water Pollut. Control Fed. 309–15.
- Lau, A. K., K. V. Lo, P. H. Liao, and J. C. Yu. 1992. Aeration experiments for swine waste composting. Bioresour. Technol. 41 (2):145–52. doi:10.1016/0960-8524(92)90185-Z.
- Leth, M., H. Jensen, and J. Iversen. 2001. Influence of different nitrogen sources on composting of miscanthus in open & closed systems. Compost Sci. Util. 9 (3):197–205. doi:10.1080/1065657X.2001.10702036.
- Li, X., R. Zhang, and Y. Pang. 2008. Characteristics of dairy manure composting with rice straw. Bioresour. Technol. 99 (2):359–67. doi:10.1016/j.biortech.2006.12.009.
- Lin, C. 2008. A negative-pressure aeration system for composting food wastes. Bioresour. Technol. 99 (16):7651–56. doi:10.1016/j.biortech.2008.01.078.
- Lu, S. G., T. Imai, H. F. Li, M. Ukita, M. Sekine, and T. Higuchi. 2001. Effect of enforced aeration on in-vessel food waste composting. Environ Technol 22 (10):1177–82. doi:10.1080/09593332208618200.
- Magalhaes, A. M. T., P. J. Shea, M. D. Jawson, E. A. Wicklund, and D. W. Nelson. 1993. Practical simulation of composting in the laboratory. Waste Manage. Res. 11 (2):143–54. doi:10.1177/0734242X9301100206.
- Mason, I. G., and M. W. Milke. 2005. Physical modelling of the composting environment: A review. Part 1: Reactor systems. Waste Manage. 25 (5):481–500. doi:10.1016/j.wasman.2005.01.015.
- Nada, W. M. 2015. Stability and maturity of maize stalks compost as affected by aeration rate, C/N ratio and moisture content. J. Soil Sci. Plant Nutr. 15 (3):751–64. doi:10.4067/S0718-95162015005000051.
- Novinscak, A., C. Surette, and M. Filion. 2007. Quantification of Salmonella spp. in composted biosolids using a TaqMan qPCR assay. J. Microbiol. Methods 70 (1):119–26. doi:10.1016/j.mimet.2007.03.019.
- Ogunwande, G. A., J. A. Osunade, K. O. Adekalu, and L. A. O. Ogunjimi. 2008. Nitrogen loss in chicken litter compost as affected by carbon to nitrogen ratio and turning frequency. Bioresour. Technol. 99 (16):7495–503. doi:10.1016/j.biortech.2008.02.020.
- Pagans, E., R. Barrena, X. Font, and A. Sánchez. 2006. Ammonia emissions from the composting of different organic wastes. Dependency on process temperature. Chemosphere 62 (9):1534–42. doi:10.1016/j.chemosphere.2005.06.044.
- Paredes, C., M. P. Bernal, J. Cegarra, and A. Roig. 2002. Bio-degradation of olive mill wastewater sludge by its co-composting with agricultural wastes. Bioresour. Technol. 85 (1):1–8. doi:10.1016/S0960-8524(02)00078-0.
- Partanen, P., J. Hultman, L. Paulin, P. Auvinen, and M. Romantschuk. 2010. Bacterial diversity at different stages of the composting process. BMC Microbiol. 10 (1):94. doi:10.1186/1471-2180-10-94.
- Pecchia, J. A., D. M. Beyer, and P. J. Wuest. 2002. The effects of poultry manure based formulations on Odor generation during Phase i mushroom composting. Compost Sci. Util. 10 (3):188–96. doi:10.1080/1065657X.2002.10702080.
- Petric, I., A. Šestan, and I. Šestan. 2009. Influence of wheat straw addition on composting of poultry manure. Process Saf. Environ. Prot. 87 (3):206–12. doi:10.1016/j.psep.2009.02.002.
- Phillip, E. A. 2010. The design and construction of a pilot-scale compost reactor for the study of gas emissions from compost under different physical conditions. Masters Abstr. Int. 49 (05).
- Prescot, L. M., J. P. Harley, and D. A. Kleon. 1996. Microbiology, 498–502. Chichester: WCB Publishers.
- Qasim, W., B. E. Moon, M. Phonsuwan, J. S. Jo, M. H. Lee, M. Nafees, and H. T. Kim. 2017. Effects of an aluminum sulfate and ferric chloride blend on poultry litter characteristics in vitro. J. Appl. Poult. Res. 27 (1):92–102. doi:10.3382/japr/pfx046.
- Ryckeboer, J., J. Mergaert, J. Coosemans, K. Deprins, and J. Swings. 2003. Microbiological aspects of biowaste during composting in a monitored compost bin. Journal Of Applied Microbiology 94 (1):127-137.
- Schloss, P. D., B. Chaves, and L. P. Walker. 2000. The use of the analysis of variance to assess the influence of mixing during composting. Process Biochem. 35 (7):675–84. doi:10.1016/S0032-9592(99)00117-X.
- Sesay, A. A., K. E. Lasaridi, and E. I. Stentiford. 1998. Aerated static pile composting of municipal solid waste (MSW): A comparsion of positive pressure aeration with hybrid positive and negative aeration. Waste Manage. Res. 16 (3):264–72. doi:10.1177/0734242X9801600308.
- Strauch, D., and G. Ballarini. 1994. Hygienic aspects of the production and agricultural use of animal wastes. Zoonoses Public Health 41 (1‐10):176–228. doi:10.1111/j.1439-0450.1994.tb00222.x.
- Sweeten, J. M., and B. W. Auvermann. 2008. Composting manure and sludge. Agrilife Extension 479:06e08.
- Tiquia, S. M. 2010. Reduction of compost phytotoxicity during the process of decomposition. Chemosphere 79 (5):506–12. doi:10.1016/j.chemosphere.2010.02.040.
- Troy, S. M., T. Nolan, W. Kwapinski, J. J. Leahy, M. G. Healy, and P. G. Lawlor. 2012. Effect of sawdust addition on composting of separated raw and anaerobically digested pig manure. J. Environ. Manage. 111:70-77.
- USDA. 2017. www.fas.usda.gov/data/south-korea-poultry-and-products-annual-2
- VanderGheynst, J. S., and F. Lei. 2003. Microbial community structure dynamics during aerated and mixed composting. Trans. ASAE 46 (2):577–84. doi:10.13031/2013.12912.
- VanderGheynst, J. S., J. M. Gossett, and L. P. Walker. 1997. High-solids aerobic decomposition: Pilot-scale reactor development and experimentation. Process Biochem. 32 (5):361–75. doi:10.1016/S0032-9592(96)00073-8.
- Venglovsky, J., N. Sasakova, M. Vargova, Z. Pacajova, I. Placha, M. Petrovsky, and D. Harichova. 2005. Evolution of temperature and chemical parameters during composting of the pig slurry solid fraction amended with natural zeolite. Bioresour. Technol. 96 (2):181–89. doi:10.1016/j.biortech.2004.05.006.
- Walker, L. P., T. D. Nock, J. M. Gossett, and J. S. VanderGheynst. 1999. The role of periodic agitation and water addition in managing moisture limitations during high-solids aerobic decomposition. Process Biochem. 34 (6):601–12. doi:10.1016/S0032-9592(98)00122-8.
- Wong, J. W. C., S. W. Y. Li, and M. H. Wong. 1995. Coal fly ash as a composting material for sewage sludge: Effects on microbial activities. Environ Technol 16 (6):527–37. doi:10.1080/09593331608616294.
- Zhang, H., G. Li, J. Gu, G. Wang, Y. Li, and D. Zhang. 2016. Influence of aeration on volatile sulfur compounds (VSCs) and NH3 emissions during aerobic composting of kitchen waste. Waste Manage. 58:369–75. doi:10.1016/j.wasman.2016.08.022.
- Zucconi, F., A. Pera, M. Forte, and M. A. R. C. De Bertoldi. 1981. Evaluating toxicity of immature compost. BioCycle 22:54–7.