ABSTRACT
The effluent generated from poultry waste processing industries contains several organic compounds such as collagen, gelatin, bovine serum albumin, carbohydrates, essential fatty acids, and so forth. This enabled the establishment of poultry waste processing industries to produce value-added products such as animal feed and organic fertilizers. During poultry waste processing, huge amounts of ammoniacal nitrogen and organic pollutants such as proteins, various carbohydrates, and fatty materials are discharged into the effluent stream which contributes to several environmental issues. Because of the shortcomings of the current conventional treatment, the present study is about with the development of a sequential bioreactor system for the effective treatment of poultry waste processing industrial effluent. Facultative anaerobe Paracoccus pantotrophus FMR19 along with the indigenous isolate Bacillus albus MN527241 obtained from clarifying sludge was used as mixed consortia for the treatment of poultry waste processing industrial effluent. The mixed microbial consortia resulted in the maximum activity of enzymes such as protease (247 U/mL) and lipase (28.266 U/mL) thereby achieving 90% of ammoniacal nitrogen reduction and 98% of COD removal within five days. Further, the confirmatory analysis of poultry effluent treatment was carried out using gas chromatography-mass spectroscopy (GC-MS), High-Performance Liquid Chromatography (HPLC), Fourier Transform Infrared Spectroscopy (FT-IR), and SDS-PAGE. Hence, the sequential bioreactor-based treatment approach has proved to be highly effective in removal of organic pollutants in the poultry waste processing industrial effluent.Implications: The poultry waste processing industrial (PWPI) effluent contains huge ammoniacal nitrogen and COD and affects the environment. Aerobic moving bed biofilm reactor and up-flow anaerobic sludge blanket reactor are in current practice and shows considerable reduction in the ammoniacal nitrogen and COD in long retention time. Therefore, there is a need of sustainable treatment process that could effectively remove the organic pollutants from the effluent in short duration. Our study focused on the application of sequential bioreactor approach for the treatment of PWPI using aerobic followed by anaerobic treatment process and observed efficient organic pollutants removal in short duration.
Introduction
In India, the poultry processing industries are one of the major contributors to the regional economic development as well as employment in the country (Chatterjee and Rajkumar Citation2015). Meat processing industry (including poultry) is a major consumer of freshwater amongst food processing industries (Bustillo-Lecompte and Mehrvar Citation2015). This leads to the generation of huge quantities of wastewater. More particularly, India has unorganized management system for the disposal of waste produced during poultry processing sectors.The effluent generated from poultry industries are rich in proteins, blood residues, fats, and lard (Yaakob et al. Citation2018) resulting in high organic load and ammoniacal nitrogen (Cao and Mehrvar Citation2011). Also, due to the presence of huge amounts of organic pollutants in the effluent, their discharge in the water bodies without prior treatment creates heavy odor which could attracts flies, rodents, and other pests carrying diseases and could cause several health and environmental hazards (Steinfeld et al. Citation2006). In order to combat the effect, the biomolecules present in the poultry industrial effluent are being utilized for the production of value-added products such as animal feed, biodiesel, biogas, organic fertilizer, chitosan, natural pigments, collagen, gelatin, and so forth. (Jayathilakan et al. Citation2012). The recovery of value-added products not only reduces environmental pollution, but also generates additional revenue for the industry. The steps involved in the production of animal feed and fertilizers creates a necessity for poultry waste processing industrial effluent to undergo further treatment to reduce the residual organic load and other contaminants to the prescribed permissible levels (Bustillo-Lecompte and Mehrvar Citation2015). According to a few reports, the environmental impacts caused by the improper discharge of poultry waste processing industrial effluent include: eutrophication (Yaakob et al. Citation2018), groundwater contamination, soil pollution, bio-accumulation of recalcitrant compounds, and even a loss of biodiversity. Treatment approach in industries like Aerobic Moving Bed Biofilm Reactor (MBBR) and Up-flow Anaerobic Sludge Blanket (UASB) are currently in use. Though the MBBR technology was found to be cost effective, it showed low efficiency in terms of chemical oxygen demand (COD) and ammoniacal nitrogen removal as well as it has Hydraulic Retention Time (HRT) of around 28 days. The UASB reactor provided the removal efficiencies around 70% and 79% of total chemical oxygen demand and soluble chemical oxygen demand, respectively, but it takes 147 days for the treatment (Rajakumar et al. Citation2011). Researchers have reported that the use of UASB combined with Fenton’s oxidation technology as a post treatment unit provided 99.3% reduction in COD at the end of 73 days of treatment, which is still a time-consuming technology (Yetilmezsoy and Sakar Citation2008). Therefore, in our study we have focused on the treatment of poultry waste processing industrial effluent using a sequential bioreactor wherein aerobic treatment was followed by anaerobic digestion for efficient reduction of high concentration of ammoniacal nitrogen and organic load as well as the sludge reduction. The indigenous microbes isolated from clarifying slurry tank were used in this study. The effect of each isolates were tested along with our previously isolated animal flesh degrading microbial strain Paracoccus pantotrophus FMR19 (Faridha Begum et al. Citation2016) for the enhanced removal of COD and ammoniacal nitrogen. Studies on the treatment condition such as time, pH and biomass concentration were optimized for the sequential bioreactor system. Amongst the indigenous isolates, Bacillus albus MN527241 along with the P. Pantotrophus as a mixed consortium showed maximum activity of enzymes such as protease and lipase which aids in the efficient removal of organic pollutant present in poultry waste processing industrial (PWPI) effluent within a short duration of time.
Materials and methods
The PWPI effluent used in the present study was collected from a poultry waste processing industry in Kerala through the grab sampling method. The collected effluent was stored at 4°C till usage. All the chemicals and media used in this study were of analytical grade. The mother culture of Paracoccus pantotrophus FMR19 was maintained in 2% nutrient agar slant, and further, the strain was periodically subcultured and stored at room temperature until use.
Characterization of poultry waste processing industrial effluent
The initial physico-chemical parameters of the PWPI effluent such as COD and ammoniacal nitrogen content were estimated according to standard protocol as mentioned in American public health association (APHA) (APHA Citation1998). The protein and lipid concentration were estimated using Lowry’s method (Lowry et al. Citation1951) and Park et al. (Citation2016), respectively. Instrumental analysis such as gas chromatography-mass spectroscopy (GC-MS), Fourier Transform Infrared Spectroscopy (FT-IR), and High-Performance Liquid Chromatography (HPLC) were performed to detect the presence of long chain fatty acids, functional groups, and amino acid compositions, respectively.
Isolation, screening, and identification of efficient strain for the treatment of high strength PWPI effluent
The microbial strains were isolated from indigenous source as follows. Secondary clarifying tank slurry was collected from poultry waste processing industry effluent treatment plant and the organisms were isolated using serial dilution and spread plating methods. Three strains were obtained and labeled as S1, S2, and S3. The enrichment process of the isolates was carried out by transferring 2 g of biomass of each strain to 100 ml PWPI effluent and incubated for 7 days. The individual effect of the enriched strains along with P. Pantotrophus FMR19 was studied in order to determine their efficiency in reduction of COD and ammoniacal nitrogen content. Amongst the three combinations, the synergistic effect of the strain S2 and P. Pantotrophus FMR19 showed maximum protease and lipase enzyme activity as well as better removal of COD and ammoniacal nitrogen.
The selected strain (S2) was identified using 16S rRNA sequencing. Colony polymerase chain reaction (PCR) was done to amplify the target 16SrRNA region of the DNA of strain (S2). The forward primer, 27F (5′-AGA GTT TGA TCC TGG CTC AG-3′) and reverse primer 1492R (5′-CGG CTA CCT TGT TAC GAC TT-3) and PCR 262 Master Mix (Thermo Scientific Phusion Flash High-Fidelity) were used for the amplification. The amplified 16S rRNA gene sequences were aligned using the Bioedit and CLUSTALW software. Phylogenetic tree was constructed using the neighbor joining method .
Studies on the treatment of PWPI effluent using sequential bioreactor system
First stage-aerobic treatment for the degradation of organic pollutants in PWPI effluent
Initially, 1.0 g of selected microbial consortia was transferred to 100 mL of PWPI effluent to monitor the activity of lipase and protease enzyme as well as the removal of COD and ammoniacal nitrogen. In order to enhance the enzyme activity and degradation efficiency, the treatment conditions such as time (24, 48, 72, 96 hour), pH (6, 7, 8), and biomass concentration (1% 2%, 3%, and 4% (w/v)) were optimized. Methods to determine the activity of protease and lipase were adopted from (Ramani and Sekaran Citation2012) and (Oberoi et al. Citation2001), respectively.
Second stage-anaerobic treatment for the degradation of residual organics in PWPI effluent
Followed by the aerobic treatment, in order to further reduce the COD, protein, and lipid concentration, anaerobic treatment was carried out. The experiments were performed using 250 mL serum bottle in which 100 mL of aerobically treated effluent was transferred along with the biomass. The flasks consisting of the effluent without microbial consortia served as the control. The reduction in COD, ammoniacal nitrogen, and protein content along with biomolecules production were monitored every day. Also, the crude enzymes were extracted from the treated effluent using acetone precipitation method (Suganthi et al. Citation2018) and subjected to Laemmli-SDS-PAGE (He Citation2011) in order to check the homogeneity and purity of the enzymes produced. The molecular weight of obtained bands were estimated by comparing with the pre-stained broad range protein ladder. Subjecting the effluent to aerobic treatment before anaerobic treatment is effective in the reduction of ammoniacal nitrogen as well as to prevent sludge generation. Thus, according to the optimized conditions, a sequential biological process for the treatment of poultry waste processing industrial effluent was established.
Confirmatory analysis of the treated PWPI effluent
After each stage of treatment process, the effluent was collected and processed for instrumentational analysis such as HPLC, FT-IR, and GC-MS using the methods adopted in (Kanetro, Slamet, and Wazyka Citation2018), (Hepziba Suganthi and Kandasamy Citation2017) and (Suganthi et al. Citation2018), respectively, in order to detect the presence of aminoacids, functional groups, and degradation profile of fatty acid, respectively.
Results and discussion
Physico-chemical parameters of untreated PWPI effluent
The physico-chemical parameters of the untreated PWPI effluent such as COD and ammoniacal nitrogen were found to be 46,500 mg/L and 1,330 mg/L, respectively. The high COD and ammoniacal nitrogen are due to the presence high concentration of biodegradable protein and fatty substances, which was further confirmed by the estimation of protein and lipid content that showed 3,895 mg/L and 178 mg/L, respectively. (See .)
Table 1. Physicochemical parameters of the poultry waste processing industrial effluent before and after treatment
Isolation, screening, and identification of efficient strain for the treatment of high strength PWPI effluent
The three strains labeled as S1, S2, and S3 were isolated from clarifying tank slurry by serial isolation and spread plate method. The individual strains along with P. Pantotrophus were used for treatment, on which the P. pantotrophus+S2 combination showed maximum reduction percentage of ammoniacal nitrogen, protein concentration and COD by 93%, 75%, and 85%, respectively (). Although nearly 89% of COD removal was achieved by P. Pantotrophus when compared to mixed consortia, the removal efficiency of ammoniacal nitrogen content remained less. Hence, this facilitates the selection of mixed consortia (P.pantotrophus+S2) for further studies. The selected indigenous isolate (S2) was identified as Bacillus albus by 16S-rRNA sequencing. The phylogenetic tree was constructed (), and the sequence of Bacillus albus was submitted to NCBI GenBank and obtained the accession number was MN527241.
Studies on the optimization of treatment conditions for the first stage aerobic treatment of PWPI effluent
The effect of time on the reduction of COD, ammoniacal nitrogen, and protein concentration was studied using selected consortia (Bacillus albus & Paracoccus pantotrophus) in PWPI effluent. depicts the maximum reduction in COD and protein by 89% and 75%, respectively, on day 3, and ammoniacal nitrogen around 93% was achieved on day 4 of the treatment process. By keeping time as constant, the effect of biomass concentration was studied. shows the inoculum concentration of 3% (w/v) decreases the COD, ammoniacal nitrogen, and protein concentration by 80%, 92%, and 55%, respectively. The protease enzyme also showed a maximum activity of 688 U/mL at 3% (w/v) inoculum concentration. Hence, the biomass concentration of 3% (w/v) was considered to be optimal. By keeping time and inoculum concentration constant, the effect of different pH ranges (6, 7, and 8) on degradation profile and enzyme activity was studied, and as can be seen from , the pH 7.0 showed 98% and 90% of reduction in COD and ammoniacal nitrogen, respectively, whereas protein was reduced by 45%. The maximum protease activity of 247 U/mL was also observed at pH 7.0, beyond which there was a decline in the activity of enzymes. Therefore, pH 7.0 was considered to be optimal.
Second stage anaerobic treatment for the degradation of residual organics in PWPI effluent
After aerobic treatment process for a period of 3 days with biomass concentration of 3% at pH 7.0, the effluent was transferred to anaerobic system to reduce sludge production in which reduction of COD and protein concentration was 10% and 58%, respectively, on day 2 of treatment. An increase in the ammoniacal nitrogen was observed, which was due to the breakdown of proteins and release of ammonia. After anaerobic treatment, the COD, ammoniacal nitrogen, and protein concentration of the effluent was estimated and were found to be 820 mg/L, 120 mg/L, and 366 mg/L, representing 98%, 90%, and 90% reduction, respectively, from untreated effluent. And also, protein extraction of the anaerobically treated effluent was carried out and it was loaded onto SDS-PAGE; the molecular weight of the proteins were found to be in the range 15–30 kDa, which represents alkaline serine protease. Other protease enzymes such as cysteine protease could be seen at 50 kDa and 65 kDa.
Instrumental confirmation of the sequential treatment of PWPI effluent
In , the HPLC analysis of the untreated effluent depicts the presence of amino acids such as arginine, threonine, valine, phenylalanine, and lysine in high concentration. After stage I, aerobic treatment, the amount of these amino acids was found to be reduced compared to the initial sample in which asparagine, phenylalanine, and tryptophan was significantly reduced. After stage II, anaerobic treatment, these amino acids were further reduced, which was due to the utilization of essential amino acids by microorganisms for its growth and metabolism. The presence of certain amino acids like leucine and proline after the sequential treatment of the effluent suggests that the proteins present in the effluent was hydrolyzed into its respective amino acids (see ). In , the FT-IR spectra of untreated and the treated effluent distinctly represents the degradation of organic compounds. Majorly, N-H stretch found in untreated sample at 2,362 cm−1 (Saravana Kumar and Liakath Ali Khan Citation2016; Daraji Citation2012) is absent in stage I (aerobic) treated sample indicating reduction of ammoniacal nitrogen. After stage II, anaerobic treatment process, a significant difference was observed compared to the untreated effluent. The FT-IR of anaerobically treated sample shows the major peaks at 1,564 cm−1 and 1,417 cm−1, which corresponds to the presence of secondary amines (Jackson et al. Citation2009) and C = N stretching (Jana Citation2015), respectively, and the appearance of peak at 1,639 cm−1 corresponds to tertiary amide (Sjahfirdi and Nasikin Citation2012). The increase in the intensity of the corresponding peaks represents the breakdown of protein into simpler molecules. The peak at 2,958 cm−1, indicates the presence of C–H stretching vibration which was due to the degradation of organic compounds after anaerobic treatment.
Table 2. Comparison of amino acids composition before and after treatment
Figure 3. (a) SDS PAGE of crude enzyme. (b) HPLC analysis. (c) FT-IR spectra of the stage-wise treated poultry waste processing industrial effluent
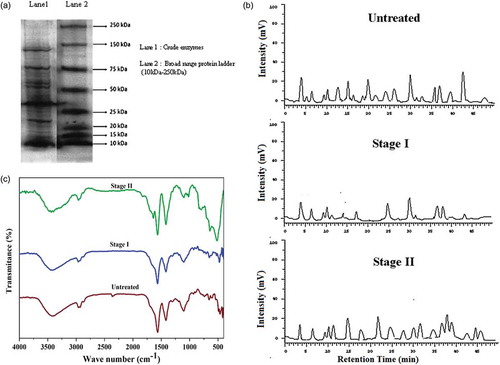
Further, the fatty acids profile of untreated and stage-wise treated effluent was determined by GC-MS analysis as shown in . The chromatogram of initial untreated effluent showed the presence of short chain and long chain fatty acids ranges between C10–C17, which includes Methyl 9-oxononanoate (C10), Methyl n-dodecanoate (C13), Methyl n-tetradecanoate (C15), Methyl n-hexadecanoate (C17), Methyl linoleate (19), Methyl cis-9-octadecenoate (C19), and Methyl n-octadecanoate (C19). After aerobic treatment, the peaks corresponding to short chain fatty acids C10--C17 disappeared, whereas reduction in the peak abundance of the long chain fatty acids C19 was observed. After the anaerobic treatment, there was a further reduction in the number and abundance of these peaks. This shows that the selected bacterial consortia were able to effectively degrade or utilize the fatty acids present in the PWPI effluent.
Conclusion
The present study provides the sustainable treatment strategy for the efficient removal of high COD and ammoniacal nitrogen present in the PWPI effluent. The study signifies that the isolated microbial strain Bacillius albus MN527241 along with the Paracoccus pantotrophus FMR19 as a consortium acts synergistically during the treatment process and thereby affords enhanced degradation of organic compounds as well as removal of ammoniacal nitrogen simultaneously within a very short duration. The GC-MS and HPLC analysis results proved confirmation of treatment efficiency. Moreover, application of the sequential bioreactor system results in removal of COD and ammoniacal nitrogen to a minimum level that could be discharged into the environment without causing any environmental impacts.
Acknowledgment
The authors thank Department of Biotechnology, School of Bioengineering, SRM Institute of Science and Technology for their analytical support.
Disclosure statement
No potential conflict of interest was reported by the authors.
Additional information
Notes on contributors
Ramani K.
Ramani K. is Associate Professor in the Department of Biotechnology, School of Bioengineering, SRM Institute of Science and Technology. She has 14 years of research experience in the field of industrial waste management and Environmental Biotechnology. Thanmaya Mohan, Noorul Shaheen Sheikh Farid, and Sowmya A. are pursuing their final year B. Tech. Biotechnology under the guidance of Dr. K. Ramani in the Department of Biotechnology, SRM Institute of Science and Technology. Swathi K.V. is a Ph.D. scholar doing her research in the Biomolecules and Biocatalysis Laboratory, Department of Biotechnology in SRM Institute of Science and Technology.
References
- APHA. 1998. Standard methods for the examination of water and wastewater, 20th Edn, American Public Health Association, American Water Works Association and Water Environment Federation. https://trove.nla.gov.au/work/16646325?q&versionId=41929314.
- Bustillo-Lecompte, C. F., and M. Mehrvar. 2015. Slaughterhouse wastewater characteristics, treatment, and management in the meat processing industry: A review on trends and advances. J. Environ. Manage. 161 (September):287–302. doi:10.1016/j.jenvman.2015.07.008.
- Cao, W., and M. Mehrvar. 2011. Slaughterhouse wastewater treatment by combined anaerobic baffled reactor and UV/H2O2 processes. Chem. Eng. Res. Des. 89 (7):1136–43. doi:10.1016/j.cherd.2010.12.001.
- Chatterjee, R. N., and U. Rajkumar. 2015. An overview of poultry production in India. Indian J. Anim. Health 54 (2):89–108.
- Daraji, A. H. K. A. 2012. A new procedure for preparation of sulfon-Z compounds. J. Thi-Qar Sci. 3 (2):85–93.
- Faridha Begum, I., R. Mohankumar, M. Jeevan, and K. Ramani. 2016. GC-MS analysis of bio-active molecules derived from paracoccus pantotrophus FMR19 and the antimicrobial activity against bacterial pathogens and MDROs. Indian J. Microbiol. 56 (4):426–32. doi:10.1007/s12088-016-0609-1.
- He, F. 2011. Laemmli-SDS-PAGE. Bio-protocol 1 (11). doi: 10.21769/bioprotoc.80.
- Hepziba Suganthi, S., and R. Kandasamy. 2017. A novel single step synthesis and surface functionalization of iron oxide magnetic nanoparticles and thereof for the copper removal from pigment industry effluent. Sep. Purif. Tech. 188:458–67. doi:10.1016/j.seppur.2017.07.059.
- Jackson, P., K. Robinson, G. Puxty, and M. Attalla. 2009. In situ Fourier Transform-Infrared (FT-IR) analysis of carbon dioxide absorption and desorption in amine solutions. Energy Procedia 1:985–94. doi:10.1016/j.egypro.2009.01.131.
- Jana, S. 2015. Spectroscopic characterization of disulfiram and nicotinic acid after biofield treatment. J. Anal. Bioanal. Tech. 6 (5). doi: 10.4172/2155-9872.1000265.
- Jayathilakan, K., K. Sultana, K. Radhakrishna, and A. S. Bawa. 2012. Utilization of byproducts and waste materials from meat, poultry and fish processing industries: A review. J. Food Sci. Technol. 49:278–93. doi:10.1007/s13197-011-0290-7.
- Kanetro, B., A. Slamet, and A. Wazyka. 2018. Effect of various solvent on the specific amino acids of black soybean (Glycine Soja) Sprout.” IOP Conf. Ser. Earth Environ. Sci. 102 (1). doi:10.1088/1755-1315/102/1/012002.
- Lowry, O. H., N. J. Rosebrough, A. L. Farr, and R. J. Randall. 1951. Protein measurement with the folin phenol reagent. J. Biol. Chem. 193 (1):265–75. http://www.jbc.org/.
- Oberoi, R., Q. K. Beg, S. Puri, R. K. Saxena, and R. Gupta. 2001. Characterization and wash performance analysis of an SDS-stable alkaline protease from a Bacillus Sp. World J. Microbil. Biotechnol. 17 (3):493–97. doi:10.1023/A.
- Park, J., H. J. Jeong, E. Y. Yoon, and S. J. Moon. 2016. Easy and rapid quantification of lipid contents of marine dinoflagellates using the sulpho-phospho-vanillin method. Algae 31 (4):391–401. doi:10.4490/algae.2016.31.12.7.
- Rajakumar, R., T. Meenambal, J. Rajesh Banu, and I. T. Yeom. 2011. Treatment of poultry slaughterhouse wastewater in upflow anaerobic filter under low upflow velocity. Int. J. Environ. Sci. Tech. 8 (1):149–58. doi:10.1007/BF03326204.
- Ramani, K., and G. Sekaran. 2012. Production of lipase from pseudomonas gessardii using blood tissue lipid and thereof for the hydrolysis of blood cholesterol and triglycerides and lysis of red blood cells. Bioprocess Biosyst. Eng. 35 (6):885–96. doi:10.1007/s00449-011-0673-1.
- Saravana Kumar, M., and F. Liakath Ali Khan. 2016. Quantitative prediction of urinary stone samples using partial least square regression coupled with FTIR spectra. Int. J. Adv. Sci. Tech. Res. Issue 6.
- Sjahfirdi, L., and M. Nasikin. 2012. Protein identification using fourier tranform infrared (ftir). IJRRAS 10. www.arpapress.com/Volumes/Vol10Issue3/IJRRAS_10_3_06.pdf418.
- Steinfeld, H., P. Gerber, T. Wassenaar, V. Castel, M. Rosales, and C. de Haan. 2006. Livestock’s long shadow: Environmental issues and options. Renewable Res. J. 24 (4):15–17. http://www.virtualcentre.org.
- Suganthi, S., H. Shabnam Murshid, S. Sriram, and K. Ramani. 2018. Enhanced biodegradation of hydrocarbons in petroleum tank bottom oil sludge and characterization of biocatalysts and biosurfactants. J. Environ. Manage. 220 (December 2017):87–95. doi:10.1016/j.jenv.
- Yaakob, M. A., R. M. S. R. Mohamed, A. A. S. Al-Gheethi, and A. H. M. Kassim. 2018. Characteristics of chicken slaughterhouse wastewater. Chem. Eng. Trans. 63:637–42. doi:10.3303/CET1863107.
- Yetilmezsoy, K., and S. Sakar. 2008. Improvement of COD and color removal from UASB treated poultry manure wastewater using fenton’s oxidation. J. Hazard. Mater. 151 (2–3):547–58. doi:10.1016/j.jhazmat.2007.06.013.