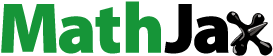
ABSTRACT
In this study, an effective circulating system was developed to remove heavy metals in medical waste incineration (MWI) fly ash. MWI fly ash (MWIFA)-column experiments were performed to remove Cu, Pb, Zn, Cd, and Ni from MWIFA using EDTA disodium (Na2EDTA). Iron-column experiments were conducted to study the removal effect of zero-valent iron on the five heavy metals from washing wastewater. Toxicity Characteristic Leaching Procedure (TCLP) test method was employed to evaluate heavy metals toxicity of MWIFA residues generated after 0–0.2 mol/L Na2EDTA solution treated. After being washed by 0.2 mol/L Na2EDTA solution, TCLP leaching values of Cu, Pb, Zn, Cd, and Ni were the lowest and satisfied the standard (GB 5085.3–2007), and the leaching values were 58.4 ± 2.0 mg/L, 2.81 ± 0.14 mg/L, 64.3 ± 4.0 mg/L, 0.156 ± 0.005 mg/L, 0.381 ± 0.006 mg/L. Concentrations of Cu, Pb, Zn, Cd, and Ni in iron-column effluent were reduced by 99.7%, 91.6%, 91.6%, 75.4%, and 75.7%, respectively. Na2EDTA was recovered and recycled to the removal of heavy metals from MWIFA. Comparing new Na2EDTA solution with recycled Na2EDTA solution, recycled Na2EDTA and water could be reutilized to dispose MWIFA. The removal efficiencies of Cu, Pb, Zn, Cd, and Ni by recycled 0.2 mol/L Na2EDTA solution were 67.1%, 68.8%, 63.2%, 73.9%, 50.7%, respectively, the removal efficiencies using recovered Na2EDTA decreased by 2.6%, 3.9%, 3.3%, 4.2%, and 1.6%, respectively. Successive batch experiments were also conducted to evaluate industrialization potential and reusable times for recycled Na2EDTA. After four recirculation cycles, extraction efficiencies of Pb and Cd (removal efficiency at different cycles divided by removal efficiency of new Na2EDTA) declined toward 80%. Results from this research indicated that this circulating system possessed industrialization potential.
Implications: An effective circulating system was developed to remove heavy metals in MWI fly ash (MWIFA). Integration of Na2EDTA with Fe0 promoted the removal of heavy metals from MWIFA. Na2EDTA, NaCl and water were stepwise extracted from iron-column effluent, respectively. Recovered Na2EDTA can still effectively remove heavy metals from MWIFA. Results from this research indicated that this circulating system possessed industrialization potential.
Introduction
Incineration of dangerous medical waste has significant advantage for both volume and mass reduction of waste as well as decomposition of organic compounds (Kim et al. Citation2019). It can thermally eliminate all pathogens (in the temperature rang of 1,100–1,200°C) (Papamarkou et al. Citation2018; Shaaban Citation2007), and at the same time, it may decrease the waste weight more than 70 wt% (90%, in terms of their original volume) (Alvim-Ferraz and Afonso Citation2005; Kougemitrou et al. Citation2011; Papamarkou et al. Citation2018; Sabiha et al. Citation2008; Tsakalou et al. Citation2018; Zhao et al. Citation2009) It is suitable for all kinds of medical wastes except for specific types such as radioactive or batteries (Tsakalou et al. Citation2018). One of the main drawbacks of the incineration process is that the significant amounts of Medical Waste Incineration (MWI) Bottom Ash (BA) and Fly Ash (FA), usually enriched with toxic substances, are generated (Tsakalou et al. Citation2018). Because of containing highly toxic organic pollutants (dioxins, furans, and polycyclic aromatic hydrocarbons), leachable alkali chlorides, and a lot of soluble heavy metals such as Cu, Pb, Zn, As, Cd, and so on, Medical Waste Incineration Fly Ash (MWIFA) is classified as hazardous wastes (Liu et al. Citation2018; Xie and Zhu Citation2013). Leaching concentrations for many kinds of soluble heavy metals in MWIFA exceed the maximum admissible values for hazardous waste landfill, MWIFA yet cannot fulfill the limited value for deposit in hazardous waste landfill without a prior treatment (Kim et al. Citation2019; Liu et al. Citation2018; Xie and Zhu Citation2013). It is known that metals in MWIFA are not biodegradable, can be easily precipitated into the soil and eventually leached into underground water if not immobilized or removed in time (Wang et al. Citation2012), The dispersion of heavy metals from ash into the air, soil, and water leads to significant environmental pollution (Cozea et al. Citation2018; Necsulescu et al. Citation2008;), which may indirectly cause harm to human beings and the environment. When heavy metals in MWIFA enter environment after long-term leaching, the environment and human health will be seriously threatened. Heavy metals are nonrenewable resources, which should be recycled for sustainable waste management (Deliyanni et al. Citation2017). Developing new recovery technologies for heavy-metal recycling and residual ash disposal is essential (Liu et al. Citation2019). In recent years, many scholars in the world have conducted many investigations related to heavy metals in MWIFA, especially focused on removal of heavy metals from FA (Meer and Nazir Citation2018). Acid leaching pretreatment can facilitate the separation and recovery of heavy metals from MWIFA together with other post-treatment, such as thermal treatment, melting, solvent extraction, sulfidation precipitation, flotation, and immobilization treatment (Liu et al. Citation2017b; Mangialardi Citation2004; Yang et al. Citation2014). Many kinds of lixiviants have been applied in the acid leaching pretreatment process: organic acids, such as tartaric acid, acetic acid, oxalic acid, and citric acid; inorganic acids, such as HNO3, HCl, H2SO4; chelating reagents, such as Diethylenetriaminepentaacetic acid (DTPA), Ethylenediaminetetraacetic acid (EDTA), Ethylenediaminedisuccinic acid (EDDS), and Nitrilotriacetic acid (NTA), citric acid (CA), and alkaline lixiviants, such as sodium hydroxides and ammonium salts (Liu et al. Citation2018; Sumalatha, Naveen, and Malik Citation2019). After acid leaching, soluble heavy metals such as Zn and Pb are almost completely transferred into liquid phase from solid phase, which is more easily removed and recovered in a subsequent disposal process (Liu et al. Citation2019). In previous literatures, comprehensive comparison of extensive lixiviants utilized for removal of heavy metals from three kinds of FA (Municipal solid waste incineration fly ash, MWIFA, and coal FA) are conducted by studying in detail their reaction conditions, metals leached, and percentage extraction achieved (Liu et al. Citation2018; Meer and Nazir Citation2018); among those agents, chelating reagents, which can form stable complexes with almost all the heavy metals over a broad pH range (Bilgin and Tulun Citation2016; Sumalatha, Naveen, and Malik Citation2019), present better leaching ability (Liu et al. Citation2018). Because of the toxicity and potential carcinogen of DTA and DTPA (Neilson, Artiola, and Maier Citation2003), EDTA has become an important chelating agent that can detoxify many heavy metals, presenting the following advantages: a low degree of biodegradability in groundwater and in soil, and a high degree of complex formation with respect to heavy metals (Cheikh et al. Citation2010). EDTA disodium (Na2EDTA) is the most utilized kind of EDTA chemicals due to the high solubility. In previous studies, researchers have widely utilized EDTA to extract heavy metals from sludge (Cheikh et al. Citation2010; Gitipour et al. Citation2016), cathode ray tube waste (Pant, Singh, and Upreti Citation2014), contaminated soil (Bilgin and Tulun Citation2016; Voglar and Lestan Citation2014), hazardous fine fraction at an old glass dump (Jani and Hogland Citation2018), and municipal solid waste incineration fly ash (MSWIFA) (Fedje et al. Citation2010; Hong et al. Citation2000; Hong, Tokunaga, and Kajiuchi Citation2000; Janoš et al. Citation2002). EDTA is effective and useful for extraction of heavy metals from MSWIFA, because EDTA could form stable water-soluble complexes with various metal ions (Hong, Tokunaga, and Kajiuchi Citation2000); however, few literature studies have been reported about treating heavy metals in MWIFA with EDTA disodium (Na2EDTA). MSWIFA and MWIFA are all classified as FA; nevertheless, MWIFA contains large amounts of chlorines and carbons, which makes the constituents of MWIFA more complex than MSWIFA, and thus, treatment of MWIFA is also more difficult (Liu et al. Citation2018). Descripted in a latest research literature, an experimental investigation was conducted to reduce heavy metal concentration from MWIFA using ethylene diaminetetra acetic acid disodium (Na2EDTA) as a chelating reagent, the heavy metals removal results showed that Na2EDTA was effective to reduce heavy metals concentration (Ababneh et al. Citation2020). Based on the above-mentioned research results, we can get the significant information that EDTA is an effective and efficient chelating reagent which can be applied to recover heavy metals from MWIFA. Chelating reagents present better leaching ability, chelated metals are highly stable under wide range of pH conditions, and the removal of chelated metals using most existing technologies is difficult (Strawn and Baker Citation2009; Zhu et al. Citation2019); however, they are more harmful to the environment (Liu et al. Citation2018). Treatment of heavy metal-complex has become a critical issue in environmental protection; the main problems faced in the treatment of chelated heavy metals are how to effectively eliminate toxic heavy metals and how to safely dispose of chelating agents (Zhu et al. Citation2019).
In a latest review regarding removal of chelated heavy metals from aqueous solution, different methods to remove chelated heavy metals from wastewater, such as electrolysis, membrane separation, replacement-coprecipitation, adsorption, titanium oxide (TiO2) photocatalysis, and fenton-like oxidation, are discussed in detail for the first time, along with their detailed mechanisms (Zhu et al. Citation2019).
In recent years, the use of ZVI for the treatment of toxic contaminants in groundwater and wastewater has received wide attention, and encouraging treatment efficiencies have been documented (Fu, Dionysiou, and Liu Citation2014). As a readily available, inexpensive, nontoxic, abundant, cheap, and moderately strong reducing agent, ZVI has been regarded as a potential candidate in the field of contaminated groundwater remediation and wastewater treatment (Fu, Dionysiou, and Liu Citation2014; Guan et al. Citation2015b).
ZVI is a reactive metal with standard redox potential (E0 = −0.44 V) and effective reductant when reacting with oxidized contaminants (Fu, Dionysiou, and Liu Citation2014). Removal mechanism of contaminants by ZVI concerns the directional transfer of electrons from ZVI to the contaminants, which transforms the latter into nontoxic or less toxic species (Fu, Dionysiou, and Liu Citation2014). Removal efficiencies of EDTA-chelated heavy-metal ions mainly depend on the reactivity of ZVI, easy surface passivation, and particle aggregation, which significantly limit the effectiveness and practical application of NZVI (Lv et al. Citation2019). In addition, it can have an adverse effect on the ecosystem by releasing NZVI into the environment. In order to solve these shortcomings, this study uses irregular spiral iron scraps. Although the specific surface area of the iron filings is not as large as NZVI, and the reaction activity is not as high as NZVI, it overcomes some of its disadvantages. It is cheap, easy to obtain, and large in quantity, which greatly improve the practicality of iron filings.
In this study, column leaching experiments were conducted to understand the removal efficiencies of heavy metals from MWIFA using Na2EDTA. After being treated, the toxicity analysis of MWIFA residues was conducted by TCLP test to assess risk of MWIFA residues. Iron-column with ZVI was utilized to recover heavy metals from heavy metal-EDTA complexes in waste Na2EDTA solution after MWIFA-column experiments. Na2EDTA in the iron column effluent was recycled to treat MWIFA, and the effectiveness of recovered Na2EDTA was also assessed.
Materials and methods
Materials
MWIFA used in this experiment was obtained from a medical waste incineration plant in Zhengzhou city, Henan province, China. A random method was applied, sample-collecting period lasted one month, and approximately 5 kg fresh MWI sample was withdrawn from storage location in the incineration plant; the subsequent complete mixture of MWIFA was conducted to ensure the representativeness and homogeneity of sample. Before experiment, all raw materials are dried in the oven at 105°C up to constant weight. MWIFA samples were mixed evenly and sealed in a dry sealed container after being sieved by a 2 mm screen.
Total heavy metals analysis
A homogenized dry sample of 0.100 g was weighed and then put in a Teflon digestion tank, digested via a microwave digestion unit for 2 h at 200°C after adding 10 mL HNO3, 6 mL HF, and 2 mL HClO4. After digestion, the sample was then placed on a hot plate at 140°C to heat to nearly 1 mL. After cooled to room temperature, the solution was diluted to a volume of 50 mL with deionized distilled water after being filtered through 0.45 µm cellulose acetate filter membrane. Concentrations of Cu, Pb, Zn, Cd, and Ni in the digests were then determined by inductively coupled plasma atomic emission spectrometry (ICP-MS).
Column experiments
Schematic diagram of continuous column experiments was shown in . Column experiments were performed at a plexiglass cylinder. Column was successively packed with quartz sand (2 mm thick), MWIFA (30.00 g), fine quartz sand (above MWIFA, 2 cm below the water outlet) and thick quartz sand (2 cm thick, to the top of the MWIFA column) from water inlet to water outlet. Prepared 500 mL different concentrations of Na2EDTA (0, 0.02, 0.05, 0.08, 0.1, 0.2 mol/L), and the Na2EDTA solutions were introduced into MWIFA columns by the peristaltic pump with a controlled flow rate of 2 mL/min, respectively. The pH values of collected effluent were measured by pH meter, and the total solution was sampled at 0, 5, 10, 20, 30, 45, 60, 75, 90, 105, 120, and150 min. Then each obtained sample was measured by ICP-MS after being acidified.
According to Rathor, Chopra, and Adhikari (Citation2017), the removal efficiencies of heavy metals from MWIFA were calculated using EquationEquation (1)(1)
(1) :
where R is the removal efficiency, and C and C0 (mg/kg) are the initial and final concentration of the heavy metal in MWIFA.
The 90 g iron filings were put into a homogenous solution of ethanol and water with a volume ratio of 1:1 after being treated with a weak acid, and then heated to 50°C in a water bath for 2 h and then dried with nitrogen. Collected solution obtained after MWIFA-column using different concentrations of Na2EDTA as extracting solution was passed through a column containing treated iron filings (iron-column) by using a peristaltic pump, and the effluent was tapped with a beaker. And at 0, 5, 10, 20, 30, 45, 60, 75, 90, 105, 120, 150 min, the experiment was performed according to the procedure of the previous step. Two parallel experiments were performed during the experiment to ensure the effectiveness of the experiment.
Toxicity characteristic leaching procedure (TCLP)
A toxicity characteristic leaching procedure (TCLP) test was conducted in accordance with the US Environmental Protection Agency (USEPA) Method 1311 to evaluate the leaching toxicity of solid wastes (Lu, Hsu, and Lin Citation2019). The extraction fluid (solution # 1 pH 4.93 ± 0.05 and solution # 2 pH 2.64 ± 0.05) of the sample was determined by a preliminary experimental method. The solid-to-liquid ratio was fixed at 1:20. The extraction was performed using capped polypropylene (PP) bottles loaded on a rotary tumbler at 30 ± 2 rpm for 18 h ± 2 h. After extraction, the mixture was centrifuged by high-speed centrifuge and filtrated through 0.45 µm cellulose acetate filter membrane prior to metal measurement. Leachate was acidified before being analyzed by ICP-MS. All TCLP experiments were carried out in duplicate to ensure the validity of the experiment.
Recovery of Na2EDTA
NaOH solution (1.0 mol/L) was added dropwise to the solution obtained after the column experiment until the pH exceeded 13.0; static settlement of 5 h was utilized to ensure a complete precipitation (solid substance Ⅰ). The precipitation (solid substance Ⅰ) was separated via centrifugation at 3,000 rpm and dried at 60°C. The pH of the homogeneous mixture of all the collected solution (solution Ⅰ) after solid-liquid separation was adjusted to less than 1 for formation of white precipitate with HCl solution (12.0 mol/L). Static settlement of 5 h was utilized to ensure a complete precipitation (solid substance Ⅱ) in the same way. Solid substance Ⅱ was separated via centrifugation at 3,000 rpm and dried at 60°C. Homogeneous mixture of all the collected solution (solution Ⅱ) after solid-liquid separation would be utilized in the subsequent experiment. Precipitation Ⅱ was dissolved in NaOH solution (1.0 mol/L) to form a new solution (solution III). White solid (noted as solid substance III) was obtained after the evaporation of new solution (solution III) in 80°C water bath. Solution Ⅱ was adjusted to pH = 7 using 1 mol/L HCl then distilled to collect the resulting white solid (noted as solid substance Ⅳ) and water. Three kinds of solid substance (Ⅱ, III, Ⅳ) were detected by X-ray powder diffraction (XRD), and the concentration of heavy metals in the final solution was determined by ICP-MS. All the above experiments were performed duplicate for data analysis to ensure the validity of the experiment.
Assessment of recycled Na2EDTA
Successive batch experiments were also conducted to evaluate industrialization potential and reusable times recycled Na2EDTA. Removal efficiencies of heavy metals from MWIFA at different recirculation cycles using recycled Na2EDTA (new Na2EDTA was defined as cycle 0) were assessed using EquationEquation (2)(2)
(2) :
where Pi (P is abbreviation for percentage) is defined as the extraction efficiency at different recirculation cycles using recycled Na2EDTA (new Na2EDTA is defined as cycle 0), R is the removal efficiency, and R0 and Ri(%) are initial removal efficiency using new Na2EDTA and removal efficiency of the heavy metals from MWIFA at different recirculation cycles using recycled Na2EDTA (new Na2EDTA was defined as cycle 0). The i is the serial number of recirculation cycles(0, 1, 2, 3, 4 in this experiment).
Characterizations and measurements
All solutions were filtered through 0.45 µm cellulose acetate filter membrane and diluted with 3% HNO3 (wt%). Cu, Pb, Zn, Cd, and Ni in solution were quantitatively analyzed by the ICP-MS analysis. Mineralogical properties were conducted by X-ray powder diffraction (UltimaIV Series, Rigaku, Japan) using CuKα radiation and identified from the JCPDS-ICDD databases. The voltage and current with 40 kV and 40 mA are adopted during the measurement, respectively. The measurement was carried out in the 2θ range of 10–50° with the speed of 10° per minute, XRD scans run at 0.02° per step.
Results and discussion
Total concentration of heavy metals
showed the concentration of five heavy metals in MWIFA sample and soil background values in China. Concentrations of Cu, Pb, Zn, Cd, and Ni in MWIFA sample were 6067.2 ± 422.3 mg/kg, 2219.7 ± 20.6 mg/kg, 24,252.5 ± 416.4 mg/kg, 60.3 ± 12.1 mg/kg, and 227.1 ± 17.8 mg/kg, respectively. Generally, heavy metals concentrations in MSWFA sample far exceeded the corresponding soil background values in China, which made them a potentially hazardous source of environmental pollution. Moreover, Cu, Pb, Zn, Cd, and Ni had been proved to possess high toxicity to the ecosystem, and MWIFA is classified as hazardous waste (Liu et al. Citation2018; Xie and Zhu Citation2013).
Table 1. Heavy metal concentrations in the fly ash sample and soil background value in China (mg/kg dry weight).
Heavy metals removal and leaching behavior of MWIFA residue
Batch experiments were conducted to elucidate the effects of Na2EDTA concentration on removal efficiencies of Cu, Pb, Zn, Cd, and Ni from MWIFA at different concentrations of Na2EDTA solution. The results of this experiment were shown in ; we can conclude that Na2EDTA was also effective in solubilizing Cu, Pb, Zn, Cd, and Ni from MWIFA due to the high stability constants of Na2EDTA with heavy metals, and this conclusion was similar with the results descripted in previous literature (Hong, Tokunaga, and Kajiuchi Citation2000). In our research, removal efficiency of heavy metals increased with simultaneous increase of Na2EDTA concentration. When Na2EDTA concentration increased from 0.00 to 0.20 mol/L, removal efficiencies of Cu, Pb, Zn, Cd, and Ni increased from 0.5% to 69.7%, 1.9% to 72.7%, 1.0% to 66.5%, 5.8% to 78.1%, and 2.9% to 52.3%, respectively. The removal efficiencies of Cu, Pb, Zn, Cd, and Ni in our research were close to the results in the previous study (Hong, Tokunaga, and Kajiuchi Citation2000).
US EPA TCLP test was a frequently-used method to evaluate the leaching toxicity of heavy metals in solid wastes. shows the leaching concentrations of Cu, Pb, Zn, Cd, and Ni from MWIFA sample and MWIFA residue obtained after heavy metals extraction using Na2EDTA by TCLP. EPA TCLP regulatory standards were applied for Pb and Cd; however, there were no standard limits for Zn, Cu, and Ni in EPA TCLP regulatory standards, and thus, Chinese regulatory levels of national standard GB 5085.3–2007 were employed as supplementary reference standard in the study. As shown in , although the TCLP leaching amounts of Cu, Pb, Zn, Cd, and Ni was smaller than the regulatory limits when the concentration of Na2EDTA was 0.1 mol/L, TCLP leaching amount of Zn was 85.8 ± 3.0 mg/L, which was close to 100 mg/L of its regulatory limit value. Therefore, the concentration of Na2EDTA further increased. After washed by 0.2 mol/L Na2EDTA solution, TCLP leaching values of Cu, Pb, Zn, Cd, and Ni were the lowest and satisfied the standard (GB 5085.3–2007, Identification standards for hazardous wastes–-Identification for extraction toxicity), and the leaching values were 58.4 ± 2.0 mg/L, 2.81 ± 0.14 mg/L, 64.3 ± 4.0 mg/L, 0.156 ± 0.005 mg/L, 0.381 ± 0.006 mg/L. Combining removal efficiency of heavy metal with TCLP leaching, 0.2 mol/L was chosen as the optimal concentration for removal of heavy metals, also the suitable concentration to realize the TCLP leaching amounts of Cu, Pb, Zn, Cd, and Ni all far less than the standard limits.
Heavy metal removal from leachate with zero-valent iron (ZVI)
EDTA-chelated MⅡ (M refers to divalent metal) could be removed by ZVI, EDTA-chelated CuII was taken for example to elucidate the removal mechanism. In theory, the EDTA-chelated CuII was first replaced by FeIII ions, which was originated from the oxidation of Fe0 (Liu et al. Citation2017a). EDTA-chelated CuII could not be reduced by Fe0 during Fe0 corrosion (Ku and Chen Citation1992); the primary product, FeⅡ, may stay at the interface, or may be transported away from the surface of Fe0, where it was subjected to homogeneous oxidation to FeIII by dissolved oxygen (DO) (Guan et al. Citation2015a). The pH values of MWIFA-column effluent (also the influent of iron-column) are shown in ; acid environment of the solution facilitated the formation of FeⅡfrom Fe0. The stability constant of FeIII-EDTA (log K = 25.2) was remarkably higher than CuII-EDTA (log K = 18.8) (Liu et al. Citation2017a). Then, the released free CuII ions were further removed through multiple pathways, including precipitation, adsorption onto the surface of ZVI corrosion products, and reduction by ZVI (Karabelli et al. Citation2008; Jiang et al. Citation2015). Some reaction of the above process are described as EquationEquations (3)(3)
(3) –(Equation7
(7)
(7) ) (Guan et al. Citation2015a; Ju and Hu Citation2011; Jiang et al. Citation2010):
Stability constant of FeIII-EDTA (logK = 25.2) (Liu et al. Citation2017a; Zhu et al. Citation2019) was also significantly higher than PbII-EDTA (log K = 17.9) (Neilson, Artiola, and Maier Citation2003), ZnII-EDTA (log K = 16.4) (Neilson, Artiola, and Maier Citation2003), CdII-EDTA (log K = 16.4) (Neilson, Artiola, and Maier Citation2003), and NiII-EDTA (log K = 18.61) (Kornev et al. Citation2017), so FeIII could replace the heavy metals in these five heavy metal-EDTA complexes, which achieved the decomplexation effect of ZVI on heavy metal-EDTA complexes.
ZVI in the iron-column first produced FeII ions, FeII ions were easily oxidized to FeIII ions. In the above process, OH− will be generated. The production of OH− increased the pH of iron-column effluent. Then, the released free CuII, PbII, ZnII, CdII, and NiII ions reacted with the OH− generated continuously in the above process to form hydroxide the pH value of the solution declined, Fe(OH)2 and Fe(OH)3 colloids produced during the reaction. Otherwise, the surface of the ZVI corrosion production could also adsorb Cu, Pb, Zn, Cd, and Ni particles. These could decrease concentrations of Cu, Pb, Zn, Cd, and Ni in the waste liquid, which was also consistent with . Changes of Cu, Pb, Zn, and Ni in iron-column influent and effluent are shown in . The concentrations of Cu, Pb, Zn, Cd, and Ni in iron-column influent and effluent were 242 ± 18 mg/L, 82.3 ± 8.0 mg/L, 765 ± 16 mg/L, 1.24 ± 0.03 mg/L, 2.16 ± 0.09 mg/L, and 0.785 ± 0.040 mg/L, 6.93 ± 0.10 mg/L, 64.6 ± 6.0 mg/L, 0.305 ± 0.030 mg/L, and 0.524 ± 0.005 mg/L, respectively. The concentrations of the five heavy metals decreased by 99.7%, 91.6%, 91.6%, 75.4%, and 75.7%, respectively.
Recovery of Na2EDTA and reuse of the recovered Na2EDTA solution
XRD was employed for qualitative analysis of solid substanceⅡ, III, and Ⅳ using EDTA, Na2EDTA and NaCl as reference. One aim of the experiment was to recover EDTA, Na2EDTA, and NaCl; solid substance Ⅱ, III, and Ⅳ were noted as recycled EDTA, recycled Na2EDTA, and recycled NaCl, respectively. XRD patterns of recycled EDTA, recycled Na2EDTA, new EDTA, and Na2EDTA are shown in . Although there were some miscellaneous peaks in recycled EDTA and recycled Na2EDTA, they were basically consistent with the EDTA and Na2EDTA peaks. Combination of the abovementioned two results proved that EDTA and Na2EDTA were the main products of the recovery. As shown in , XRD pattern of solid substance Ⅳ exactly matched XRD pattern of the new NaCl. Therefore, NaCl was considered to be the main component of solid substance Ⅳ.
Recycled Na2EDTA was used to remove Cu, Pb, Zn, Cd, and Ni from the original MWIFA; the results are shown in . The removal rates of Cu, Pb, Zn, Cd, and Ni were 67.1%, 68.8%, 63.2%, 73.9%, and 50.7%, respectively. Compared with the removal of Cu, Pb, Zn, Cd, and Ni with the new Na2EDTA, the removal rates using recovered Na2EDTA decreased by 2.6%, 3.9%, 3.3%, 4.2%, and 1.6%, respectively. Recycled Na2EDTA also possessed the high removal efficiency of heavy metal from MWIFA. This circulating system possessed industrialization potential for MWIFA disposal to simultaneously realize removal of heavy metals, cyclic utilization of Na2EDTA, and extraction of NaCl. The concentrations of Cu, Pb, Zn, Cd, and Ni in the final recovered water, discharge standard of pollutants for municipal wastewater treatment plant (GB18918-2002) and class V water standard of surface water environmental quality standard (GB3838-2002) were shown in . The concentrations of Cu, Pb, Zn, Cd, and Ni in the recovered water were 0.166 mg/L, 0 mg/L, 0.431 mg/L, 0.00521 mg/L, and 0.0165 mg/L, respectively, which were lower than those limits in the two standards.
Table 2. Comparison of heavy metal concentrations in recycled water and two standards.
Figure 8. Removal efficiencies of Cu, Pb, Zn, Cd, and Ni from MWIFA using new Na2EDTA and recycled Na2EDTA solution.
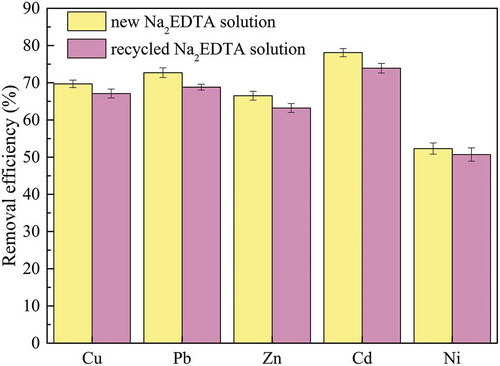
Based on the results noted above, conclusions were drawn that Na2EDTA could be recycled and reutilized for recovery of heavy metals from MWIFA. Besides, reusable times for the recycled Na2EDTA would be significant information to assess the industrialization potential. Furthermore, successive batch experiments were also conducted for that evaluation, and the results are shown in . Extraction efficiency by recycled Na2EDTA decreased gradually with simultaneous increase of Na2EDTA cycles. After 4 recirculation cycles, extraction efficiencies of Pb and Cd (removal efficiency at different cycles divided by removal efficiency of new Na2EDTA) declined toward 80%, more recirculation cycles more extraction efficiencies attenuation, increased cycles would decrease the extraction efficiency below 80% (especially Pb and Cd).
Conclusion
Na2EDTA was used to remove Cu, Pb, Zn, Cd, and Ni from MWIFA and possessed obvious removal effect. TCLP leaching values of the five heavy metals in MWIFA residue after washing with 0.2 mol/L Na2EDTA solution all lowered standard values, TCLP leaching values of Cu, Pb, Zn, Cd, and Ni were the lowest and satisfied the standard, and the leaching values were 58.4 ± 2.0 mg/L, 2.81 ± 0.14 mg/L, 64.3 ± 4.0 mg/L, 0.156 ± 0.005 mg/L, 0.381 ± 0.006 mg/L, removal efficiencies of Cu, Pb, Zn, Cd, and Ni were 69.7%, 72.7%, 66.5%, 78.1%, and 52.3%, respectively.
Economical and practical ZVI (iron filings) was used for decomplexing the complexes. Concentrations of Cu, Pb, Zn, Cd, and Ni in the iron-column effluent (relative to the iron-column influent) were reduced by 99.7%, 91.6%, 91.6%, 75.4%, and 75.7%, respectively.
In order to reduce the consumption of Na2EDTA, the possibility of recycling Na2EDTA from iron-column effluent was realized. In the process of regulating pH and evaporation, EDTA, NaCl, and water can be recovered from iron-column effluent. Recycled water reached the discharge standard of pollutants for municipal wastewater treatment plant (GB18918-2002) and the class V water standard of surface water environmental quality standard (GB3838-2002).
Recycled Na2EDTA possessed the high removal efficiency of heavy metal from MWIFA compared with the removal of Cu, Pb, Zn, Cd, and Ni with the new Na2EDTA, removal efficiencies using recovered Na2EDTA decreased by 2.6%, 3.9%, 3.3%, 4.2%, and 1.6%, respectively.
Successive batch experiments were also conducted to evaluate industrialization potential and reusable times for the recycled Na2EDTA. After four recirculation cycles, extraction efficiencies of Pb and Cd (removal efficiency at different cycles divided by removal efficiency of new Na2EDTA) declined toward 80%, more recirculation cycles, more extraction efficiencies attenuation.
Acknowledgments
The authors are grateful for the administrative support from Wei-Min Liu and the technical support from Fei-Hua Yang. The authors are all grateful for the special support from the graduate student of Dr. Chun-Feng Wang.
Disclosure statement
No potential conflict of interest was reported by the authors.
Additional information
Funding
References
- Ababneh, A., R. Al-Rousan, W. Gharaibeh, and M. Abu-Dalo. 2020. Recycling of pre-treated medical waste fly ash in mortar mixtures. J. Mater. Cycles Waste Manag. 22(1):207–20.
- Alvim-Ferraz, M. C. M., and S. A. V. Afonso. 2005. Incineration of healthcare wastes: Management of atmospheric emissions through waste segregation. Waste Manag. 25 (6):638–48. doi:10.1016/j.wasman.2004.07.017.
- Bilgin, M., and S. Tulun. 2016. Removal of heavy metals (Cu, Cd and Zn) from contaminated soils using EDTA and FeCl3. Glob. Nest. J. 18 (1):98–107.
- Cheikh, M., J. P. Magnin, N. Gondrexon, J. Willison, and A. Hassen. 2010. Zinc and lead leaching from contaminated industrial waste sludges using coupled processes. Environ. Technol. 31 (14):1577–85. doi:10.1080/09593331003801548.
- Cozea, A., E. Bucur, C. B. Lehr, L. F. Pascu, and G. Tanase. 2018. Aspects regarding the use of some species of plants as bioindicators in air quality assessment. Rev. Chim. 69 (11):4138–40.
- Deliyanni, E. A., G. Z. Kyzas, and K. A. Matis. 2017. Various flotation techniques for metal ions removal. J. Mol. Liq. 225:260–64.
- Fedje, K. K., C. Ekberg, G. Skarnemark, and B.-M. Steenari. 2010. Removal of hazardous metals from MSW fly ash-An evaluation of ash leaching methods. J. Hazard. Mater. 173 (1–3):310–17. doi:10.1016/j.jhazmat.2009.08.094.
- Fu, F., D. D. Dionysiou, and H. Liu. 2014. The use of zero-valent iron for groundwater remediation and wastewater treatment: A review. J. Hazard. Mater. 267:194–205. doi:10.1016/j.jhazmat.2013.12.062.
- Gitipour, S., S. Ahmadi, E. Madadian, and M. Ardestani. 2016. Soil washing of chromium- and cadmium-contaminated sludge using acids and ethylenediaminetetra acetic acid chelating agent. Environ. Technol. 37 (1):145–51. doi:10.1080/09593330.2011.597784.
- Guan, X., X. Jiang, J. Qiao, and G. Zhou. 2015a. Decomplexation and subsequent reductive removal of EDTA-chelated CuII by zero-valent iron coupled with a weak magnetic field: Performances and mechanisms. J. Hazard. Mater. 300:688–94. doi:10.1016/j.jhazmat.2015.07.070.
- Guan, X., Y. Sun, H. Qin, J. Li, I. M. C. Lo, D. He, and H. Dong. 2015b. The limitations of applying zero-valent iron technology in contaminants sequestration and the corresponding countermeasures: The development in zero-valent iron technology in the last two decades (1994–2014). Water Res. 75:224–48. doi:10.1016/j.watres.2015.02.034.
- Hong, K. J., S. Tokunaga, Y. Ishigami, and T. Kajiuchi. 2000. Extraction of heavy metals from MSW incinerator fly ash using saponins. Chemosphere 41 (3):345–52. doi:10.1016/s0045-6535(99)00489-0.
- Hong, K. J., S. Tokunaga, and T. Kajiuchi. 2000. Extraction of heavy metals from MSW incinerator fly ashes by chelating agents. J. Hazard. Mater. 75 (1):57–73. doi:10.1016/s0304-3894(00)00171-0.
- Jani, Y., and W. Hogland. 2018. Chemical extraction of trace elements from hazardous fine fraction at an old glasswork dump. Chemosphere 195:825–30. doi:10.1016/j.chemosphere.2017.12.142.
- Janoš, P., M. Wildnerová, and T. Loučka. 2002. Leaching of metals from fly ashes in the presence of complexing agents. Waste Manag. 22 (7):783–89. doi:10.1016/s0956-053x(02)00039-9.
- Jiang, S., J. Qu, and Y. Xiong. 2010. Removal of chelated copper from wastewaters by Fe 2+-based replacement–precipitation. Environ. Chem. Lett. 8 (4):339–42.
- Jiang, X., J. Qiao, I. M. C. Lo, L. Wang, X. Guan, Z. Lu, G. Zhou, and C. Xu. 2015. Enhanced paramagnetic Cu2+ ions removal by coupling a weak magnetic field with zero valent iron. J. Hazard. Mater. 283:880–87. doi:10.1016/j.jhazmat.2014.10.044.
- Ju, F., and Y. Hu. 2011. Removal of EDTA-chelated copper from aqueous solution by interior microelectrolysis. Sep. Purif. Technol. 78 (1):33–41.
- Karabelli, D., Ç. Üzüm, T. Shahwan, A. E. Eroğlu, T. B. Scott, K. R. Hallam, and I. Lieberwirth. 2008. Batch removal of aqueous Cu2+ ions using nanoparticles of zero-valent iron: A study of the capacity and mechanism of uptake. Ind. Eng. Chem. Res. 47 (14):4758–64.
- Kim, L., G. Traistaru, B. Stanescu, L. F. Pascu, T. Gheorghita, and D. Manolache. 2019. The chemical fractions and leaching of heavy metals in ash from medical waste incineration using two different sequential extraction procedures. Rev. Chim. 70 (1):269–74.
- Kornev, V., G. Alabdulla, T. Kropacheva, and E. Batueva. 2017. Formation of heteropolynuclear Cobalt(II) and Nickel(II) complexonates with EDTA and 2-aminopropanoic acid. Russ. J. Inorg. Chem. 62 (5):659–64.
- Kougemitrou, I., A. Godelitsas, C. Tsabaris, V. Stathopoulos, A. Papandreou, P. Gamaletsos, G. Economou, and D. Papadopoulos. 2011. Characterisation and management of ash produced in the hospital waste incinerator of Athens, Greece. J. Hazard. Mater. 187 (1):421–32. doi:10.1016/j.jhazmat.2011.01.045.
- Ku, Y., and C. H. Chen. 1992. Removal of chelated copper from wastewaters by iron cementation. Ind. Eng. Chem. Res. 31 (4):1111–15.
- Liu, F., H.-Q. Liu, G.-X. Wei, R. Zhang, G.-S. Liu, J.-H. Zhou, and -T.-T. Zeng. 2019. Detoxification of medical waste incinerator fly ash through successive flotation. Sep. Sci. Technol. 54 (1):163–72.
- Liu, F., H.-Q. Liu, G.-X. Wei, R. Zhang, -T.-T. Zeng, G.-S. Liu, and J.-H. Zhou. 2018. Characteristics and treatment methods of medical waste incinerator fly ash: A review. Processes 6 (10):25.
- Liu, F., C. Shan, X. Zhang, Y. Zhang, W. Zhang, and B. Pan. 2017a. Enhanced removal of EDTA-chelated Cu(II) by polymeric anion-exchanger supported nanoscale zero-valent iron. J. Hazard. Mater. 321:290–98. doi:10.1016/j.jhazmat.2016.09.022.
- Liu, H.-Q., F. Liu, G.-X. Wei, R. Zhang, and -D.-D. Zang. 2017b. Two-step flotation treatment for removal of toxic matter from hospital solid waste incinerator fly ash. Aerosol Air Qual. Res. 17 (5):1329–40.
- Lu, -C.-C., M. H. Hsu, and Y.-P. Lin. 2019. Evaluation of heavy metal leachability of incinerating recycled aggregate and solidification/stabilization products for construction reuse using TCLP, multi-final pH and EDTA-mediated TCLP leaching tests. J. Hazard. Mater. 368:336–44. doi:10.1016/j.jhazmat.2019.01.066.
- Lv, X., X. Qin, K. Wang, Y. Peng, P. Wang, and G. Jiang. 2019. Nanoscale zero valent iron supported on MgAl-LDH-decorated reduced graphene oxide: Enhanced performance in Cr(VI) removal, mechanism and regeneration. J. Hazard. Mater. 373:176–86. doi:10.1016/j.jhazmat.2019.03.091.
- Mangialardi, T. 2004. Effects of a washing pre-treatment of municipal solid waste incineration fly ash on the hydration behaviour and properties of ash-Portland cement mixtures. Adv. Cem. Res. 16 (2):45–54.
- Meer, I., and R. Nazir. 2018. Removal techniques for heavy metals from fly ash. J. Mater. Cycles Waste Manag. 20 (2):703–22.
- Necsulescu, C., L. Ionita, and E. Bucur. 2008. Stationary sources emissions. Total Cd, Cr, Cu determination in exhaust gases from incinerators. J. Environ. Prot. Ecol. 9 (1):1–14.
- Neilson, J. W., J. F. Artiola, and R. M. Maier. 2003. Characterization of lead removal from contaminated soils by nontoxic soil-washing agents. J. Environ. Qual. 32 (3):899–908. doi:10.2134/jeq2003.8990.
- Pant, D., P. Singh, and M. K. Upreti. 2014. Metal leaching from cathode ray tube waste using combination of Serratia plymuthica and EDTA. Hydrometallurgy 146:89–95.
- Papamarkou, S., C. Sifaki, P. E. Tsakiridis, G. Bartzas, and K. Tsakalakis. 2018. Synthetic wollastonitic glass ceramics derived from recycled glass and medical waste incinerator fly ash. J. Environ. Chem. Eng. 6 (5):5812–19.
- Rathor, G., N. Chopra, and T. Adhikari. 2017. Remediation of nickel ion from soil and water using nano particles of Zero-Valent Iron (nZVI). Orient. J. Chem. 33 (2):1025–29.
- Sabiha, J., M. Tufail, and S. Khalid. 2008. Heavy metal pollution from medical waste incineration at Islamabad and Rawalpindi, Pakistan. Microchem. J. 90 (1):77–81. doi:10.1016/j.microc.2008.03.010.
- Shaaban, A. F. 2007. Process engineering design of pathological waste incinerator with an integrated combustion gases treatment unit. J. Hazard. Mater. 145 (1):195–202. doi:10.1016/j.jhazmat.2006.11.013.
- Strawn, D. G., and L. L. Baker. 2009. Molecular characterization of copper in soils using X-ray absorption spectroscopy. Environ. Pollut. 157 (10):2813–21. doi:10.1016/j.envpol.2009.04.018.
- Sumalatha, J., B. P. Naveen, and R. K. Malik. 2019. Efficiency of washing techniques for removal of heavy metals from industrial sludge. Pollution 5 (1):189–98.
- Tsakalou, C., S. Papamarkou, P. E. Tsakiridis, G. Bartzas, and K. Tsakalakis. 2018. Characterization and leachability evaluation of medical wastes incineration fly and bottom ashes and their vitrification outgrowths. J. Environ. Chem. Eng. 6 (1):367–76.
- Voglar, D., and D. Lestan. 2014. Chelant soil-washing technology for metal-contaminated soil. Environ. Technol. 35 (11):1389–400. doi:10.1080/09593330.2013.869265.
- Wang, C., N. Zhu, Y. Wang, and F. Zhang. 2012. Co-detoxification of transformer oil-contained PCBs and heavy metals in medical waste incinerator fly ash under sub- and supercritical water. Environ. Sci. Technol. 46 (2):1003–09. doi:10.1021/es202342h.
- Xie, Y., and J. Zhu. 2013. Leaching toxicity and heavy metal bioavailability of medical waste incineration fly ash. J. Mater. Cycles Waste Manag. 15 (4):440–48.
- Yang, S., A. Saffarzadeh, T. Shimaoka, and T. Kawano. 2014. Existence of Cl in municipal solid waste incineration bottom ash and dechlorination effect of thermal treatment. J. Hazard. Mater. 267:214–20. doi:10.1016/j.jhazmat.2013.12.045.
- Zhao, L., F.-S. Zhang, K. Wang, and J. Zhu. 2009. Chemical properties of heavy metals in typical hospital waste incinerator ashes in China. Waste Manag. 29 (3):1114–21. doi:10.1016/j.wasman.2008.09.003.
- Zhu, Y., W. Fan, T. Zhou, and X. Li. 2019. Removal of chelated heavy metals from aqueous solution: A review of current methods and mechanisms. Sci. Total Environ. 678:253–66. doi:10.1016/j.scitotenv.2019.04.416.