ABSTRACT
Recycled manure solids (RMS) produced in dairy farms from fresh manure need to be sanitized before using them as bedding material. However, the impact on air quality of composting RMS remains unknown. Four RMS composting methods were tested during a 10-day aging of piles in experimental chambers: static windrow (SW), turned windrow (TW), SW following drum composting for 24 h (DC24) or SW following drum composting for 72 h (DC72). Air samples were collected using a SASS®3100 Dry Air Sampler on days 0 (pilling of the RMS), 5, and 10. Bacteria (16S rRNA genes), Penicillium/Aspergillus, A fumigatus, and 11 human pathogenic bacteria (e.g. Klebsiella pneumonia) were quantified by qPCR while endotoxins and dust particles were, respectively, measured by LAL assays and with a DustTrakTM DRX Aerosol Monitor. On day 0, RMS produced by SW and TW yielded the lowest concentrations of airborne bacteria, while DC24 resulted in the lowest levels of Penicillium/Aspergillus and dust particles. SW method led on day 5 to the lowest concentration of bacteria and Penicillium/Aspergillus, and DC24 and DC72 to the lowest concentration of airborne dust. On day 10, SW and TW piles were associated with the lowest levels of Penicillium/Aspergillus and dust particles. A significant difference was observed between concentration of airborne bacteria, Penicillium/Aspergillus and endotoxins before and during the turnover of TW piles. None of the studied human pathogens was detected in the air samples. Results of the present study suggest that SW and TW are the most promising methods for the production of composted RMS with respect to microbial air quality. However, the experimental chambers do not accurately represent commercial dairy barns and further research on these composting methods is necessary. Finally, the study highlights that bedding material and its management may be determinant factors for air quality in dairy barns.
Implications: The research evaluated the impact on microbial air quality of composting recycled manure solids (RMS) produced from fresh cow manure. RMS need to be composted or sanitized before using them as bedding material for animals. The impact on animal health of RMS still needs to be confirmed, while the effect on air quality and the health of dairy farmers is unknown. In the present study, microbial air quality associated with four RMS composting methods was investigated. Data revealed that two methods resulted in lower aerosolization of dust particles, endotoxins, molds, and bacteria.
Introduction
Since bedding materials such as straw, sawdust, and sand are expensive, Canadian dairy producers are looking for alternative materials such as recycled manure solids (RMS) (Husfeldt et al. Citation2012). RMS are produced by the solid-liquid separation of fresh cow manure and composed of excreted undigested fibers (Menear and Smith Citation1973). In Canadian dairy farms, RMS are composted (e.g., static or turned piles for days) or use directly as bedding without any sanitation measures. Producers’ decisions are currently often directed and influenced by the farm equipment industry. However, RMS represent a possible health risk for both animals and humans. RMS may be a reservoir of pathogens in dairy barns resulting in spreading infections among the herd. The impact on animal health of RMS in barns still needs to be confirmed, while the effect on air quality and the health of dairy farmers has never been evaluated (Harrison, Bonhotal, and Shwarz Citation2008; Husfeldt et al. Citation2012). Long-term and frequent exposure to the high concentration of bioaerosols are however known to put farmers at risk of developing respiratory disorders (Donham Citation1986; Walser et al. Citation2015).
Bioaerosols are composed of airborne microorganisms (alive or dead), microbial structural components (e.g., endotoxins), plant fragments and animal cells, and are mainly produced in dairy barns during the mechanical disturbance of cow bedding (Garcia et al. Citation2013; Kullman et al. Citation1998; Schenker et al. Citation1998). A microbial load in RMS higher than in traditional bedding could then be associated with elevated concentrations of bioaerosols and consequent risks for dairy farmers’ respiratory health. Asthma, chronic bronchitis, and extrinsic allergic alveolitis (farmer’s lung disease) have already been reported among dairy farmers (Malmberg Citation1990; Schenker et al. Citation1998) and some of these respiratory problems were associated with an occupational exposure to aerosolized microbes. The actinomycete Saccharopolyspora rectivirgula was identified as an etiologic agent of extrinsic allergic alveolitis (Cormier et al. Citation1986) while the mold Aspergillus fumigatus is responsible for aspergillosis in immunosuppressed individuals (Dagenais and Keller Citation2009; Schenker et al. Citation1998). Both microorganisms may be found airborne and in high concentrations in Canadian dairy farms (Lecours et al. Citation2012; Mbareche et al. Citation2019). Moreover, endotoxins, a wall component of Gram-negative bacteria composing bioaerosols in dairy barns (Boucher et al. Citation2018; Duchaine et al. Citation1999), are linked to immunotoxic responses and reduction of lung functions (Thorn Citation2001). Few exposure limits exist for bioaerosols. A total organic dust threshold limit value (time-weighted average (TLV-TWA)) of 10 mg/m3 is recommended by the Canadian Center for Occupational Health and Safety while in Europe, a health-based recommended occupational exposure limit of 90 EU/m3 for endotoxins has been suggested by the Dutch Expert Committee on Occupational Safety (DECOS Citation2010).
The present study is part of a broader research initiative aiming at evaluating and characterizing different RMS production and composting methods which could help Canadian dairy farmers in adopting a RMS bedding and keeping a healthy herd. The aim of the present study was to evaluate the biological air quality (concentration of bioaerosols) associated with each RMS composting methods, and subsequently to identify the best method with regards to limiting the concentration of bioaerosols and thus, the risk for dairy farmers’ potential exposure. Airborne dust, endotoxins, and human pathogenic agents were sampled and quantified on experimental days 0, 5, and 10 ().
Figure 1. A confined and independently ventilated experimental chamber with a polyethylene drainage pipe on its concrete floor (a) filled with 0.67 m3 of RMS (b). For the turned windrow composting method, RMS were daily turned (c). Airborne dust, endotoxins and microorganisms were sampled with a DustTrakTM DRX Aerosol Monitor (TSI) and a SASS®3100 Dry Air Sampler (Research International) on days 0 (pipe filling with RMS), 5 (5-day aging of RMS) and 10 (10-day aging of RMS) (d)
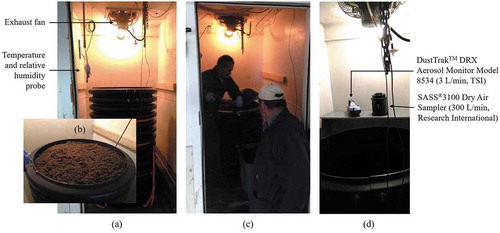
Material and methods
Experimental setup
The experimental setup and design were described previously by Fournel et al. (Fournel et al. Citation2019). Briefly, RMS were produced (solid-liquid separation) for the study with a screw press and composted in pipes in controlled and confined experimental chambers (, 1.2 m width × 2.4 m length × 2.4 m height) at the Research and Development Institute for the Agri-Environment (IRDA, Deschambault, QC, Canada) in July 2017 using: 10 days static windrow, daily turned windrow for 10 days, 10 days static windrow following a 24 h in a drum composter or 10-day static windrow following a 72 h in a drum composter. The fresh manure to be separated came from an in-barn storage pit of a nearby Canadian dairy farm. The temperature, the physicochemical characteristics and the culturable bacterial content of RMS were evaluated during the 10-day composting process as reported by Fournel et al. (Fournel et al. Citation2019).
Each chamber is equipped with a variable-speed exhaust fan. An air conditioning unit or a heating device could be used to cool or warm the air before entering the chambers. Temperature (model CS500) and relative humidity (model CR1000) were measured using a probe suspended from the ceiling (model CS500, Campbell Scientific Canada, Corp., Edmonton, AB, Canada). For the present study, setting temperature was 23°C for all chambers, which represented the average maximum temperature in Deschambault during the summer months of the past 5 yr (Environment Canada Citation2018). Air temperature varied around 23°C for all chambers (22.7–23.3°C) and relative humidity varied from 47% to 67% throughout the 10-day experiment. Ambient air conditions were similar between chambers (P > .05, Fournel et al. Citation2019). Ventilation rates were calculated from differences of pressure across a 204-mm iris orifice damper (model 200, Continental Fan Manufacturing Inc., Buffalo, NY) located inside the exhaust duct of each room. Pressure was measured across the damper each 5 s and the data logger recorded the average each 15 min. During the present study, the ventilation system delivered between 3.7 and 4.0 m3/h of fresh air (Fournel et al. Citation2019).
On the concrete floor of each chamber, a polyethylene drainage pipe (1.5 m deep and 0.75 m in diameter) with a smooth interior wall and a corrugated exterior wall () contained 0.67 m3 of RMS (). Each pipe and its content simulated the middle column of a large compost pile. Four composting approaches were randomly attributed to each of the 10 chambers.
The static windrow (SW) method involved composting using undisturbed RMS piles and the turned windrow (TW) referred to daily manually turned the RMS piles (). The 24 h drum composting method (DC24) involved 10 days aging of undisturbed RMS that were previously drum composted for 24 h or 72 h for the 72 h drum composting method (DC72). A commercial drum composter (1.2 m in diameter × 3 m length; AGF Brome, Cowansville, QC, Canada) was used and equipped with a circular inline duct fan (model CK 4” A, Östberg, Cambridge, ON, Canada) producing a flow up to 60 L/s. The composter turned continuously with a delay of 10 s between each full rotation. RMS were piled (sampling day 0) and aged (sampling days 5 and 10) inside three chambers for the composting of RMS through the SW and TW methods, and two chambers for the DC24 and DC72 methods. On each sampling day, the air was sampled once in each chamber (for each sampling day, n = 3 chambers for SW and TW methods and n = 2 chambers for the DC24 and DC72 methods).
Air sampling methods
Air samples were collected using a SASS® 3100 Dry Air Sampler (Research International, Monroe, WA, USA) on an electrostatic filter at a flow rate of 300 L/min for 10 min. A DustTrakTM DRX Aerosol Monitor (Model 8534, TSI, Shoreview, MN, USA) measured in real-time the concentration in milligrams per cubic meter of air (mg/m3) of total dust particles, PM10, PM2.5, and PM1 at a flow rate of 3 L/min for 10 min. Air samples were collected from each chamber at 2.5 cm (for SW, DC24, and DC72) and 5.1 cm (for TW) above the RMS piles (). As illustrated in and 1d (side-by-side comparison), the air samplers were placed on a shelf near the inhalable zone of a man working on/shoveling the compost pile. The shelf was supported by pieces of wood screwed to the wall (one piece is showed in ). Samples and measurements were collected on day 0, during the loading of RMS into the pipes, as well as on days 5 and 10. For TW composting method, samples were taken before the pile turnover and during the refilling of the pipes on days 5 and 10. On each sampling day, unused SASS® 3100 electrostatic filters were used as field blanks.
Air sample processing
Bioaerosols were extracted from the SASS® 3100 electrostatic filters using a SASS® 3010 Particle Extractor (Research International) and 5 mL of a sterile extraction solution (138 mM sodium chloride, 2.7 mM potassium chloride, 0.05% Triton X-100, <0.1% sodium azide, 10 mM sodium phosphate, pH 7.4). One milliliter of each sample was filtered on an IsoporeTM 0.2 µm pore size polycarbonate membrane filter (Merck Millipore Ltd., Carrigtwohill, COR, Ireland), as described in Mbareche et al. (Mbareche et al. Citation2019). Total DNA from filters was then extracted with a DNeasy® PowerLyzer® PowerSoil® DNA Kit (QIAGEN, Mississauga, ON, Canada).
Airborne endotoxins quantification
Endotoxins were quantified from the extracted SASS® 3100 electrostatic filters. Kinetic-QCLTM Kinetic Chromogenic Limulus Amebocyte Lysate (LAL) Assays (LONZA, Walkersville, MD) were performed on air samples and field blanks according to the manufacturer’s instructions (Boucher et al. Citation2018) using Pasteur oven sterilized borosilicate tubes, gloves, and pyrogen-free laboratory consumables. Inhibition/enhancement tests were performed with air samples and field blanks. Concentration from field blanks was subtracted from those of the air samples. Data were analyzed with Gene5 2.00 software (BioTek, Winooski, NH, USA) and expressed as endotoxin units per cubic meter of air (EU/m3).
Microbial quantification using PCR
A CFX96 or CFX384 Touch™ Real-Time PCR Detection System (Bio-Rad, Hercules, CA, USA) was used to perform quantitative polymerase chain reaction (qPCR). Data were analyzed with the Bio-Rad CFX Manager software (Version 3.1). Standard curves from 1 × 106 to 1 copy of genomic DNA or synthetic plasmid (Integrated DNA Technologies Coralville, IA, USA) and negative controls (no template) were included in each qPCR protocol. All performed qPCR resulted in an amplification efficiency ranging from 90% to 110%. The gene copy numbers were then converted into gene copies per cubic meter of air (copies/m3). Since Escherichia coli genomic DNA was used as the standard curve for the quantification of total bacteria by PCR, the results were expressed in E. coli equivalent genomes per cubic meter of air (E. coli equivalent/m3). Penicillium/Aspergillus concentration was as well expressed in Aspergillus fumigatus equivalent genomes/m3 of air (A. fumigatus equivalent/m3) as standard curves were built with A. fumigatus genomic DNA.
The primer and probe sequences for all the studied microorganisms are listed in and were all purchased from Integrated DNA Technologies. Reagent concentrations and the thermoprotocols used for each qPCR reaction are presented in the supplementary materials (Tables A.1 and A.2, respectively).
Table 1. Primers and probes used for PCR quantification of selected microorganisms
Statistical analysis
Data were expressed as mean ± standard deviation and analyzed using a mixed ANOVA with two fixed factors. One was the day of sampling (days 0, 5, and 10) and was considered to be a repeated measure factor. The other factor was the composting method. The ANOVA was used to determine if there was an interaction between these fixed factors. The chamber was treated as a random effect in the analyses. Multiple statistical models were applied to obtain the one that best fits the covariance structure. The likelihood ratio test and Akaike’s information criterion were used to select the best fit covariance structure. The univariate normality assumption was verified using a Shapiro–Wilk test on the error distributions from the statistical model after applying a Cholesky factorization. The Brown and Forsythe’s variation of Levene’s test was used to verify the homogeneity of variances. All variables were log-transformed to fulfill the model assumptions and the reported p values are based on these transformations. For variables with non-detectable values (left censored), a non-parametric-mixed statistical model on longitudinal data, proposed by Brunner, Domhof, and Langer Citation2002, was performed. The results were considered significant when p-values were less than 0.05. The data were analyzed using the statistical software SAS v9.4 (SAS Institute Inc., Cary, NC).
Results
Airborne dust
On day 0, during the filling of the pipes with RMS, SW, TW, and DC24 composting methods showed the lowest dust concentration (P < .005) for all dust particle sizes (total dust particles, PM10, PM2.5, and PM1). Following 5 days of aging, DC24 and DC72 methods yielded lower dust levels (P < .04) than SW and TW. On day 10, SW, TW, and DC24 were once again associated with the lowest concentration (P < .04) for all dust particle sizes (). For all dust particle sizes, on day 10, concentration was lower (P < .03) before the turnover of the TW piles than during the mechanical disturbance of piles (). On day 5, no statistical difference was observed ().
Table 2. Airborne dust, total bacteria, Penicillium/Aspergillus, and endotoxin concentrations in chambers containing RMS composted by static windrow (SW), daily turned windrow (TW), static windrow following 24 h in a rotating composting drum (DC24) or by static windrow following 72 h in a rotating composting drum (DC72)
Table 3. Airborne dust, total bacteria, Penicillium/Aspergillus, and endotoxin concentrations in chambers containing RMS composted by daily turned windrow
Total airborne bacteria and Penicillium/Aspergillus
On day 0, the SW and TW composting methods yielded lower concentration (P < .04) of total bacteria than the DC24 and DC72 methods. The SW and TW methods were also associated with lower concentration (P < .02) of airborne Penicillium/Aspergillus than DC72 during the filling of the pipes (day 0), while the DC24 piles were associated with lower airborne Penicillium/Aspergillus concentration than SW (P = .01) on day 0 (). After 5 days of aging, SW, TW, and DC24 associated bioaerosols yielded the lowest concentration (P < .04) of total airborne bacteria, while SW showed lower levels (P < .02) of airborne Penicillium/Aspergillus than DC24. On day 10, SW and TW composting methods resulted in lower concentrations (P < .04) of total bacteria than DC24, while SW, as for day 5, resulted in lower airborne Penicillium/Aspergillus concentration (P = .01) than DC24.
During the turnover of TW RMS piles, total airborne bacteria and Penicillium/Aspergillus concentration was higher (P < .001) than before the turnover ().
Airborne pathogens
Escherichia coli, E. coli O157:H7, Klebsiella pneumoniae, Legionella sp., L. pneumophila, L. longbeachae, Staphylococcus aureus, methicillin-resistant S. aureus (MRSA), Salmonella sp., Streptococcus agalactiae, and S. rectivirgula were not detected in the air of any chamber. For A. fumigatus, on day 5, one DC24 associated air sample revealed a concentration above the detection limit of the analysis method with 2.0 × 102 gene copies/m3 (data not shown).
Airborne endotoxins
For all sampling days, airborne endotoxin concentration was not significantly different between the studied composting methods. However, even if statistical significance could not be reached, DC24 bioaerosols seemed to exhibit higher endotoxin concentration than the other composting methods (). Not surprisingly, during TW piles turnover, endotoxin concentration was higher (P = .002) than before the turnover ().
Discussion
Bioaerosols and air quality represent a daily challenge for dairy farmers. Given their nature, RMS could be a significant source of bioaerosols. Although few recommendations exist for bioaerosol exposure limits, various indicators were studied to evaluate the potential contribution of RMS composting to air quality reduction. The objectives of the present study were to evaluate the bioaerosols associated with each RMS composting methods and handling, and subsequently, to identify the method more promising with regards to minimizing bioaerosols exposure. The SW, TW, DC24 and DC72 composting methods and the experimental setup (e.g., static and turned windrows, 10-day aging of piles) were studied as these methods are more likely to be adopted by dairy farmers than other time-consuming and labor-intensive sanitation measures. During the experiments, piles reached the desired composting temperature range of 40°C to 65°C either in piles in the experimental chambers or in the drum composter (Fournel et al. Citation2019). In the bottom of the piles, the temperature reached similar levels (40–50°C) for all composted RMS. However, for the top part of the piles, TW responded differently with temperature of 35–45°C compared to 60°C for the other methods during the last 7 days of the experiment (Fournel et al. Citation2019).
On day 0, during the filling of the pipes with RMS, the DC24 composting method led to the lowest concentration of PM1 and PM2.5, while SW, TW, and DC24 yielded the lowest concentrations of PM10 and total dust. SW and TW methods were associated with the lowest total bacteria concentration and DC24 associated bioaerosols contained the lowest Penicillium/Aspergillus concentration. It is not surprising that SW and TW methods showed similar bioaerosols since the RMS piled in those chambers both came directly from the screw press without having been in the drum composter. Following 5 days of aging, DC24 and DC72 methods resulted in the lowest concentrations of PM1, PM2.5, PM10, and total dust, while SW was associated with the lowest concentrations of total bacteria and Penicillium/Aspergillus. On day 10, RMS produced by SW and TW methods led to the lowest concentrations of PM1, PM2.5, PM10, total dust, and Penicillium/Aspergillus (). Overall, RMS composted by SW and TW methods yielded the lowest concentrations of most air quality indicators measured on the three sampling days. However, handling the RMS will likely contribute to air quality deterioration in dairy barns. As shown in the present study, there was a significant difference between before and during the turnover of TW piles for concentrations of total airborne bacteria, Penicillium/Aspergillus, and endotoxins.
None of the RMS composting methods was associated with concentrations exceeding the recommended total organic dust TLV of 10 mg/m3 (). Results are consistent with those reported in previous studies conducted in dairy barns, in which no dust concentrations were higher than the TLV-TWA (Basinas et al. Citation2014; Burch et al. Citation2010; Choudhry et al. Citation2012; Eduard, Pearce, and Douwes Citation2009; Garcia et al. Citation2013; Kullman et al. Citation1998; Reynolds et al. Citation2012; Samadi et al. Citation2012). On the other hand, for all evaluated methods, the piling of RMS on day 0 and the turnover of the TW piles yielded airborne endotoxin concentrations of up to 5 times higher than the European suggested exposure limit value of 90 EU/m3 () (DECOS Citation2010). Concentrations up to 40,000 EU/m3 of air were already described in commercial dairy barns (Basinas et al. Citation2014; Choudhry et al. Citation2012; Duchaine et al. Citation1999; Eduard, Pearce, and Douwes Citation2009; Garcia et al. Citation2013; Reynolds et al. Citation2012; Samadi et al. Citation2012). Unfortunately, there is no suggested exposure limit value for airborne microorganisms detected by molecular biology (PCR). In the present study, less than 5 × 107 E. coli eq/m3 for total bacteria and 4 × 103 A. fumigatus eq/m3 for Penicillium/Aspergillus were observed in the chambers (). In commercial barns, up to 108 gene copies/m3 for total bacteria and 106 gene copies/m3 for Penicillium/Aspergillus were revealed (Lecours et al. Citation2012; Mbareche et al. Citation2019). Even though concentrations up to 104 gene copies/m3 of A. fumigatus were previously observed in dairy barns by Mbareche et al. (Mbareche et al. Citation2019), only one air sample associated with a DC24 pile on day 5 showed a concentration above the detection limit of the analysis method. Moreover, the bacteria K. pneumonia, Legionella species, and S. rectivirgula were not found in the air of any chambers. No study has ever reported airborne K. pneumoniae and Legionella species in commercial dairy barn, but Lecours et al. (Citation2012) did reveal the presence of the actinomycete S. rectivirgula in farms, ranging from below the detection limit to 1.3 × 107 gene copies/m3 (Lecours et al. Citation2012). Klebsiella pneumoniae and Legionella species are both responsible for pneumonias in humans. Streptococcus, Staphylococcus, and Klebsiella species as well as E. coli have previously been found in RMS using culture (Fournel et al. Citation2019; Harrison, Bonhotal, and Shwarz Citation2008; Husfeldt et al. Citation2012). In the present study, when RMS were aging or even handled (filling of the pipes and daily turnover for TW piles), these bacteria were not detected in bioaerosols using qPCR (that includes culturable and non-culturable cells). Likewise, airborne E. coli, E. coli O157:H7, S. aureus, Salmonella sp., S. agalactiae, and MRSA were not present or concentrations were below the detection limits. In humans, E. coli, E. coli O157: H7, and Salmonella sp. are common etiologic agents of food poisoning (foodborne diseases) cases while S. aureus and MRSA could be involved in skin and soft-tissue infections, and even bacteremia. S. agalactiae are a known etiologic agent of severe invasive infections in newborns and immunocompromised people.
Although the results of the present study were obtained in environmentally controlled chambers and, thus, cannot be directly extrapolated to commercial dairy barns, high concentration of bioaerosols while the turnover of RMS piles suggest that handling RMS may jeopardize dairy producers’ respiratory health. Garcia et al. (Citation2013) demonstrated that compared to other tasks, the addition of bedding materials in dairy barns is associated with higher levels of airborne dust (PM1, PM2.5) and endotoxins. Moreover, Samadi et al. (Citation2012) described differences in the air quality of dairy barns using different types of bedding materials (Samadi et al. Citation2012). In the present study, even composting methods of RMS coming from one Canadian dairy farm impacted differently the nature (content) and the production (concentrations) of bioaerosols. Producers should therefore consider wearing respiratory protective equipment (e.g., N95 mask) to protect themselves from exposure to bioaerosols when handling RMS or other types of bedding materials. Finally, air quality in Canadian commercial dairy barns needs to be further investigated as management of the buildings, the herd, and the bedding materials are handled differently by each dairy producers. Forthcoming investigations will need to detail the bedding material used in sampled barns, or the bedding management system as it might explain the variability in dust and endotoxin concentrations observed between published studies. The variability may as well be explained by the sampling methods, the seasons, the animal density, and the geographical area (Garcia et al. Citation2013; Reynolds et al. Citation2013). Thus, a better description of the bedding material and management in each evaluated dairy barn could lead to an understanding of the impact of bedding material on air quality.
Conclusion
Among the four studied RMS composting methods, SW and TW led to the lowest production of bioaerosols in environmentally controlled chambers. However, data from the chambers could not be extrapolated to determine air quality impact in commercial dairy barns in which air movements, animals (number and activities), feed handling, and maintenance activities can also be sources of bioaerosols. Yet, dairy producers could refer to the results of the present study to select RMS production and management methods inside barns. Finally, the type of bedding material and bedding management need to be detailed in research on air quality in dairy barns for accurate comparison between studies.
Supplemental Material
Download MS Word (19.2 KB)Acknowledgment
This work was supported by the Canadian Agricultural Adaptation Program (2014-2019) from Agriculture and Agri-Food Canada (AAFC, Ottawa, ON, Canada). The authors are thankful to the Fonds de recherche du Québec—Nature et technologies (FRQNT, Québec, Québec, Canada), the Centre de Recherche en Sciences Animales de Deschambault (CRSAD, Deschambault, Québec, Canada), the Institut de recherche Robert-Sauvé en santé et sécurité du travail (IRSST, Québec, Québec, Canada) and the Université Laval (Québec, Québec, Canada) for student scholarships (K. Duquette-Lozeau and S. Fournel). J. Lemieux was supported through CD Discovery program of the Natural Sciences and Engineering Research Council of Canada RGPIN-2014-05900 (NSERC, Ottawa, Ontario, Canada). Special thanks to the IRDA’s team for providing technical and professional support, to Serge Simard for statistical analyses and to Amanda Topperoff and Michi Waygood (BLUE-PENCIL) for English revision of the manuscript.
Disclosure statement
No potential conflict of interest was reported by the authors.
Supplementary material
Supplemental data for this paper can be accessed on the publisher’s website.
Additional information
Funding
Notes on contributors
Karine Duquette-Lozeau
Karine Duquette-Lozeau completed a MSc in microbiology at the Université Laval (Research supervisor: Caroline Duchaine). The present study is the subject of her master’s thesis.
Valérie Létourneau
Valérie Létourneau is a research associate in Caroline Duchaine’s laboratory at the Centre de recherche de l’Institut universitaire de cardiologie et de pneumologie de Québec-Université Laval. She completed a Ph.D. in microbiology at Université Laval and is since working on air quality assessment and mitigation of bioaerosols in different agricultural settings.
Joanie Lemieux
Joanie Lemieux completed a MSc in microbiology at the Université Laval (Research supervisor: Caroline Duchaine).
Sébastien Fournel
Sébastien Fournel is an assistant professor at Université Laval in Agri-Food Engineering. He is also an Educational Leadership Chairholder on Sustainable Agricultural Buildings. His research work includes precision livestock farming, environmental control of animal buildings, manure management, animal welfare, gas, odor and bioaerosol production at the farm level, conversion of agri-food residues and biomass in energy and bioproducts, and soil and water quality from farm operations.
Caroline Côté
Caroline Côté is an agronomist and realized a Ph.D. in veterinary science at the University of Montreal where she is now associate professor. She has been working for 20 years as researcher at the Research institute for the Agri-Environment (IRDA) on the hygienic aspects of manure and irrigation water management. She has realized many research projects regarding the fate of indicator and pathogenic microorganisms in the environment.
Stéphane Godbout
Stéphane Godbout completed a Ph.D. in engineering in 1996. He worked three years for The Quebec Hog Development Center where he initiated research projects in swine production. Since 2000, he is a research scientist in engineering at Research and Development Institute for the Agri-Environment (IRDA) and an assistant professor at Université Laval. His research program focus mainly on animal production including manure management (treatment, separation, spreading), air quality and treatment, odours, biomaterials, bioenergy, LCA and animal welfare.
Caroline Duchaine
Caroline Duchaine is a full professor in the Département de biochimie, de microbiologie et de bio-informatique, Université Laval, researcher at the Centre de recherche de l’Institut universitaire de cardiologie et de pneumologie de Québec-Université Laval and holder of the Canada Research Chair on Bioaerosols. Since 2000, she has headed the bioaerosol research team, leader in aerovirology, air microbiota analyzes, in vitro bioaerosols, industrial hygiene and agricultural health. She works closely with doctors, engineers, agronomists and physicists in order to develop transdisciplinary research projects aimed at understanding the role of bioaerosols in several contexts: public health, veterinary, agriculture, industry and hospitals. She has supervised more than 115 students and participated in more than 110 research projects, funded by provincial, national and international agencies. She has published over 500 peer-reviewed manuscripts, reports and abstracts. She has been a Fonds de recherche du Québec-Santé and Canadian Institute for Health Research Fellow.
References
- Bach, H. J., J. Tomanova, M. Schloter, and J. C. Munch. 2002. Enumeration of total bacteria and bacteria with genes for proteolytic activity in pure cultures and in environmental samples by quantitative PCR mediated amplification. J. Microbiol. Methods 49 (3):235–45. doi:10.1016/S0167-7012(01)00370-0.
- Basinas, I., T. Sigsgaard, M. Erlandsen, N. T. Andersen, H. Takai, D. Heederik, O. Omland, H. Kromhout, and V. Schlunssen. 2014. Exposure-affecting factors of dairy farmers’ exposure to inhalable dust and endotoxin. Ann. Occup. Hyg. 58 (6):707–23.
- Boucher, M., P. B. Lecours, V. Létourneau, M. Veillette, C. Duchaine, and D. Marsolais. 2018. Organic components of airborne dust influence the magnitude and kinetics of dendritic cell activation. Toxicol. Vitro 50:391–98. doi:10.1016/j.tiv.2018.04.011.
- Brunner, E., S. Domhof, and F. Langer. 2002. Nonparametric analysis of longitudinal data in factorial experiments. New York, NY: Wiley.
- Burch, J. B., E. Svendsen, P. D. Siegel, S. E. Wagner, S. von Essen, T. Keefe, J. Mehaffy, A. S. Martinez, M. Bradford, L. Baker, et al. 2010. Endotoxin exposure and inflammation markers among agricultural workers in Colorado and Nebraska. J. Toxicol. Env. Health Part A 73 (1):5–22. doi:10.1080/15287390903248604.
- Choudhry, A. H., S. J. Reynolds, J. Mehaffy, D. I. Douphrate, K. Gilmore, J. L. Levin, and M. W. Nonnenmann. 2012. Evaluation of parlor cleaning as an intervention for decreased occupational exposure to dust and endotoxin among dairy parlor workers-a pilot study. J. Occup. Environ. Hyg. 9 (7):D136–D140. doi:10.1080/15459624.2012.691410.
- Cormier, Y., J. Belanger, P. Leblanc, and M. Laviolette. 1986. Bronchoalveolar lavage in farmers lung-disease - diagnostic and physiological significance. Br. J. Ind. Med. 43 (6):401–05.
- Dagenais, T. R. T., and N. P. Keller. 2009. Pathogenesis of Aspergillus fumigatus in invasive aspergillosis. Clin. Microbiol. Rev. 22 (3):447–65. doi:10.1128/CMR.00055-08.
- de Carvalho, N. L., J. L. Goncalves, B. G. Botaro, L. F. de Prada-silva, and M. V. Dos Santos. 2015. Detection and enumeration of streptococcus agalactiae from bovine milk samples by real-time polymerase chain reaction. Curr. Microbiol. 71 (3):363–72. doi:10.1007/s00284-015-0855-1.
- Donham, K. J. 1986. Hazardous agents in agricultural dusts and methods of evaluation. Am. J. Ind. Med. 10 (3):205–20. doi:10.1002/ajim.4700100305.
- Duchaine, C., A. Meriaux, G. Brochu, and Y. Cormier. 1999. Airborne microflora in Quebec dairy farms: Lack of effect of bacterial hay preservatives. Am. Ind. Hyg. Assoc. J. 60 (1):89–95. doi:10.1080/00028899908984426.
- Dutch Expert Committee on Occupational Safety (DECOS). A Committee of the Health Council of the Netherlands in cooperation with the Nordic expert group for criteria documentation of health risks from chemicals No. 2010/04OSH, The Hague, July 15, 2010.
- Eduard, W., N. Pearce, and J. Douwes. 2009. Chronic bronchitis, COPD, and lung function in farmers the role of biological agents. Chest 136 (3):716–25. doi:10.1378/chest.08-2192.
- Environment Canada. 2018. Historical data. Accessed June 29, 2018. https://climate.weather.gc.ca/historical_data/search_historic_data_e.html
- Fournel, S., S. Godbout, P. Ruel, A. Fortin, K. Duquette-Lozeau, V. Letourneau, M. Genereux, J. Lemieux, D. Potvin, C. Cote, et al. 2019. Production of recycled manure solids for use as bedding in Canadian dairy farms: II. Composting methods. J. Dairy Sci. 102 (2):1847–65. doi:10.3168/jds.2018-14967.
- Garcia, J., D. H. Bennett, D. Tancredi, M. B. Schenker, D. Mitchell, S. J. Reynolds, and F. M. Mitloehner. 2013. Occupational exposure to particulate matter and endotoxin for California dairy workers. Int. J. Hyg. Environ. Health 216 (1):56–62. doi:10.1016/j.ijheh.2012.04.001.
- Hamilton, K. A., W. Ahmed, A. Palmer, J. P. S. Sidhu, L. Hodgers, S. Toze, and C. N. Haas. 2016. Public health implications of Acanthamoeba and multiple potential opportunistic pathogens in roof-harvested rainwater tanks. Environ. Res. 150:320–27. doi:10.1016/j.envres.2016.06.017.
- Harrison, E., J. Bonhotal, and M. Shwarz. 2008. Using manure as solid bedding. New-York: Conell Waste Management Institute.
- Haugland, R. A., M. Varma, L. J. Wymer, and S. J. Vesper. 2004. Quantitative PCR analysis of selected Aspergillus, Penicillium and Paecilomyces species. Syst. Appl. Microbiol. 27 (2):198–210. doi:10.1078/072320204322881826.
- Herpers, B. L., B. M. de Jongh, K. van der Zwaluw, and E. J. van Hannen. 2003. Real-time PCR assay targets the 23S-5S spacer for direct detection and differentiation of Legionella spp. and Legionella pneumophila. J. Clin. Microbiol. 41 (10):4815–16. doi:10.1128/JCM.41.10.4815-4816.2003.
- Huletsky, A., R. Giroux, V. Rossbach, M. Gagnon, M. Vaillancourt, M. Bernier, F. Gagnon, K. Truchon, M. Bastien, F. J. Picard, et al. 2004. New real-time PCR assay for rapid detection of methicillin-resistant Staphylococcus aureus directly from specimens containing a mixture of staphylococci. J. Clin. Microbiol. 42 (5):1875–84. doi:10.1128/JCM.42.5.1875-1884.2004.
- Husfeldt, A. W., M. I. Endres, J. A. Salfer, and K. A. Janni. 2012. Management and characteristics of recycled manure solids used for bedding in midwest freestall dairy herds. J. Dairy Sci. 95 (4):2195–203. doi:10.3168/jds.2011-5105.
- Joly, P., P. A. Falconnet, J. Andre, N. Weill, M. Reyrolle, F. Vandenesch, M. Maurin, J. Etienne, and S. Jarraud. 2006. Quantitative real-time Legionella PCR for environmental water samples: Data interpretation. Appl. Environ. Microbiol. 72 (4):2801–08. doi:10.1128/AEM.72.4.2801-2808.2006.
- Kullman, G. J., P. S. Thorne, P. F. Waldron, J. J. Marx, B. Ault, D. M. Lewis, P. D. Siegel, S. A. Olenchock, and J. A. Merchant. 1998. Organic dust exposures from work in dairy barns. Am. Ind. Hyg. Assoc. J. 59 (6):403–13. doi:10.1080/15428119891010668.
- Lecours, P. B., M. Veillette, D. Marsolais, and C. Duchaine. 2012. Characterization of bioaerosols from dairy barns: Reconstructing the puzzle of occupational respiratory diseases by using molecular approaches. Appl. Environ. Microbiol. 78 (9):3242–48. doi:10.1128/AEM.07661-11.
- Lu, Z., J. Wang, and Y. Zhang. 2012. Quantitative real-time PCR detection of airborne Staphylococcus aureus in hospital indoor atmosphere. Modern Appl. Sci. 6 (3). doi:10.5539/mas.v6n3p22.
- Malinen, E., A. Kassinen, T. Rinttila, and A. Palva. 2003. Comparison of real-time PCR with SYBR Green I or 5 ‘-nuclease assays and dot-blot hybridization with rDNA-targeted oligonucleotide probes in quantification of selected faecal bacteria. Microbiology-(UK) 149:269–77. doi:10.1099/mic.0.25975-0.
- Malmberg, P. 1990. Health-effects of organic dust exposure in dairy farmers. Am. J. Ind. Med. 17 (1):7–15. doi:10.1002/ajim.4700170104.
- Mbareche, H., M. Veillette, G. J. Bilodeau, and C. Duchaine. 2019. Fungal aerosols at dairy farms using molecular and culture techniques. Sci. Total Environ. 653:253–63. doi:10.1016/j.scitotenv.2018.10.345.
- Menear, J. R., and L. W. Smith. 1973. Dairy-cattle manure liquid-solid separation with a screw press. J. Anim. Sci. 36 (4):788–91. doi:10.2527/jas1973.364788x.
- Reynolds, S. J., M. L. Clark, N. Koehncke, S. von Essen, L. Prinz, T. J. Keefe, J. Mehaffy, M. Bradford, B. Cranmer, M. E. Davidson, et al. 2012. Pulmonary function reductions among potentially susceptible subgroups of agricultural workers in Colorado and Nebraska. J. Occup. Environ. Med. 54 (5):632–41. doi:10.1097/JOM.0b013e31824d2e1c.
- Reynolds, S. J., M. W. Nonnenmann, I. Basinas, M. Davidson, L. Elfman, J. Gordon, S. Kirychuck, S. Reed, J. W. Schaeffer, M. B. Schenker, et al. 2013. Systematic review of respiratory health among dairy workers. J. Agromedicine 18 (3):219–43. doi:10.1080/1059924X.2013.797374.
- Samadi, S., F. van Eerdenburg, A. R. Jamshidifard, G. P. Otten, M. Droppert, D. J. J. Heederik, and I. M. Wouters. 2012. The influence of bedding materials on bio-aerosol exposure in dairy barns. J. Expo. Sci. Environ. Epidemiol 22 (4):361–68. doi:10.1038/jes.2012.25.
- Schafer, J., P. Kampfer, and U. Jackel. 2011. Detection of Saccharopolyspora rectivirgula by quantitative real-time PCR. Ann. Occup. Hyg. 55 (6):612–19.
- Schenker, M. B., D. Christiani, Y. Cormier, H. Dimich-Ward, G. Doekes, J. Dosman, J. Douwes, K. Dowling, D. Enarson, F. Green, et al.. 1998. Respiratory health hazards in agriculture. Am. J. Respir. Crit. Care Med. 158(5):S1–S76.
- Shannon, K. E., D. Y. Lee, J. T. Trevors, and L. A. Beaudette. 2007. Application of real-time quantitative PCR for the detection of selected bacterial pathogens during municipal wastewater treatment. Sci. Total Environ. 382 (1):121–29. doi:10.1016/j.scitotenv.2007.02.039.
- Thorn, J. 2001. The inflammatory response in humans after inhalation of bacterial endotoxin: A review. Inflamm. Res. 50 (5):254–61. doi:10.1007/s000110050751.
- Walser, S. M., D. G. Gerstner, B. Brenner, J. Bunger, T. Eikmann, B. Janssen, S. Kolb, A. Kolk, D. Nowak, M. Raulf, et al. 2015. Evaluation of exposure-response relationships for health effects of microbial bioaerosols - A systematic review. Int. J. Hyg. Environ. Health 218 (7):577–89. doi:10.1016/j.ijheh.2015.07.004.