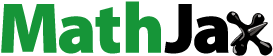
ABSTRACT
Respiratory droplets serve as a viable transmission mechanism for many viruses and other pathogens. Facemasks are commonly used to minimize the risk of this transmission. However, information on the size-resolved filtration efficiency of commonly available commercial facemasks is not readily available in the literature. To fill this gap, the current study performs aerosolized chamber experiments to evaluate the filtration efficiencies of commonly available commercial facemasks’ materials in a size range of 0.3–10 µm. Results rank the performance of filtration through commercial facemasks’ materials as follows (values in brackets indicate the average filtration efficiencies across 0.3–10 µm): 6-Layer N95 mask (0.918) > N95 mask – without valve (0.88) > KN95 mask (0.84) > N95 mask -with valve (0.834) > Heavy knitted cotton mask (0.808) > Surgical mask (0.778) > Cotton mask-2 layers (0.744) > Nylon fabric mask-2 layers (0.740) > T-shirt fabric mask-2 layers (0.708) > T-shirt fabric mask-1 layer (0.648). The size-resolved filtration efficiencies through the material across the evaluated commercial facemasks ranged from 38–83% in the size range of 0.3–0.5 µm, 55–88% in the size range of 0.5–1 µm, 69–93% in the size range of 1–2.5 µm, 76–96% in the size range of 2.5–5 µm, and 86–99% in the size range of 5–10 µm. Subsequently, the filtration efficiencies of materials post washing (with detergent in warm water and allowing to dry completely) were also evaluated. The average reduction in filtration efficiencies post washing are as follows: 6-Layer N95 mask: 3%, N95 mask – without valve: 2%, KN95 mask: 4%, N95 mask -with valve: 3%, Heavy knitted cotton mask: 4%, Surgical mask: 18%, Cotton mask-2 layers: 11%, Nylon fabric mask-2 layers: 6%, T-shirt fabric mask-2 layers: 6%, T-shirt fabric mask-1 layer: 8%. This decrease in the filtration efficiency was more pronounced for the sub-micron particles than the super-micron ones.
Implications: Facemasks are commonly used to minimize the risk of pathogens through ambient air transmission. However, information on the size-resolved filtration efficiency of commonly available commercial facemasks materials is not readily available in the literature. To fill this gap, the current study performs aerosolized chamber experiments to evaluate the filtration efficiencies of commonly available commercial facemasks materials in a size range of 0.3–10 µm. The performance of the commercial facemasks materials as follows in the order of (values in brackets indicate the average filtration efficiencies across 0.3–10 µm): 6-Layer N95 mask (0.918) > N95 mask – without valve (0.88) > KN95 mask (0.84) > N95 mask -with valve (0.834) > Heavy knitted cotton mask (0.808) > Surgical mask (0.778) > Cotton mask-2 layers (0.744) > Nylon fabric mask-2 layers (0.740) > T-shirt fabric mask-2 layers (0.708) > T-shirt fabric mask-1 layer (0.648). The choice of facemask is greatly driven by the size of viable respiratory droplets that need to be eliminated. If droplets with particle size less than 0.5 µm are required to be filtered, N95 masks without the valve or more layers are preferred. If the primary objective is to filter particles between 0.5–1 µm, then N95 (both with or without valves) or KN95 masks are recommended. Surgical masks and heavy knitted cotton masks may also be used for this purpose, but with caution.
Introduction
Human-to-human transmission of respiratory viruses and other pathogens (measles virus, Mycobacterium tuberculosis, etc.) via respiratory droplets has been widely documented in the epidemiology literature (Dbouk and Drikakis Citation2020; Manjarrez-Zavala Citation2013; Richard et al. Citation2020; Stadnytskyi et al. Citation2020). Speaking, coughing, breathing, or sneezing activities are the primary sources of human respiratory droplets (Dbouk and Drikakis Citation2020; Johnson et al. Citation2011). Facemasks are commonly used to minimize the risk of transmission of pathogens through these droplet particles. For instance, to limit the spread of the SARS-CoV-2 (widely referred to as COVID-19) virus during the ongoing pandemic, several government authorities made the use of facemasks mandatory in public areas.
Particle filtration in facemasks is majorly achieved through inertial impaction, interception, and diffusion mechanisms (Hinds Citation1999) (a detailed discussion on the filtration mechanism of facemasks is given in section S1 of the supplementary section). For particles less than 0.1 µm, diffusion mechanism dominates, and for particles greater than 1 µm, both inertial impaction and interception mechanisms are noticeable (see section S1 in the supplementary section). However, for particles between 0.1–1 µm, both diffusion and interception take place, but none dominates. As a result, a local minimum in particle filtration efficiency (see Figure S2) is observed in this region (Lee and Liu Citation1980; Mahdavi Citation2013). This minimum filtration efficiency is reported for ~0.3 µm particle diameter (Mahdavi Citation2013).
Typically, respiratory droplet particles tend to have a broad size distribution with predominant modes in the super-micron (> 1 µm) region (Cao et al. Citation2015; Johnson et al. Citation2011; Stadnytskyi et al. Citation2020). However, particles greater than 10 µm would tend to settle fast (at T = 298 K and P = 1 atm, the terminal settling velocity for 0.3 µm particles is ~ 1.6 cm h−1; for 1 µm particles is ~ 12.7 cm h−1; and for 10 µm particles is ~1092 cm h−1) due to their high settling velocities (calculation of terminal settling velocities for varying particle diameter is discussed in section S2 of the supplementary section). Therefore, it is crucial to understand the size-resolved filtration efficiency of facemasks in the range of 0.3 (minimum filtration efficiency) – 10 (particles greater than 10 µm will tend to settle quickly) µm. Researchers have attempted to assess the filtration efficacy of commonly available commercial facemasks in the past (Fischer et al. Citation2020; Steinbrook Citation2020; Verma, Dhanak, and Frankenfield Citation2020; Whiley et al. Citation2020). However, information on the size-resolved filtration efficiency of commonly available commercial facemasks is limited. Therefore, the current study aims to evaluate the size-resolved filtration efficiency of 10 commonly available (in India) commercial facemasks’ material using two state-of-the-art optical particle counters in aerosolized chamber experiments. The following section discusses the experimental setup and measurement of filtration efficiency in detail.
Materials and methods
A schematic of the experimental setup for evaluating the filtration efficiency of commercial facemasks’ material is shown in (an actual snapshot of the experimental setup is shown in Figure S4 in the supplementary section). It consists of two sealed acrylic chambers: the Upstream mixing chamber and the Downstream collection chamber. Particle-laden air flows from the upstream mixing chamber to the downstream collection chamber through the mask specimen attached to a mannequin head. Double-sided tapes were used to seal the mask specimens firmly onto it. The purpose of double-sided tape was to prevent particle leakage around the edges of the mask and ensure characterization of filtration solely through facemasks’ material.
Figure 1. Schematic of the experimental setup for evaluating the filtration efficiency of commercial facemasks’ materials
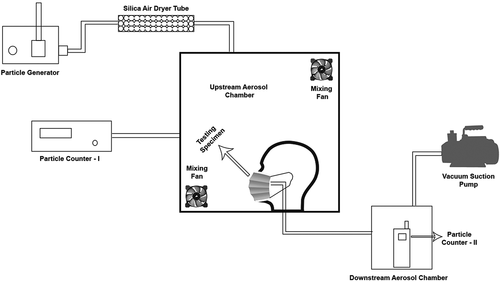
TSI particle generator was used to generate aerosol particles. For particle generation, compressed air passes through a venturi forming a low-pressure zone that draws the solution to be aerosolized from a container. The solution subsequently rises to a point where it meets the air stream, thereby breaking into aerosol droplets of various sizes. For the study’s experiments, a solution of Sodium Chloride and Calcium Carbonate (3:1 by weight) was used in the particle generator, producing aerosols in both accumulation and coarse mode. Therefore, the generated aerosol is a wet mixture of NaCl and CaCO3. NaCl is a highly hygroscopic (κ = 1.28) inorganic aerosol (Petters and Kreidenweis Citation2007), with growth factor reported up to 2.25 in diameter at RH = 85% (Hansson et al. Citation1998). On the other hand, CaCO3 is a non-hygroscopic inorganic aerosol with κ ~ 0.043 and reported with a negligible growth (average growth factor = 1 ± 0.02) over the RH range of 3–90% (Gibson, Hudson, and Grassian Citation2006). However, CaCO3 majorly constituted the coarse mode particles. Consequently, the majority of the generated aerosol particles are expected to be in the coarse fraction. This could result in saturation and increased particle losses at different inlets in the experimental chamber, attributable to higher impaction of larger particles. Therefore, to avoid such issues, the generated particle-laden air was dried (causing the highly hygroscopic aerosol, NaCl, to lose water and shrink) using a silica dryer tube before sending it to the mixing chamber. The size distribution of generated aerosols (after being dried using silica dryer) measured (using GRIMM PAS 1.108) over a 12-hour continuous run period is presented in Figure S5 in the supplement section. Two diagonally placed mixing fans ensured uniform mixing of particles inside the upstream mixing chamber.
Particle-laden air from the upstream mixing chamber is sucked through the mask specimen into the downstream collection chamber using a vacuum suction pump. The flow rates of vacuum suction and particle generation were kept the same (~10 lpm; breathing rate is: ~7–8 LPM). During the experiments, the flow rates were continuously monitored using mass flow meters (Model: TSI 4040 Mass Flow Meter). However, due to the lack of a datalogger, the values were not documented. Nevertheless, observations suggested that the flow values were steady and close to 10 lpm throughout the experimental study. Measurements of particle concentration in the upstream and downstream chambers were carried out using GRIMM PAS 1.108 (Sousan et al. Citation2016) and CEM DT-9880 (Ilyashenko, Kovshov, and Navitskaite Citation2019), respectively. Both these instruments employ the optical scattering principle (Patra et al. Citation2021) to count the number of particles per cubic centimeter of air. The concentration output of both these instruments is given in terms of size-fractionated bins. The bin widths of both the instruments were matched as follows: 0.3–0.5 µm, 0.5–1 µm, 1–2.5 µm, 2.5–5 µm and 5–10 µm. The filtration efficiencies through the commercial facemasks’ materials were calculated for each of these size bins in the experiments using EquationEquation (1)(1)
(1) . Higher numerical values of filtration efficiencies indicate better performance of the facemasks’ material.
To evaluate the filtration efficiency, ten commonly available commercial facemasks are used. The evaluation was performed in two stages. First, fresh unused masks were evaluated. Thereafter, the same masks were washed using detergent in warm water, sun-dried for two hours, and were subsequently tested after a gap of one week, ensuring no residual humidity in the masks. Therefore, the study not only evaluates the filtration efficiency of the commercial facemasks’ materials, but also understands how the performance of the masks’ material changes once they are washed. The masks tested in this study are as follows: Surgical mask, N95 mask (without valve), N95 mask (with valve), KN95 mask, 6-Layer N95 mask, Cotton mask (2 layers), T-Shirt fabric mask (2 layers), T-Shirt fabric mask (1 layer), Nylon fabric mask (2 layers), and Heavy knitted cotton mask (2 layers) (Figure S6 in the supplementary section). It is to be noted that the term “N95” used in this study refers to the readily available commercial N95 filtering facemasks, four kinds of which were evaluated – Typical N95 facemask (without valve), Typical N95 facemask (with valve), KN95 facemask, and 6-layer N95 facemask.
In each experimental run, a particular facemask was mounted on the mannequin head using double-sided tapes, and particle concentration measurements were conducted for continuous 60 mins. However, before starting these experiments, ten minutes of lead time was given, wherein both the particle generation and suction through the mask operated without concentration measurements. This was given to allow uniform mixing of particles in the chamber. Three similar masks of each kind were tested to avoid any potential bias. The filtration efficiency reported per bin for each mask is the mean of the filtration efficiency through their materials obtained in all three runs for the experimental duration.
For statistical evaluation, two-tailed statistical t-tests are used in this study to compare the filtration efficiencies of any two masks or assess the percentage decrease in the filtration efficiency of the masks’ materials post washing. These t-tests were conducted with the null hypothesis that the difference in filtration efficiencies between the two masks being compared is not statistically significant and the alternative hypothesis that the difference is statistically significant. At 95% confidence, if the p-value of the test is less than 0.05, then the null hypothesis is rejected, inferring that the difference is statistically significant (Patra, Chilukuri, and Vanajakshi Citation2021). A p-value greater than 0.05 suggests no statistical evidence of disparity in comparison.
One thing to note here is that the filtration efficiency calculation in this experimental setup is centric to upstream and downstream concentration estimation given by GRIMM PAS 1.108 and CEM DT-9880, respectively. Therefore, to account for sensor response and particle losses through the tubing, co-location experiments were performed with particle generation and suction in the setup without any filtration specimen. This experiment was run for eight continuous hours before starting the filtration evaluation experiments. The outputs of both the instruments were used to calculate the correction factors (CF) for different bins (using EquationEquation (2)(2)
(2) ), which were used in the experimental measurements to account for these losses. Results obtained for the evaluation of filtration efficiencies of the facemasks’ materials are discussed in the next section.
Results
presents the correction factor obtained for the experimental setup across all the bins. The correction factor for particles smaller than 1 µm is attributed to the lower sensitivity of the CEM DT-9880 instrument in detecting sub-micron particles. For larger particles, particle losses through impaction on tubings are higher. Therefore, as the particle size increases in the super-micron region, particle loss due to impaction also increases. Consequently, the downstream concentration decreases; hence, decreasing the CF. The obtained CF values were used in the subsequent concentration measurements to account for these losses.
Once the CFs for all the bins were obtained, filtration efficiency evaluation experiments were carried out. The results of filtration efficiency through the materials for all the evaluated commercial facemasks is presented in .
It can be seen from that the filtration efficiency through the material of all the evaluated masks increased with increasing particle size. Overall, the performance of the facemasks’ material can be summarized as follows (values in brackets indicate the average filtration efficiencies across all bins): 6-Layer N95 mask (0.918) > N95 mask – without valve (0.88) > KN95 mask (0.84) > N95 mask -with valve (0.834) > Heavy knitted cotton mask (0.808) > Surgical mask (0.778) > Cotton mask-2 layers (0.744) > Nylon fabric mask-2 layers (0.740) > T-shirt fabric mask-2 layers (0.708) > T-shirt fabric mask-1 layer (0.648). The 6-layer N95 mask’s material assembly outperformed among all other masks in each size bin. The filtration efficiency of the t-shirt material masks (both single and double layers) were found to be significantly lower than other masks (p < .05). It was also observed that the heavy knitted cotton mask’s material offered greater filtration efficiency than the similarly configured standard cotton mask. Without a valve, the performance of the N95 mask’s material was better than the one with a valve. Also, the performance of the N95 mask’s material with a valve was similar to the KN95 mask’s material (p = .92). Similar results were also observed for cotton and nylon masks’ material with two layers.
Once, the filtration efficiencies of the unused facemasks’ materials were evaluated, the facemasks were washed and re-tested. presents the results of filtration efficiencies through the materials of the facemasks post washing.
Figure 4. Filtration efficiencies of the commercial facemasks’ materials (post washing with detergent in hot water)
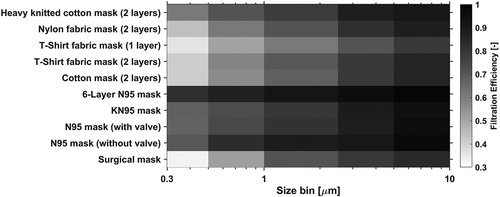
The percentage drop in average filtration efficiencies through the materials of the facemasks after being washed are as follows: 6-Layer N95 mask: 3% (p = .88), N95 mask – without valve: 2% (p = .79), KN95 mask: 4% (p = .65), N95 mask -with valve: 3% (p = .77), Heavy knitted cotton mask: 4% (p = .71), Surgical mask: 18% (p = .31), Cotton mask-2 layers: 11% (p = .48), Nylon fabric mask-2 layers: 6% (p = .67), T-shirt fabric mask-2 layers: 6% (p = .73), T-shirt fabric mask-1 layer: 8% (p = .65). The maximum drop in filtration efficiency was observed for the surgical mask’s material. However, across all the masks, the reductions in their materials’ filtration efficiencies were not statistically significant. Additionally, it was observed that post washing, the filtration efficiency of most sub-micron particles fell greater than compared to the filtration efficiency of most super-micron particles.
Discussion and conclusion
Using the aerosolized chamber experiments, the major inferences drawn from this study are:
The choice of facemask is greatly driven by the size of viable respiratory droplets that need to be eliminated. If droplets with particle size less than 0.5 µm are required to be filtered, N95 masks without the valve or more layers are preferred. If the primary objective is to filter particles between 0.5–1 µm, then N95 (both with or without valves) or KN95 masks are recommended. Surgical masks and heavy knitted cotton masks may also be used for this purpose, but with caution.
Most of the facemasks’ materials work well for filtering super-micron particles, barring single-layer masks.
Increasing the knitting stitches in cotton masks tends to improve its filtration efficiency through the material.
Upon single wash, the reductions in filtration efficiencies through the materials of the tested commercial facemasks were not statistically significant. The 6-Layer N95 mask, N95 mask – without valve, KN95 mask, N95 mask -with valve, and Heavy knitted cotton mask exhibited a drop in materials’ filtration efficiencies by less than 5%. Nylon fabric mask-2 layers, T-shirt fabric mask-2 layers, T-shirt fabric mask-1 layer were marked with a reduction in materials filtration efficiencies between 5–10%. For both Surgical mask and Cotton mask-2 layers, the reductions in materials filtration efficiencies were greater than 10%. The highest reduction was observed for surgical masks’ material. Further laboratory evaluations are encouraged to determine the maximum number of times these masks may be washed before drastically reducing their filtration efficiencies.
In relevance with the current ongoing COVID-19 pandemic, the literature suggests that droplet particles containing SARS-CoV-2 RNA mostly occur in two size ranges: 0.25–1 µm and >2.5 µm (Lindsley et al. Citation2020). Therefore, recommendation for the usage of a mask as obtained from this study is as follows: Multi-layered N95 masks > N95 masks without valve > KN95 or N95 with valve masks > Surgical masks > Properly knitted cotton masks (minimum two layers). However, it is important to note that the findings of this study cannot be generalized to pathogens for which the human-generated particle size distributions are not well characterized. But, if the human-generated particle size distribution of a pathogen is known (in the size range of 0.3–10 µm), the study provides evidence-based selection of appropriate facemask for protection.
There are certain limitations that need to be addressed for this study. Firstly, the pressure drop across facemasks during filtration is not evaluated in this analysis. Second, it was not possible to perform the analysis at finer bin widths due to the instrumentation limitation of CEM DT-9880. Nevertheless, the study attempted to make the readers aware of the filtration performance through the materials of the commonly available commercial masks. However, the results of the study must be interpreted with caution. The reason for characterizing filtration only by material and not quantifying the overall protection is that overall protection is influenced by masks’ fit, which is a subjective parameter. Incorporating subjective parameters into the experiment would have complicated comparing the results across different facemasks (since it is difficult to isolate the particles downstream that have penetrated the filtration layer from those escaped around the edges). Therefore, considering particle bypass around the edges of the facemasks, the overall protection from the facemasks would tend to be lesser than the values reported in the study. To ensure adequate safety, masks must be worn that fit properly, or, as reported in a recent article, mask frames can be used to increase the efficiency of inward protection (Tong et al. Citation2021).
Supplemental Material
Download MS Word (1.6 MB)Acknowledgment
The authors would like to thank the Director, CSIR-IMMT and Head, Environment and Sustainability Department for their support to undertake this study. ISRO-GBP-ATCTM project is acknowledged for financial support.
Disclosure statement
No potential conflict of interest was reported by the author(s).
Supplementary material
Supplemental data for this paper can be accessed on the publisher’s website.
Additional information
Funding
Notes on contributors
Satya S. Patra
Satya S. Patra is currently a Ph.D scholar in the Lyles school of Civil Engineering, Purdue University. His research interest lies in understanding the physical, chemical and biological aspects of airborne particles in indoor as well as outdoor environments. Additionally, he is passionate about development and evaluation of low cost sensors.
Jyotishree Nath
Jyotishree Nath is pursuing Ph.D. in Environment and Sustainability Department at CSIR- Institute of Minerals and Materials Technology, Bhubaneswar, Odisha, India. Her research interest is studying the tropospheric microbiome and its emission and transportation sources in ambient aerosol and its impact on human health.
Subhasmita Panda
Subhasmita Panda is a postgraduate in Chemistry and currently pursuing Ph.D. at CSIR-Institute of Minerals and Material Technology, Bhubaneswar, Odisha, India. Her major research focus is to understand the chemical characterstics of ambient aerosols and how they relate to atmospheric chemistry, climate, air quality and human health.
Trupti Das
Trupti Das is a microbiologist by training and has worked extensively on development of sustainable processes for biological treatment of industrial and municipal wastewater. A part of her research pursuit also includes the study of atmospheric aerosols and trace gases and their overall impact on the regional atmosphere. The recent focus is on monitoring and characterization of ambient aerosols to determine their composition, source and fate in the atmosphere, to understand the spatial temporal variation, transport pathways using measurement and remote sensing data. Impact of fine particulate matter and ozone on human health as well as on plant growth and productivity are some new initiatives.
Boopathy Ramasamy
Boopathy Ramasamy is a Ph.D in Chemical Engineering-Environmental Technology, currently working as a Scientist in Environment & Sustainability Department, CSIR-Institute of Minerals and Materials Technology, Bhubaneswar, Odisha, India. His major thirst area are advanced oxidation process for the removal of pollutants in water and wastewater, design and development of novel bioreactors and sustainable composite materials for the pollutants removal. Also having knowledge and interest on physical and chemical characterization of ambient aerosol (Indoor and outdoor) and trace gases.
References
- Cao, G., S. Liu, B. E. Boor, and A. Novoselac. 2015. Characterizing the dynamic interactions and exposure implications of a Particle-Laden Cough Jet with different room airflow regimes produced by low and high momentum jets. Aerosol Air Qual. Res. 15 (5):1955–66. Taiwan Association for Aerosol Research. doi:https://doi.org/10.4209/aaqr.2015.03.0146.
- Dbouk, T., and D. Drikakis. 2020. On respiratory droplets and face masks. Phys. Fluids 32 (6):63303. American Institute of Physics. doi:https://doi.org/10.1063/5.0015044.
- Fischer, E. P., M. C. Fischer, D. Grass, I. Henrion, W. S. Warren, and E. Westman. 2020. Low-cost measurement of face mask efficacy for filtering expelled droplets during speech. Sci. Adv. 6 (36):eabd3083. doi:https://doi.org/10.1126/sciadv.abd3083.
- Gibson, E. R., P. K. Hudson, and V. H. Grassian. 2006. Aerosol chemistry and climate: Laboratory studies of the carbonate component of mineral dust and its reaction products. Geophys. Res. Lett. 33 (13). John Wiley & Sons, Ltd. doi:https://doi.org/10.1029/2006GL026386.
- Hansson, H.-C., M. J. Rood, S. Koloutsou-Vakakis, K. Hämeri, D. Orsini, and A. Wiedensohler. 1998. NaCl aerosol particle hygroscopicity dependence on mixing with organic compounds. J. Atmos. Chem. 31 (3):321–46. doi:https://doi.org/10.1023/A:1006174514022.
- Hinds, W. C. 1999. Aerosol technology: Properties, behavior, and measurement of airborne particles. New York, NY: John Wiley & Sons.
- Ilyashenko, I., S. Kovshov, and E. Navitskaite. 2019. Assessment of the aerotechnogenic situation in the city of St. Petersburg based on instrumental measurements of air dustiness and computer modeling of its distribution. J. Ecol. Eng. 20 (4):150–56. doi:https://doi.org/10.12911/22998993/102808.
- Johnson, G. R., L. Morawska, Z. D. Ristovski, M. Hargreaves, K. Mengersen, C. Y. H. Chao, M. P. Wan, Y. Li, X. Xie, D. Katoshevski, et al. 2011. Modality of human expired aerosol size distributions. J. Aerosol Sci 42 (12):839–51. doi:https://doi.org/10.1016/j.jaerosci.2011.07.009.
- Lee, K. W., and B. Y. H. Liu. 1980. On the minimum efficiency and the most penetrating particle size for fibrous filters. J. Air Pollut. Control Assoc. 30 (4):377–81. Taylor & Francis. doi:https://doi.org/10.1080/00022470.1980.10464592.
- Lindsley, W. G., F. M. Blachère, N. C. Burton, B. Christensen, C. F. Estill, E. M. Fisher, S. B. Martin, K. R. Mead, J. D. Noti, and M. Seaton. 2020. COVID-19 and the workplace: Research questions for the aerosol science community. Aerosol Sci. Technol. 54 (10):1117–23. Taylor & Francis. doi:https://doi.org/10.1080/02786826.2020.1796921.
- Mahdavi, A. 2013. Efficiency measurement of N95 filtering facepiece respirators against ultrafine particles under cyclic and constant flows. Montreal, Quebec: Concordia University.
- Manjarrez-Zavala, M. E. 2013. Pathogenesis of viral respiratory infection. In, Respiratory Disease and Infection-A new insight, ed. D. P. Rosete-Olvera, Ch. 1. Rijeka: IntechOpen. doi:https://doi.org/10.5772/54287.
- Patra, S. S., B. R. Chilukuri, and L. Vanajakshi. 2021. Analysis of road traffic pattern changes due to activity restrictions during COVID-19 pandemic in Chennai. Transp. Lett.:1–9. March. Taylor & Francis. doi:https://doi.org/10.1080/19427867.2021.1899580.
- Patra, S. S., R. Ramsisaria, R. Du, T. Wu, and B. E. Boor. 2021. A machine learning field calibration method for improving the performance of low-cost particle sensors. Build. Environ. 190:107457. doi:https://doi.org/10.1016/j.buildenv.2020.107457.
- Petters, M. D., and S. M. Kreidenweis. 2007. A single parameter representation of hygroscopic growth and cloud condensation nucleus activity. Atmos. Chem. Phys. 7 (8):1961–71. doi:https://doi.org/10.5194/acp-7-1961-2007.
- Richard, M., J. M. A. van den Brand, T. M. Bestebroer, P. Lexmond, D. de Meulder, R. A. M. Fouchier, A. C. Lowen, and S. Herfst. 2020. Influenza A viruses are transmitted via the air from the nasal respiratory epithelium of ferrets. Nat. Commun. 11 (1):766. doi:https://doi.org/10.1038/s41467-020-14626-0.
- Sousan, S., K. Koehler, L. Hallett, and T. M. Peters. 2016. Evaluation of the Alphasense optical particle counter (OPC-N2) and the Grimm portable aerosol spectrometer (PAS-1.108). Aerosol Sci. Technol. 50 (12):1352–65. doi:https://doi.org/10.1080/02786826.2016.1232859.
- Stadnytskyi, V., C. E. Bax, A. Bax, and P. Anfinrud. 2020. The Airborne lifetime of small speech droplets and their potential importance in SARS-CoV-2 transmission. Proc. Natl. Acad. Sci. 117 (22): 11875 LP–11877. doi:https://doi.org/10.1073/pnas.2006874117.
- Steinbrook, R. 2020. Filtration efficiency of face masks used by the public during the COVID-19 pandemic. JAMA Intern. Med. December. doi:https://doi.org/10.1001/jamainternmed.2020.8234.
- Tong, Y., J. Pan, E. Kucukdeger, A. L. Johnson, L. C. Marr, and B. N. Johnson. 2021. 3D printed mask frames improve the inward protection efficiency of a cloth mask. ACS ES&T Eng. 1:1000–08. April. American Chemical Society. doi:https://doi.org/10.1021/acsestengg.1c00028.
- Verma, S., M. Dhanak, and J. Frankenfield. 2020. Visualizing the effectiveness of face masks in obstructing respiratory jets. Phys. Fluids 32 (6):61708. American Institute of Physics. doi:https://doi.org/10.1063/5.0016018.
- Whiley, H., T. P. Keerthirathne, M. A. Nisar, M. A. F. White, and K. E. Ross. 2020. Viral filtration efficiency of fabric masks compared with surgical and N95 masks. Pathogens 9:762. doi:https://doi.org/10.3390/pathogens9090762.