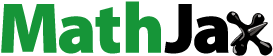
ABSTRACT
Sampling and analysis of filterable particulate matter (FPM), FPM2.5, condensable particulate matter (CPM), polycyclic aromatic hydrocarbons (PAHs), sulfur oxides (SOx), and nitrogen oxides (NOx) emitted from a coal-fired boiler equipped with selective catalytic reduction (SCR)+ electrostatic precipitator (ESP) + wet flue gas desulfurization (WFGD) + wet electrostatic precipitator (WESP) as air pollution control devices (APCDs) are conducted. The results show that NOx concentration emitted from the coal-fired boiler is 56 ± 2.17 ppm (with the NOx removal efficiency of 47.2%), which does not meet the best available control technology (BACT) emission standard (≤ 30 ppm). On the other hand, the WFGD adopted has a good removal efficiency for SOx and HCl. Both SOx and HCl emission concentrations are < 1 ppm, and removal efficiencies are > 99%. The FPM and FPM2.5 emitted from the coal-fired boiler are 0.9 ± 0.06 mg/Nm3 and < 0.09 ± 0.006 mg/Nm3, respectively. The overall removal efficiency of FPM achieved with ESP+WFGD+WESP+MGGH is 99.98%. However, high concentration of CPM (37.4 ± 6.3 mg/Nm3) is measured, which is significantly higher than FPM and FPM2.5. The concentrations of 27 PAHs at the WESP inlet and stack are measured as 667 ng/Nm3 and 547 ng/Nm3, respectively while the removal efficiencies of gas- and solid-phase PAHs are 9% and 58%, respectively. The results show that APCDs adopted are not effective in removing PAHs (only 18%), and gas-phase PAHs contribute the most in the total PAH emission. In addition, the benzo(a)pyrene equivalent (BaPeq) concentration emitted from the stack is 28.8 ng-BaPeq/Nm3, and most of it is contributed by 4–6 ring PAHs with high toxic equivalent factors (TEFs). Furthermore, the emission factors of air pollutant emitted from coal-fired boilers equipped with different combinations of APCDs are compiled and compared. The results show that except for CPM and NOx, the emission factors of air pollutant calculated for this coal-fired boiler are lower if compared with other studies.
Implications: Primary particles discharged from coal-fired processes include filterable particulate matter (FPM) and condensable particulate matter (CPM). PM2.5 emissions would be greatly underestimated if CPM is ignored. Polycyclic aromatic hydrocarbons (PAHs) are semi-volatile organic compounds (SVOCs) formed by two or more fused benzene rings. PAHs have attracted much public attention because of toxicity and carcinogenicity. This study selects one coal-fired boiler with the best available control technology (BACT) to simultaneously measure the concentrations of PM, PAHs, and gaseous pollutants at the inlet and outlet of air pollution control devices (APCDs) to understand the efficacy of APCDs adopted and pollutant emission intensity.
Introduction
Fossil fuels, including coal, oil, and natural gas, account for about 86% of the world’s primary energy consumption (Feng, Li, and Cui Citation2018). Among them, coal plays an important role in the energy generation and consumption structure. Coal is mainly used by industrial boilers and coal-fired power plants to generate steam and electricity. Significant amounts of particulate matter (PM), sulfur oxides (SOx), nitrogen oxides (NOx) and polycyclic aromatic hydrocarbons (PAHs) are emitted from the coal combustion process, leading to the degradation of air quality. In the atmospheric environment, the reaction between different gaseous precursors, such as NH3, NOx and SOx results in the formation of secondary pollutants, such as PM and photochemical smog. Epidemiologic study has confirmed that PM adsorbs harmful substances such as heavy metals, organic compounds and acid gases (Li et al. Citation2017). In particular, particles with the aerodynamic diameter less than or equal to 2.5 μm (PM2.5) have attracted great attention because the fine particles inhaled into the bronchus may cause respiratory disease, affecting cardiopulmonary health and increasing the risk of lung cancer. Previous studies indicate that coal-fired activities are major contributors of PM, gaseous pollutants and persistent organic pollutants (POPs) in the vicinity (Chen et al. Citation2019; Corio and Sherwell Citation2000; Hsu et al. Citation2016).
Primary particles discharged from industrial processes include filterable particulate matter (FPM) and condensable particulate matter (CPM). FPM refers to solid or liquid particles directly discharged from flue gas and can be captured by the filter of the stack sampling train. According to the U.S. EPA, CPM is in vapor phase under stack conditions but condenses and reacts upon cooling and dilution of the ambient air to form solid and liquid PM after exiting the stack (U.S. EPA Citation2016). Ehrlich et al. (Citation2007) measured PM10 and PM2.5 emissions from stationary sources in Germany, and the results show that PM10 and PM2.5 accounted for 90% and 40%–70% of total particulate matter (TPM), respectively. Goodarzi (Citation2006) measured FPM2.5 in the flue gas of a Canadian coal-fired power plant and showed that PM2.5 accounted for 30–50% of TPM. The results indicate that PM2.5 accounts for a significant portion of TPM emissions. Maguhn et al. (Citation2003) measured the concentration of pollutants in the exhaust of municipal solid waste incinerators (MWIs), indicating that the concentration of fine particles increased with increasing SOx concentration, and both emissions are highly correlated. Moreover, CPM exists in the form of gaseous substances, which can be divided into inorganic components including SO4−2, NO3− and NH4+, and organic components such as alkanes and aromatic compounds before being emitted into the atmosphere (Wang et al. Citation2020). Previous study indicates that SO2 reacts with water and ammonia escaping from SCR to form SO4−2 and NH4+, and both may form CPM due to temperature change (Farber and Sloat Citation2005). Thus, removal efficiencies of SOx and NOx achieved with existing APCDs not only affect the emissions of SOx and NOx but also CPM. Once being discharged into the atmosphere, both FPM and CPM contribute to haze problems (Yang et al. Citation2017). On the other hand, the difference between FPM and CPM emission rates is significant. Zheng et al. (Citation2018) studied the PM2.5 emission characteristics of a circulating fluidized bed boiler (CFB), indicating CPM contributed 76.7% of PM2.5 emission. Yang et al. (Citation2018) investigated the PM2.5 emission characteristics of four coal-fired boilers, and the results showed that the CPM of the four boilers accounted for 54.3%, 50.7%, 71.1% and 87.7% of PM2.5, respectively. However, CPM emissions from stationary sources are not regulated. Overall, PM2.5 emissions would be greatly underestimated if CPM is ignored because CPM accounts for a significant portion of total PM2.5 emission (Corio and Sherwell Citation2000; Yang et al. Citation2015, Citation2014).
PAHs are semi-volatile organic compounds (SVOCs) including two or more fused benzene rings. Most PAHs are emitted from incomplete combustion of fossil fuels. PAHs may exist in gas phase and solid phase, depending on their molecular weight (Ravindra, Sokhi, and Van Grieken Citation2008). PAHs have attracted much public attention because of toxicity, carcinogenicity and genotoxicity (Cancer Citation1987). Sixteen PAHs have been listed as priority control pollutants by the U.S. EPA.
In order to effectively improve air quality, Taiwan’s Air Pollution Prevention and Control Law strictly restricts the emissions from large stationary sources and requires them to meet the best available control technology (BACT) standards, with PM and SOx or NOx being less than or equal to 10 mg/Nm3, 25 ppm, and 30 ppm, respectively. The purpose of this study is to better understand the air pollution emission characteristics of a coal-fired process and check to see if it meets Taiwan’s BACT standards. This study selects one coal-fired boiler equipped with BACT to measure the concentration of particulate matters (FPM, FPM2.5, CPM), polycyclic aromatic hydrocarbons (PAHs) at the inlet and outlet of air pollution control equipment (APCDs) simultaneously to understand the efficacy of APCDs adopted and pollutant emission intensity. In addition, the concentrations of sulfur oxides (SOx) and nitrogen oxides (NOx) were measured to evaluate the performance of WFGD and SCR adopted. At the same time, the emission factors of air pollutants are updated.
Experimental methods
Sampling information
In this study, a coal-fired boiler located in central Taiwan was selected for flue gas sampling to evaluate the removal efficiencies of FPM, FPM2.5, CPM, PAHs, SOx and NOx achieved with existing air pollution control devices (APCDs). shows the process of APCDs adopted in this coal-fired boiler. Australian coal was applied as the fuel and the proximate analysis data indicated that volatile matter, ash, fixed carbon, sulfur content and lower heating value (LHV) were 34.0%, 15.7%, 47.8%, 0.71%, and 6,726 kcal/kg, respectively. The APCDs adopted include selective catalytic reduction (SCR), electrostatic precipitator (ESP), wet flue gas desulfurization (WFGD), and wet electrostatic precipitator (WESP). After removing NOx with SCR, the flue gas passes through the medium gas–gas heater (MGGH) to recover the heat and to reduce the temperature of flue gas entering the electrostatic precipitator (ESP) to improve the removal efficiency of PM. After passing through ESP, the flue gas is introduced into the WFGD for removing SOx. The last device is WESP for the final capture of particulate matter, and then the temperature of the flue gas is raised by MGGH to prevent white plume. The flue gas sampling points include SCR inlet, SCR outlet, WESP inlet and stack. The concentrations of gaseous pollutants including NOx and SOx are measured at the SCR inlet and outlet, WESP inlet and stack, while FPM samplings are conducted at the SCR outlet, WESP inlet and stack simultaneously. PAHs samplings are carried out at the WESP inlet and stack. Additionally, FPM2.5 and CPM measurements are conducted simultaneously at the stack. The concentrations of pollutant reported are all corrected with an oxygen content of 6%.
Sampling method
FPM2.5 sampling and analysis
The sampling of FPM2.5 was conducted according to the U.S. EPA Method 201A “Determination of PM2.5 and PM10 Emissions from Stationary Sources.” The sampling equipment includes probe nozzle, PM2.5 cyclone splitter, filter holder, pitot tube and stainless steel (with glass liner) sampling probes, vacuum pump and control cabinet. The sampling system is operated by control cabinet (Autokinetic Source Sampler, APEX XC-5000) and the sampling procedure has to meet the requirements of U.S. EPA Method 201A. In order to achieve isokinetic sampling, an appropriate probe nozzle was selected. After removing the particles with the aerodynamic diameter greater than 2.5 μm by cyclone splitter (APEX, SFA-47), PM2.5 particles were collected with a 47 mm quartz filter (Tissuquartz, 2500 QAT-UP). In this study, the sampling devices for FPM2.5 and CPM were connected in series for simultaneous sampling as shown in ). Before sampling, the filter was conditioned at 20 ± 5.6°C for 24 hours and weighed to a constant weight by the gravimetric technique (Shimadzu balance, AP135W). After sampling, the sample was recovered for conditioning at 20 ± 5.6°C for 24 hours and weighing, and the result is reported with the unit of mg/Nm3 (dry base standard gas volume).
CPM sampling and analysis
Sampling of condensable particulate matter (CPM) was conducted according to the U.S. EPA Method 202 “Dry Method for Determining Condensable Particulate Emissions from Stationary Sources.” The sampling devices for FPM2.5 and CPM were connected in series with the U.S. EPA method 201A and 202, respectively, for simultaneous sampling as shown in ). After FPM2.5 was collected by a 47 mm quartz filter (Tissuquartz, 2500 QAT-UP), the CPM sample was collected by the condenser, impingers, and a teflon filter (Advantec, J020A090C PTFE). After sampling, the contents of the impingers and the filter were purged with nitrogen at a constant flow rate of 14 L/min for 1 hour to remove dissolved SO2. Thereafter, deionized water and n-hexane (Merck, 98%) were used to rinse the inorganic (water-soluble) and organic (organic-solvent-soluble) components, respectively, in the sampling tube, the connected glass tube, impact bottle and filter clamp. The water-soluble and organic-solvent-soluble samples are named as inorganic and organic fractions, respectively, in Method 202. The water-soluble, organic-solvent-soluble, and the CPM filter were collected in clean containers, respectively, dried and weighed in the laboratory. CPM is the summation of the water-soluble, organic-solvent-soluble fractions. The analysis procedures can be referred to the U.S. EPA Method 202.
PAHs sampling and analysis
) shows the flue gas sampling assembly for polycyclic aromatic hydrocarbons (PAHs). PAHs in the stack gas were sampled according to the US EPA Method 23A. The flue gas sampling assembly includes front nozzle, stainless probe, filter holder, condenser, XAD-2 adsorbent, and impinger. After flue gas removes moisture, the flue gas passes through the 90 mm quartz filter (Tissuquartz, 2500 QAT-UP) to collect solid-phase PAHs, and through the condenser to capture the remaining gas-phase PAHs with XAD-2 (Aencore) adsorbent. The sampling system is operated by Autokinetic Source Sampler (APEX XC-5000) and the sampling meets the requirements of US EPA Method 23A.
The filter containing solid-phase PAHs and the XAD-2 resin containing gas-phase PAHs were extracted for 22 ± 2 hours with dichloromethane (Fisher, 99.9%). An isotope-spiked solution of PAHs was added before extraction to quantify the concentration of each PAH congener. The dichloromethane extract was then concentrated to approximately 2 mL by rotary evaporation and replaced by 8 mL of hexane. The sample was then passed through a cleanup column packed with activated silica gel. The column was eluted to obtain PAHs in the purifying solution and condensed to 5 mL by rotary evaporation. The collected eluent was concentrated to 450 μL with nitrogen flow. The samples were then added with recovery standards and analyzed by gas chromatography/mass spectrometry (GC/MS) (Thermo Fisher Scientific, Double Focusing Sector Mass Spectrometer) using a fused silica capillary column DB-5 MS (60 m × 0.25 mm×0.25 μm) under selected ion monitoring (SIM) mode. In total 27 PAHs including 16 U.S. EPA PAHs, 15 E.U. PAHs, and three additional PAHs are analyzed in this study. Detailed information regarding these PAHs including ring number and TEF values are presented in Table S1 of the Supporting Information. The toxic equivalency factors (TEFs) are used to calculate the toxic equivalent concentration of PAHs based on the toxicity of BaP (BaPeq). It is used to assess the carcinogenic risk of PAHs, and the TEFs published in previous studies are adopted. BaPeq of the PAHs is calculated according to EquationEquation (1)(1)
(1) .
where PAHi is the concentration of the individual PAH in the sample and TEFi is the TEF of individual PAH.
Flue gas composition analysis
After particle matter was removed via 90 mm quartz filter (Tissuquartz, 2500 QAT-UP), water vapor was removed from the gas stream by silica gel and the concentrations of gaseous pollutants including O2, CO, CO2, NOx, and SO2 were measured by a flue gas analyzer (Testo 350, Germany).
Emission factor
The emission factor (EF) is calculated based on the concentration of pollutant, gas flow rate and coal feeding rate. The EF of pollutant (g/ton of coal) is a key parameter for the calculation of pollutant emitted from coal-fired boiler. It is defined as the emission of air pollutants into the atmosphere caused by the industrial activity of the emission source. The factor can be used to calculate the total emission of air pollutants in the area, so as to propose appropriate control and improvement measures to achieve the goal of maintaining air quality in the vicinity. The EF is calculated based onEquationEquation (2)(2)
(2) (Dat et al. Citation2020):
where C is the concentration of pollutant emitted during testing (mg/Nm3), while Q is the gas flow rate (Nm3/hr).
Results
Characteristics of gaseous pollutants and operating parameters
shows the flue gas composition of the coal-fired boiler investigated, including moisture content, oxygen content, CO, CO2, NOx, SO2, HCl, and NH3 concentrations. The results indicate that the moisture, O2 and CO2 contents measured at the stack are 13 ± 1.39%, 6.5. ± 0.30% and 13 ± 0.07%, respectively. The CO concentrations measured at the SCR inlet and stack are 6.6 ± 0.71 and 4.8 ± 0.61 ppm, respectively.
Table 1. Concentration of gaseous pollutants measured at various sampling locations
SCR has been recognized as the best available control technology for NOx, and the typical commercial SCR process can remove 80–90% of NOx (Forzatti Citation2001). Under low boiler load conditions in conventional coal-fired units, the flue gas temperature at SCR inlet may not be within the optimum reaction temperature range (320–400°C) for high DeNOx efficiency (Ruan et al., Citation2019a). Sampling results show that the NOx concentration at the SCR inlet is 108 ± 3.39 ppm (145 mg/Nm3). After being treated by SCR, NOx concentration is reduced to 56 ± 2.17 ppm (75 mg/Nm3), indicating that the NOx removal efficiency achieved with SCR is 47.2%. It shows that the expected performance of 80% removal is not achieved and that the NOx concentration emitted is above the BACT emission standard (≤ 30 ppm). Ruan et al. (Citation2019b) investigated the SCR performance of an ultra-low emission coal-fired boiler in China and the results indicated that NOx concentrations measured at the SCR inlet and outlet were 165 and 24 mg/Nm3, respectively, with the NOx removal efficiency of 86%. Wu et al. (Citation2018) measured NOx emitted from four ultra-low coal-fired power plants and the results indicated that NOx emissions were in the range of 29–34 mg/Nm3. Low NOx removal is related to the operating conditions of SCR, such as temperature and NH3/NOx ratio. Additionally, SO3 reacts with water vapor present in flue gas and with ammonia to form sulfuric acid and ammonium sulfates, which may deposit onto the catalyst, reducing the catalytic activity (Forzatti Citation2001). Hence, long-term use of the catalyst without proper maintenance may decrease the activity, resulting in lower NOx removal efficiency than the original design value (80%). The plant is required to regularly inspect the SCR equipment and catalytic activity to ensure the NOx removal efficiency is above 80%. The results of acid gases (SO2, HCl) measurements show that the concentration of SO2 at the SCR inlet is 323 ± 4.59 ppm (923 mg/Nm3). After the passing through SCR, the SO2 concentration is reduced to 284 ± 19.2 ppm (811 mg/Nm3). Ren et al. (Citation2020) investigated the emission of sulfur trioxide from coal-fired power plants, indicating that commercial SCR catalyst causes the formation of SO3 (SO2 → SO3). Thus, the SCR catalyst causes the oxidation of some SO2 into SO3. After passing through the WFGD system, SO2 concentration is reduced to < 1 ppm (3 mg/Nm3), and the removal efficiency of SO2 is ≥ 99%, indicating that the WFGD has a good performance. Wu et al. (Citation2018) measured SOx emission from an ultra-low coal-fired power plants, and the results showed that SOx concentration measured at the WFGD inlet was 1597 mg/Nm3. After passing through WFGD, the SOx concentration was reduced to 14 mg/Nm3, with the removal efficiency of 99.1%. In addition, the HCl concentration measured at SCR outlet is 16.8 ± 0.34 ppm. After WFGD, the HCl concentration is reduced to < 1 ppm. The acid gases are considered as important precursors of CPM because they easily react with limestone/lime in the desulfurization slurry and is absorbed by the droplets in the FGD to form gypsum rain, which further increases the emission of fine particles. In addition, the NH3 concentration measured at the stack is also < 1 ppm, indicating that ammonia escaped from SCR can be efficiently removed by WFGD and WESP.
Emission characteristics and distribution of particulate matter
Emission of FPM, FPM2.5 and CPM
shows the FPM concentrations measured at three sampling points. The results show that the PM concentration measured at the SCR outlet is 5,341 ± 264 mg/Nm3. After passing through the ESP, the FPM concentration decreases to 8.5 ± 0.75 mg/Nm3, ESP has 99.8% removal efficiency for FPM. Finally, after passing through WESP, the FPM emitted from the stack is further reduced to 0.9 ± 0.06 mg/Nm3. The WESP has 90% removal efficiency for FPM. Overall, the removal efficiency of FPM achieved with ESP+WFGD+WESP+MGGH is up to 99.98%, demonstrating that existing APCDs have a good overall removal efficiency for FPM. Li et al. (Citation2017) studied the FPM emission from an ultra-low emission coal-fired power plant with the APCDs (SCR+ESP+WFGD+WESP) adopted being similar to this study. The results show that the FPM concentrations measured at SCR outlet and stack are 2,485 and 1.6 mg/Nm3, respectively, which are close to the values obtained in this study.
shows the FPM, FPM2.5 and CPM concentrations measured at the coal-fired boiler. The results indicate that FPM, FPM2.5 and CPM concentrations emitted from the stack are 0.9 ± 0.06, <0.09 ± 0.006 and 37.4 ± 6.3 mg/Nm3, respectively. As far as FPM and FPM2.5 emissions are concerned, the APCDs adopted by the coal-fired boiler have a good overall removal efficiency for fine particles under normal operating conditions. In terms of CPM emission, the CPM emitted from this coal-fired boiler is much higher than the concentration of FPM. Therefore, neglect of CPM emission will greatly underestimate the emission of particulate matter. Table S2 shows the comparison of FPM2.5 and CPM emissions reported in various studies. The results show that the FPM concentrations measured in this study are relatively low compared to the previous studies. In particular, the concentration of fine particles (FPM2.5) emitted is 0.09 ± 0.006 mg/Nm3. On the other hand, the CPM concentration is relatively high compared to previous studies. Corio and Sherwell (Citation2000) studied the CPM emission characteristics of four coal-fired boilers, and the results indicate that the average CPM concentration emitted was 37.5 mg/Nm3. Lu, Wu, and Pan (Citation2010) investigated the characteristics of PM emission from coal-fired power plants, and the average CPM emission was 35 mg/Nm3, accounting for 24.6%, 33.6%, and 56.9% of TPM, PM10, and PM2.5, respectively. Yang et al. (Citation2014) selected five different types of stationary sources to analyze CPM emissions, and the average CPM emission was 23.53 mg/Nm3. Pei (Citation2015) studied three coal-fired boilers, and the average CPM concentration was 25 mg/Nm3. In addition, Li et al. (Citation2017) studied the use of limestone in WFGD of an ultra-low emission coal-fired boiler and the results indicate that the slurry washing and flue gas carrying effects increase the SO42- mass concentration by approximately 4.2 times. As flue gas enters WESP, it causes a voltage increase, affecting the removal efficiency of CPM (Mertens et al. Citation2017). Yang et al. (Citation2014) pointed out that sulfur oxides, nitrogen oxides and water vapor of the flue gas and ammonia escaping would react to form CPM precursors including SO3, NH4+ and NO3−. As these precursors are discharged from the stack into the atmosphere, they are cooled to form CPM, depending on the ambient temperature and dilution effect (Yang et al. Citation2015).
CPM composition
shows the composition of CPM emitted from the coal-fired boiler investigated, including organic and inorganic. The results show that inorganic CPM dominates, with organic and inorganic CPM being 3.8 and 33.6 mg/Nm3, respectively. Inorganic matter is the main component contributing to the CPM emitted from this coal-fired boiler, accounting for 89.8% of CPM emitted. Corio and Sherwell (Citation2000) studied the organic and inorganic CPM emitted from four coal-fired boilers, and the results showed that the average contribution of organic to the CPM was approximately 23%. Previous studies have shown that CPM emitted from coal-fired boilers are mainly inorganic, for instance, Yang et al. (Citation2014) investigated CPM emissions from coal-fired power plants and coal-fired boilers and the results showed that inorganics accounted for 89% and 69%, respectively.
Existing particulate control devices (ESP+WESP) are found to have high overall removal efficiency for FPM2.5. However, current regulation and control strategies focus on FPM emissions and ignore the CPM concentration of flue gas. This study indicates that control of CPM concentration is also an important factor that must be considered because high CPM emissions will greatly increase the total PM2.5 emissions. In addition, most studies have shown that WESP can effectively remove fine particles, but its efficiency for CPM removal remains unclear as revealed in this study.
PAHs mass concentration distributions
shows the concentrations of 27 PAHs in gas phase and solid phase measured at WESP inlet and stack, respectively. The results show that the concentration of PAHs measured at the WESP inlet is 667 ng/Nm3. After passing through WESP+MGGH, the PAHs concentration decreases slightly to 547 ng/Nm3. In addition, the removal efficiencies of gas and solid-phase PAHs are evaluated, and the results show that the removal efficiency of solid-phase PAHs is 58%, while the removal efficiency of gas-phase PAHs is only 9%, with the overall removal efficiency of 18%.
Table 2. Gas-phase and solid-phase PAHs measured at two sampling locations of the coal-fired boiler investigated (ng/Nm3)
indicates that relatively high concentrations of 2–4 ring PAHs are measured at WESP inlet and stack, especially Nap concentrations are measured as 392 and 285 ng/Nm3, respectively. Nap is of the highest concentration among 27 PAHs species analyzed. Other important contributing species include Pyr (69 and 83 ng/Nm3), FL (34 and 60 ng/Nm3), and PA (22 and 29 ng/Nm3). After passing through WESP+MGGH, the species distribution did not change significantly. The concentrations of PAHs with LMW (light-molecular-weight) are relatively higher, and the concentration of gas-phase PAHs is much higher than that of solid-phase. The results of PAHs species distribution show that some species reveal increasing trend across the APCDs. Especially, gas-phase 3–4 ring PAHs (Ant, PA, FL, Pyr, CHR and BcFE) concentrations increase significantly (). The remaining PAHs species with relatively low increase in concentration include CYC, BbF, BkF and BjF. This trend is mainly ascribed to the fact that HMW (high-molecular-weight) PAHs are more concentrated in the fine particle mode in comparison with LMW PAHs, and 4-ring congeners also tend to be accumulated in fine particles (Zhang et al. Citation2020). On the other hand, Shen et al. (Citation2010) studied the distribution of PAHs on the particles with different sizes from coal combustions and the results show that PAHs are more associated with smaller PM than with larger PM, nearly 90% of particulate PAHs are bound to PM2.5. Xu et al. (Citation2016) investigated the impact of WESP on PM emission characteristics and the results show that WESP exhibits a good performance on the removal of PM of less than 2 μm. On the other hand, PM larger than 2 μm are effectively washed away by WESP water, some large new PM enriched in S and Na is produced in the WESP due to the slurry entrainment. Li et al. (Citation2016) studied PAHs emitted from ultra-low coal-fired power plants in China. Although WESP helps to remove fine particles, the results indicate that different WESP specifications and operating conditions result in big variation of removal efficiency. WESP is selective for the removal of PAHs of different species, and the discharge process may also affect the decomposition and synthesis of PAHs, which may cause an increase in the concentration of some PAHs species. Khakharia et al. (Citation2019) investigated the formation of aerosol nuclei within a WESP and the results indicate that as the applied voltage is increased the particle removal efficiency increases until a certain point, after which generation of new fine particles is observed. Allen et al. (Citation1996) studied the distribution of PAHs in particles and the results show that PAHs are mainly emitted in gas phase or associated with fine particles. PAHs can become associated with new particles either by the growth of fine combustion-generated particles or by volatilization from fine particles followed by condensation onto new particles. The PAHs species investigated have different physical and chemical properties such as molecular weight, vapor pressure, resulting in different removal efficiency of PAHs by WESP. Moreover, it has also been found that the fraction of PAH in the gas phase increases with temperature (Allen et al., Citation1996). In addition, the concentrations of some PAHs at the WESP inlet are relatively low, which might reach a certain limit for PAH scavenging. As for the solid-phase PAHs, only Nap concentration increases, but the level is not significant. However, Nap is one of the most volatile PAH. Nap has the highest vapor pressure in PAHs species and tend to be associated with the gas phase. At the same time, Nap is known as a common and widespread presence in air. Solid-phase PAHs are easily affected by Nap in atmosphere, and their distribution changes through adsorption and volatilization reactions (Abdel-Shafy and Mansour Citation2016; Jia and Batterman Citation2010). In addition, the results show that 6-ring PAHs species including DBalP, DBP, DBaiP, and DBahP were not detected at both APCD inlet and outlet.
shows the distributions of gas- and solid-phase PAHs in the flue gas. The results indicate that the distribution of gas-phase PAHs at the WESP inlet is significantly higher than that of solid-phase PAHs. Gas-phase and solid-phase PAHs account for 81% and 19% of total PAHs at the WESP inlet, respectively. After passing through WESP+MGGH, the distribution of gas-phase PAHs increased to 90%. It shows that the removal efficiency of WESP+MGGH for gas-phase PAHs is limited, while solid-phase PAHs can be effectively removed by WESP+MGGH. shows the distribution of 2–6 ring PAHs at the WESP inlet and stack, respectively. The results indicate that gas-phase PAHs are mainly composed of 2–3 rings (75%), while 2–3 ring PAHs account for 27% of solid-phase PAHs at the WESP inlet. Obviously, LMW PAHs account for the majority of the gas-phase PAHs, while HMW PAHs account for the majority of the solid-phase. After passing through WESP+MGGH, the contribution of 2–3 ring PAHs to gas-phase PAHs decreased to 61%, and the remaining 4–6 rings increased to 39%, while 4-ring PAHs increased to 18% in gas-phase. For the solid-phase PAHs, the contribution of 2–3 ring PAHs increased to 43%, while 4–6 ring PAHs decreased to 57%. The results show that existing APCD has a poor removal efficiency for 2–4 ring PAHs and some PAHs species even increase in concentration. Wang et al. (Citation2016) studied the emission of PAHs in size-segregated PM from coal-fired power plants, and the results show that size-segregated PM2.5–10 of 4-ring PAHs has a distribution of 35.8–49.3% (this study is 32%), and the lighter species, more readily equilibrate between fine and bulk particles. Wang et al. (Citation2018) studied the emission characteristics of gas- and solid-phase PAHs from coal-fired boiler, indicating that gas-phase PAHs may transform into solid-phase because the partitioning of gaseous and particulate PAHs is not in-equilibrium. Thus, gas-phase PAHs may condense into preexisting particles in the process of cooling of flue gas, which indirectly leads to an increase in the LMW percentage of solid-phase PAHs. On the other hand, some species of solid-phase PAHs with higher vapor pressure are converted into gas-phase PAHs in the process of flue gas heating such as MGGH, resulting in the increase of the gas-phase PAHs.
Benzo(α)pyrene (BaP) is a carcinogen and is widely used as a reference compound for the health risk assessment associated with PAHs. Toxic equivalency factor (TEFs) is the value assigned to each PAH species relative to the toxicity of BaP. shows the TEQ distribution of 27 PAHs measured at this coal-fired boiler. The results show that the BaPeq toxic concentrations measured at the WESP inlet and stack are 39.7 and 28.8 ng-BaPeq/Nm3, respectively (). The removal efficiency of PAHs achieved with WESP+MGGH is 28% based on the TEQ concentrations measured at WESP inlet and stack, respectively. Among 27 PAHs investigated, BcFE (28.1 and 21.6 ng-BaPeq/Nm3, respectively), BaP (6.7 and 1.7 ng-BaPeq/Nm3) and BjF (0.7 and 1.6 ng-BaPeq/Nm3) make most contributions. It is attributed to the high TEFs of these three species, being 20, 1 and 0.3, respectively. The TEFs of MMW (medium-molecular-weight) and HMW PAHs are significantly higher than those of LMW PAHs (100–20,000 times of 2–3 ring PAHs). For the species distribution, the mass concentrations of MMW and HMW PAHs are much lower than that of LMW PAHs, but the overall toxicity contributions are much higher than that of LMW PAHs. Therefore, the removal efficiency of particles should be improved to further reduce the emission of solid-phase PAHs, especially the particles with large specific surface areas (such as PM1.0). On the other hand, the removal efficiency of gas-phase PAHs achieved with WESP+MGGH is only 9%, and the TEQ removal efficiency is even negative. Although WESP helps remove fine particles, WESP is selective for PAHs removal, which may affect the decomposition and synthesis of PAHs, causing the increase of some PAHs species, especially 3–5 rings, thereby affecting the removal of TEQ concentration.
Emission factors of pollutants from coal-fired boilers
lists the emission factors (EFs) of pollutants based on the concentrations measured at the stack and operating parameters. Emission factors of various pollutants from different coal-fired boilers in various countries are also compiled and compared. The gas flow rate of the coal-fired boiler investigated in this study is measured as 300,600 Nm3/hr, and the coal feeding rate is 24.7 ton/hr. The results show that the emission factors of FPM, FPM2.5 and CPM in this coal-fired boiler are 11, 1.09 and 581 g/ton, respectively, indicating that CPM emission is 52.8 times that of FPM. The overall results show that this coal-fired boiler has a lower emission factor of FPM if compared with other studies, but CPM emission is relatively high.
Table 3. Emission factors of various pollutants (g/ton of coal) from coal-fired boilers
Within the coal-fired boiler, oxidation of sulfur and nitrogen in the fuel causes the formation of sulfur oxides (SOx) and nitrogen oxides (NOx). Atmospheric transport and reactions lead to secondary pollution. Therefore, effective control of NOx and SOx emissions is one of the key points in improving air quality in the vicinity. The NOx concentration emitted from this coal-fired boiler is 56 ± 2.17 ppm, with the NOx emission factor of 912 g/ton, indicating that the performance of SCR is relatively poor. On the other hand, the SOx concentration emitted is 1 ppm, with the SOx emission factor of 0.04 g/ton. Compared with other studies, this study shows that the operation of the existing FGD system in this coal-fired boiler is excellent.
Overall, the results obtained show that the APCDs adopted by this coal-fired boiler have good removal efficiencies for FPM, FPM2.5, SO2 and PAHs. However, the existing APCDs cannot efficiently remove CPM and NOx. It is important to properly operate and maintain the SCR to ensure NOx removal efficiency. Overall, large variations in the emission factors reported by different studies can be ascribed to many factors, such as boiler configuration, coal type and composition, and the performance of APCDs.
Conclusion
The characteristics of FPM, CPM, PAHs, and gaseous pollutants including SO2, NOx and HCl emitted from a coal-fired boiler located in Taiwan are investigated via intensive stack sampling and the results show that:
The NOx concentration emitted from the coal-fired boiler is 56 ± 2.17 ppm, indicating that the NOx removal efficiency (47.2%) achieved with the SCR adopted is not up to expectation. This may also be one of the reasons for the high concentration of CPM. It is recommended to check the activity of the catalyst and the NH3/NOx ratio regularly to ensure the good performance of SCR. On the other hand, SOx concentration emitted is < 1 ppm and the removal efficiency achieved is > 99%, indicating the operation of FGD is excellent.
The FPM concentration is reduced drastically from 5,341 ± 264 mg/Nm3 at APCD inlet to 0.9 ± 0.06 mg/Nm3 at the stack. The overall removal efficiency of FPM achieved with ESP+WFGD+WESP+MGGH is 99.98%. The FPM2.5 concentration emitted is < 0.09 ± 0.006 mg/Nm3, indicating that fine particles are well removed after passing through the APCDs. High concentration of CPM (37.4 ± 6.3 mg/Nm3) is measured at the stack, indicating that existing WESP has a limited removal efficiency for CPM and its operation needs to be optimized.
The PAHs concentration measured at the WESP inlet is 667 ng/Nm3. After passing through WESP+MGGH, the PAHs concentration is reduced to 547 ng/Nm3. The results show that APCDs adopted are not effective in removing PAHs (only 18%). The PAHs in flue gas are dominated by LMW of 2–3 rings, with gas-phase PAHs contributing the most. It is highly recommended to regulate the emissions of CPM and PAHs from coal-fired boilers in the future to reduce the impact of PM and PAHs emissions. In addition, whether WESP+MGGH can effectively reduce CPM emissions needs to be investigated in depth.
The emission factors calculated show that the emission factor of CPM is significantly higher than that of FPM and PM2.5, and the APCDs adopted by the coal-fired boiler investigated have good control efficiencies for filterable PM, and SO2.
Supplementary-Material.docx
Download MS Word (56.2 KB)Acknowledgment
The authors gratefully acknowledge the financial support provided by the Ministry of Science and Technology and Environmental Protection Administration, Executive Yuan, R.O.C. (MOST-107-EPA-F-006-004).
Disclosure statement
No potential conflict of interest was reported by the author(s).
Supplementary material
Supplemental data for this paper can be accessed on the publisher’s website
Additional information
Funding
Notes on contributors
Tang-Wei Chen
Tang-Wei Chen has a master degree in Graduate Institute of Environmental Engineering of National Central University in Taiwan. His research focus on polycyclic aromatic hydrocarbons (PAHs) and condensable particulate matter (CPM) emissions from combustion sources.
Jyh-Cherng Chen
Jyh-Cherng Chen is a distinguished professor in Department of Environmental Engineering and Science of Feng Chia University in Taiwan. He is engaged in the research of Incineration engineering, clean combustion, heat treatment technology and air pollution control technology.
Zhen-Shu Liu
Zhen-Shu Liu is a professor in Department of Safety Health and Environmental Engineering of Ming Chi University of Technology in Taiwan. She is engaged in the research of catalyst technology, air pollutant monitoring and control, resource regeneration and adsorption material synthesis.
Kai-Hsien Chi
Kai-Hsien Chi is an associate professor in Institute of Environmental and Occupational Health Sciences of National Yang Ming Chiao Tung University in Taiwan. He is engaged in the research of source characteristics, transport mechanism and environmental fate accumulation of dioxin pollutants in the environment.
Moo Been Chang
Moo Been Chang is a distinguished professor in Graduate Institute of Environmental Engineering of National Central University in Taiwan. He is engaged in the research of distribution characteristics of air toxic pollutants (such as PCDD/Fs, PAHs and PCN), PM2.5 and PAHs flow characteristics of coal combustion process and conversion and utilization of greenhouse gases (GHGs).
References
- Abdel-Shafy, H. I., and M. S. M. Mansour. 2016. A review on polycyclic aromatic hydrocarbons: Source, environmental impact, effect on human health and remediation. Egypt. J. Pet. 25:107–23. doi:https://doi.org/10.1016/j.ejpe.2015.03.011.
- Allen, J. O., N. M. Dookeran, K. A. Smith, A. F. Sarofim, K. Taghizadeh, and A. L. Lafleur. 1996. Measurement of polycyclic aromatic hydrocarbons associated with size-segregated atmospheric aerosols in Massachusetts. Environ. Sci. Technol. 30 (3):1023–31. doi:https://doi.org/10.1021/es950517o.
- Cancer, IAFRO. 1987. Overall evaluations of carcinogenicity: And updating of IARC monographs, volumes 1 to 42. IARC 7:1–440.
- Chen, X., C. Yuan, Q. Liu, T. Sheng, X. Zhang, D. Han, Z. Xu, X. Huang, H. Liao, and Y. Jiang. 2019. Emission characteristics of fine particulate matter from ultra-low emission power plants. Environ. Pollut. 255:113157. doi:https://doi.org/10.1016/j.envpol.2019.113157.
- Corio, L. A., and J. Sherwell. 2000. In-stack condensible particulate matter measurements and issues. J. Air Waste Manage. Assoc. 50:207–18. doi:https://doi.org/10.1080/10473289.2000.10464002.
- Dat, N. D., Y. J. Huang, Y. C. Hsu, and M. B. Chang. 2020. Emission characteristics of dl-PCNs, PCDD/ Fs, and dl-PCBs from secondary copper metallurgical plants: Control technology and policy. Chemosphere 253:126651. doi:https://doi.org/10.1016/j.chemosphere.2020.126651.
- Ehrlich, C., G. Noll, W. D. Kalkoff, G. Baumbach, and A. Dreiseidler. 2007. PM10, PM2.5 and PM10-emissions from industrial plants-results from measurement programmes in Germany. Atmos. Environ. 41 (29):6236–54. doi:https://doi.org/10.1016/j.atmosenv.2007.03.059.
- Farber, S. P., and G. D. Sloat. 2005. Reducing acid mist emissions from coal-fired power plants. COAL-GEN Power Generation Conference, San Antonio, Texas.
- Feng, Y., Y. Li, and L. Cui. 2018. Critical review of condensable particulate matter. Fuel 224:801–13. doi:https://doi.org/10.1016/j.fuel.2018.03.118.
- Forzatti, P. 2001. Present status and perspectives in de-NOx SCR catalysis. Appl. Catal. A-Gen. 222:221–36. doi:https://doi.org/10.1016/S0926-860X(01)00832-8.
- Goodarzi, F. 2006. The rates of emissions of fine particles from some Canadian coal-fired power plants. Fuel 85 (4):425–33. doi:https://doi.org/10.1016/j.fuel.2005.07.008.
- Hsu, W. T., M. C. Liu, P. C. Hung, S. H. Chang, and M. B. Chang. 2016. PAH emissions from coal combustion and waste incineration. J. Hazard. Mater. 318:32–40. doi:https://doi.org/10.1016/j.jhazmat.2016.06.038.
- Jia, C., and S. Batterman. 2010. A critical review of naphthalene sources and exposures relevant to indoor and outdoor air. Int. J. Environ. Res. Public Health 7:2903–39. doi:https://doi.org/10.3390/ijerph7072903.
- Khakharia, P., A. Huizinga, H. Trap, J. Monteiro, and E. Goetheer. 2019. Lab scale investigation on the formation of aerosol nuclei by a wet electrostatic precipitator in the presence of SO2 in a gas stream. Int. J. Greenh. Gas Control. 86:22–33. doi:https://doi.org/10.1016/j.ijggc.2019.01.024.
- Li, J., X. Li, M. Li, S. Lu, J. Yan, W. Xie, C. Liu, and Z. Qi. 2016. Influence of air pollution control devices on the polycyclic aromatic hydrocarbon distribution in flue gas from an ultralow-emission coal-fired power plant. Energy Fuels 30:9572–79. doi:https://doi.org/10.1021/acs.energyfuels.6b01381.
- Li, J., Z. Qi, M. Li, D. Wu, C. Zhou, S. Lu, J. Yan, and X. Li. 2017. Physical and chemical characteristics of condensable particulate matter from an ultralow-emission coal-fired power plant. Energy Fuels 31:1778–85. doi:https://doi.org/10.1021/acs.energyfuels.6b02919.
- Lu, P., J. Wu, and W. P. Pan. 2010. Particulate matter emissions from a coal-fired power plant. 2010 4th International Conference on Bioinformatics and Biomedical Engineering, Chengdu, China, 1–4.
- Maguhn, J., E. Karg, A. Kettrup, and R. Zimmermann. 2003. On-line analysis of the size distribution of fine and ultrafine aerosol particles in flue and stack gas of a municipal waste incineration plant: Effects of dynamic process control measures and emission reduction devices. Environ. Sci. Technol. 37:4761–70. doi:https://doi.org/10.1021/es020227p.
- Mertens, J., P. Khakharia, P. Rogiers, J. Blondeau, H. Lepaumier, E. Goetheer, B. Schallert, K. Schaber, and I. Moretti. 2017. Prevention of mist formation in amine based carbon capture: Field testing using a wet electrostatic precipitator (WESP) and a gas-gas heater (GGH). Energy Procedia 114:987–99. doi:https://doi.org/10.1016/j.egypro.2017.03.1244.
- Pei, B. 2015. Determination and emission of condensable particulate matter from coal-fired power plants. Huan Jing Ke Xue 36:1544–49.
- Ravindra, K., R. Sokhi, and R. Van Grieken. 2008. Atmospheric polycyclic aromatic hydrocarbons: Source attribution, emission factors and regulation. Atmos. Environ. 42:2895–921. doi:https://doi.org/10.1016/j.atmosenv.2007.12.010.
- Ren, Y., Q. Wu, M. Wen, G. Li, L. Xu, X. Ding, Z. Li, Y. Tang, Y. Wang, and Q. Li. 2020. Sulfur trioxide emissions from coal-fired power plants in China and implications on future control. Fuel 261:116438. doi:https://doi.org/10.1016/j.fuel.2019.116438.
- Ruan, R., H. Liu, H. Tan, F. Yang, Y. Li, Y. Duan, S. Zhang, and X. Lu. 2019a. Effects of APCDs on PM emission: A case study of a 660 MW coal-fired unit with ultralow pollutants emission. Appl. Therm. Eng. 155:418–27. doi:https://doi.org/10.1016/j.applthermaleng.2019.03.136.
- Ruan, R., X. Xu, H. Tan, S. Zhang, X. Lu, P. Zhang, R. Han, and X. Xiong. 2019b. Emission characteristics of particulate matter from two ultralow-emission coal-fired industrial boilers in Xi’an, China. Energy Fuels 33 (3):1944–54. doi:https://doi.org/10.1021/acs.energyfuels.8b04069.
- Shen, G., W. Wang, Y. Yang, C. Zhu, Y. Min, M. Xue, J. Ding, W. Li, B. Wang, H. Shen, et al. 2010. Emission factors and particulate matter size distribution of polycyclic aromatic hydrocarbons from residential coal combustions in rural Northern China. Atmos. Environ. 44:5237–43. doi:https://doi.org/10.1016/j.atmosenv.2010.08.042.
- U.S. EPA (United States Environmental Protection Agency). 2016. Method 202-Condensable particulate matter. https://www.epa.gov/emc/method-202-condensable-particulate-matter.
- U.S. EPA (United States Environmental Protection Agency). 2019. AP-42 (Fifth Edition): Compilation of air emissions factors, Volume I, Chapter 1: External combustion sources. https://www3.epa.gov/ttn/chief/ap42/ch01/final/c01s01.pdf.
- Wang, G., J. Deng, Y. Zhang, Y. Li, Z. Ma, J. Hao, and J. Jiang. 2020. Evaluating airborne condensable particulate matter measurement methods in typical stationary sources in China. Environ. Sci. Technol. 54:1363–71. doi:https://doi.org/10.1021/acs.est.9b05282.
- Wang, R., B. Yousaf, R. Sun, H. Zhang, J. Zhang, and G. Liu. 2016. Emission characterization and δ13C values of parent PAHs and nitro-PAHs in size-segregated particulate matters from coal-fired power plants. J. Hazard. Mater. 318:487–96. doi:https://doi.org/10.1016/j.jhazmat.2016.07.030.
- Wang, R., G. Liu, R. Sun, B. Yousaf, J. Wang, R. Liu, and H. Zhang. 2018. Emission characteristics for gaseous- and size-segregated particulate PAHs in coal combustion flue gas from circulating fluidized bed (CFB) boiler. Environ. Pollut. 238:581–89. doi:https://doi.org/10.1016/j.envpol.2018.03.051.
- Wu, B., H. Tian, Y. Hao, S. Liu, X. Liu, W. Liu, X. Bai, W. Liang, S. Lin, Y. Wu, et al. 2018. Effects of wet flue gas desulfurization and wet electrostatic precipitators on emission characteristics of particulate matter and its ionic compositions from four 300 MW level ultralow coal-fired power plants. Environ. Sci. Technol. 52:14015–26. doi:https://doi.org/10.1021/acs.est.8b03656.
- Wu, B., X. Bai, W. Liu, S. Lin, S. Liu, L. Luo, Z. Guo, S. Zhao, Y. Lv, and C. Zhu. 2020. Non-negligible stack emissions of noncriteria air pollutants from coal-fired power plants in China: Condensable particulate matter and sulfur trioxide. Environ. Sci. Technol. 54:6540–50. doi:https://doi.org/10.1021/acs.est.0c00297.
- Xu, Y., X. Liu, J. Cui, D. Chen, M. Xu, S. Pan, K. Zhang, and X. Gao. 2016. Field measurements on the emission and removal of PM2.5 from coal-fired power stations: 4. PM removal performance of wet electrostatic precipitators. Energy Fuels 30:7465–73. doi:https://doi.org/10.1021/acs.energyfuels.6b00426.
- Yang, H. H., K. T. Lee, Y. S. Hsieh, S. W. Luo, and M. S. Li. 2014. Filterable and condensable fine particulate emissions from stationary sources. Aerosol Air Qual. Res. 14:2010–16. doi:https://doi.org/10.4209/aaqr.2014.08.0178.
- Yang, H. H., K. T. Lee, Y. S. Hsieh, S. W. Luo, and R. J. Huang. 2015. Emission characteristics and chemical compositions of both filterable and condensable fine particulate from steel plants. Aerosol Air Qual. Res. 15:1672–80. doi:https://doi.org/10.4209/aaqr.2015.06.0398.
- Yang, H. H., S. M. Arafath, Y. F. Wang, J. Y. Wu, K. T. Lee, and Y. S. Hsieh. 2018. Comparison of coal-and oil-fired boilers through the investigation of filterable and condensable PM2.5 sample analysis. Energy Fuels 32 (3):2993–3002. doi:https://doi.org/10.1021/acs.energyfuels.7b03541.
- Yang, Z., C. Zheng, X. Zhang, C. Li, Y. Wang, W. Weng, and X. Gao. 2017. Sulfuric acid aerosol formation and collection by Corona discharge in a wet electrostatic precipitator. Energy Fuels 31:8400–06. doi:https://doi.org/10.1021/acs.energyfuels.7b01090.
- Zhang, L., L. Yang, Q. Zhou, X. Zhang, W. Xing, Y. Wei, M. Hu, L. Zhao, A. Toriba, K. Hayakawa, et al. 2020. Size distribution of particulate polycyclic aromatic hydrocarbons in fresh combustion smoke and ambient air: A review. J. Environ. Sci. 88:370–84. doi:https://doi.org/10.1016/j.jes.2019.09.007.
- Zheng, C., Y. Hong, S. Liu, Z. Yang, Q. Chang, Y. Zhang, and X. Gao. 2018. Removal and emission characteristics of condensable particulate matter in an ultralow emission power plant. Energy Fuels 32 (10):10586–94. doi:https://doi.org/10.1021/acs.energyfuels.8b02464.