ABSTRACT
We report results from a blind comparison of six analytical laboratories ISO/IEC 17025 accredited for the implementation of the analytical element of EN 1911, which involves the quantification of chloride in deionized water collected from HCl emitting industrial processes regulated under the EU’s Industrial Emissions Directive (IED). Both “synthetic” (sodium chloride dissolved in deionized water) and “real” (extracted and collected from a stack simulator facility) samples were prepared across ranges which were equivalent to concentrations in the stack of 0–10 mg·m−3 and 0–60 mg·m−3, respectively. Laboratory measurements of the real samples showed significantly poorer performance than the synthetic, implying that the use of synthetic samples in national proficiency testing schemes may be leading to an overly optimistic view of the uncertainties that can routinely be achieved in measurements of real industrial processes. In addition, at the applicable emission limits (10 mg·m−3 and 3 mg·m−3) and measurement ranges (0–15 mg·m−3 and 0–4.5 mg·m−3) under the IED and more recent BAT Conclusions legislation it was found that of the real samples 22 out of 102 (21.6%) and 28 out of 51 (54.9%), respectively, of the measurements would not comply with the overall uncertainty that at least one national regulator considers as necessary for EN 1911 to be an “effective tool” for the calibration of automated measuring systems (AMSs – process plant operator analyzers providing continuous monitoring of emissions). Hence, it is proposed that at the next revision of EN 1911 the standard should be revised to give the same degree of consideration to the analytical element of the method as the sampling element. Key analytical laboratory uncertainty sources should be identified, numerical uncertainty requirements should be placed on key analytical uncertainty sources, and there should be an overall uncertainty requirement for the analytical element.
Implications: The deviations observed between laboratories ISO/IEC 17025 accredited for chloride analysis bring into question the ability of the current version of EN 1911 (the CEN Standard Reference Method for monitoring HCl industrial emissions) to meet the uncertainty requirements associated with emission limits under both the EU’s Industrial Emissions Directive and the increasingly stringent industrial sub-sectors BAT Conclusions legislation. It is proposed that at the next revision of EN 1911 that uncertainty guidance and requirements for the analytical element are added if this measurement method is to continue to be mandated across Europe for ensuring emissions data meet legislative quality requirements.
Introduction
Hydrochloric acid (HCl) is acutely toxic to all forms of life, contributes to the formation of photochemical smog and increases the erosion of buildings and other infrastructure. In recent years, emissions to air from industrial processes have been regulated via the EU’s Industrial Emissions Directive [IED – (EC, Citation2010)], which came into force in 2013. With respect to HCl, the IED – in common with the legislation it superseded (EC, Citation2000) – stipulates an emission limit of 10 mg·m−3, i.e. the emission limit has remained unchanged for some time. However, recently, new legislation has been passed augmenting the requirements of the IED for some industrial sub-sectors setting increasingly stringent limits for this important pollutant.
Best Available Techniques (BAT) Reference (BREF) documents – which detail best available technology for key components of industrial facilities (e.g. for waste incinerators: waste delivery; bunker; incineration/steam generation; flue gas clean-up; emissions from the stack) – are drawn up by the EIPPCB (European Integrated Pollution Prevention and Control Bureau) with input from member states, industry and non-governmental organizations concerned with environmental protection. The Associated Emission Levels (AELs) for pollutants within such documents are included in Commission Implementing Decisions (known as BAT Conclusions (BATCs)) in-line with Article 13(5) of the IED. i.e. AELs are a legislative requirement and local competent authorities (for readability henceforth referred to as “national regulators”) are required to enforce such limits within a 4-yr period of grace from publication. With respect to HCl, key AELs and associated BATCs include: 2–6 mg·m−3 Waste Incineration (EC, Citation2019); 3–12 mg·m−3 Large Combustion Plants (EC, Citation2017); ≤1.5 mg·m−3 Non-ferrous Metals Industries (EC, Citation2016). Hence, much of this new legislation augmenting the IED is already in place and either in force or shortly going to be in force.
As with other pollutants, HCl emissions are ultimately reported into the European Pollutant Release and Transfer Register [E-PRTR (EC, Citation2006)], which is one of the key mechanisms whereby the EU meets its international commitments under the Aarhus (UNECE, Citation1998) and Kiev (UNECE, Citation2003) protocols to provide publicly available data on its progress in reducing polluting emissions. However, HCl is not part of the Convention on Long-range Transboundary Air Pollution [CLRTAP (UNECE, Citation1979)], unlike other acidic emission species, such as SO2 and NH3. It has always been considered that due to its high-water solubility HCl deposition occurs only localized to the pollution source. However, Evans et al. have reported (Evans et al. Citation2011) data of UK lake and stream samples taken from 1986 to 2007 wherein the early years there was a much higher than expected concentration of HCl than could be accounted for from sea spray alone (the atmospheric chlorine cycle being dominated by sea salt), and noting a decrease in concentration of 30–40% in this time period made a link to the UK decrease in coal burning. This finding of HCl deposition much farther from the pollution source than would be expected seems to challenge the established thinking, clearly more work and discussion in the peer review literature on this topic would be welcome.
The EC supports the monitoring and enforcement of industrial emissions legislation via Mandates formerly requesting the Comité Européen de Normalization (CEN) to produce Standard Reference Methods (SRMs) for key pollutants. An SRM being defined by EN 15259 (CEN, Citation2007b) as a “reference method prescribed by European or national legislation,” i.e. SRM documentary standards are not voluntary but mandatory. An SRM has two roles: to provide periodic measurements to demonstrate emissions are within the applicable emission limit (“compliance monitoring”); to annually provide a calibration/calibration check of process plant operators’ Automated Measuring System (AMS) via parallel measurements. The former role is self-explanatory, with respect to the latter the IED regulates processes of >50 MW thermal input and requires many processes to install AMSs (e.g. gas filter correlation, Fourier transform spectroscopy). The AMS provides continuous year-round monitoring of plant emissions to air ensuring that any breaches are captured. It is a requirement that a calibration/calibration check of the AMS is carried out annually in accordance with EN 14181 (CEN, Citation2014), which gives a standardized method for parallel measurements between the AMS and SRM. So, whether considering continuous AMS measurements of emissions or compliance measurements, the accuracy of all emissions data for a given species is in principle dependent on the applicable SRM.
The SRM for HCl is described in documentary standard EN 1911 “Stationary source emissions – Determination of mass concentration of gaseous chlorides expressed as HCl – Standard reference method” (CEN, Citation2010). EN 1911 describes what is colloquially referred to as a “wet chemistry” method. The method involves inserting a heated probe into the stack and extracting a sample of the emission through a heated filter (to remove dust) and bubbling it through a series of glass impingers filled with deionized water to dissolve the HCl as chloride. The default run time is typically 30 min, after which the deionized water solutions are decanted and despatched to an analytical laboratory for quantification generally by ion chromatography, although mercuric-thiocyanate spectrophotometry or silver titration are also permitted. Combined with knowledge of the stack flow rate, the emissions are then reported in units of mg·m−3 (at a standard temperature and pressure of 273 K and 101.3 kPa).
The 2010 version of EN 1911 is a revision of an earlier version with a few technical changes (CEN documentary standards are reviewed on a 5-yr basis where nations vote to re-confirm, revise or withdraw). The validation of EN 1911 was performed more than 20 yr ago and carried out at various waste incinerator processes. Until recently this has not necessarily been an issue since, as described above, the legislative emission limit has remained unchanged throughout most of this time, although it could be argued that industrial process has however changed and, hence, it is not certain that this would not affect the measurement (e.g. due to different interference effects). In any case, with lower emission limits due to BAT Conclusions it is timely to take stock and discuss the emissions community’s capability to monitor decreasing HCl emissions.
Complimenting a previous study we reported regarding the SO2 SRM (Coleman et al. Citation2019a), here we report results from a study involving splitting (to eliminate stack gas extraction and collection uncertainties) chloride samples collected from a stack simulator facility and synthetic samples prepared in a laboratory. When a sampling team collects HCl from a stack, it is at liberty to despatch the collected samples to any ISO/IEC 17025 accredited laboratory for analysis. Consequently, the uncertainty that the measurements are subject to is driven by the variance across the community of analytical laboratories. Hence, the purpose of this study was not to assess the proficiency of individual laboratories but rather to understand the variance (and therefore uncertainty) across the community of analytical laboratories and therefore if EN 1911 is providing sufficient control of the analytical method. This variance is discussed in the context of the capability of EN 1911 to provide both compliance monitoring and the calibration of AMS, under both the IED and the new BAT Conclusions legislation covering some industrial sub-sectors.
Experimental
“Synthetic samples” to simulate HCl emissions captured as chloride in solution were prepared by dissolving NaCl in deionized water (the solvent stipulated in EN 1911). Five solutions were prepared of 4.76, 9.67, 14.63, 19.21, 24.41 µg·mL−1, and each stock solution was split 24-fold (NPL’s capability to prepare homogeneous solutions being evidenced via its ISO/IEC 17025:2017 (ISO, Citation2017) accreditation for such preparations). Four samples from each of the five concentration test levels were despatched to six analytical laboratories ISO/IEC 17025 accredited for the quantification of chloride in accordance with EN 1911 (i.e. each analytical laboratory received 20 samples with 120 measurements being made). None of the laboratories were told of the nature of the study, i.e. the testing was “blind.” Also, in order to maintain the pretense that the samples had all been collected during routine monitoring of a real stack, all the samples were despatched from the NPL Emissions Team with the usual chains of custody. Laboratories were asked to report quantitative values with associated uncertainties. Reported results were converted from µg·mL−1 to mg·m−3 using a typical impinger solution volume of 0.2 L and a stack gas sampled volume of 0.5 m3 (nb. unless stated otherwise m3 is expressed at 273 K and 101.3 kPa). Hence, for the reference concentrations, this gave reference stack concentration values of 1.9, 3.9, 5.9, 7.7 and 9.8 mg·m−3.
“Real samples” were prepared by generating representative stack gas matrices in the NPL Stack Simulator Facility (Coleman et al., Citation2015; Coleman et al. Citation2019b) and sampling the facility following EN 1911 in full, i.e. inserting a heated probe into the stack, extracting gas and passing it through a series of impingers filled with deionized water before despatch to analytical laboratories for chloride quantification. A total of 52 individual test matrices were generated varying the concentration of each species across the following ranges: HCl 0–60 mg·m−3; SO2 0–290 mg·m−3; CO 0–100 mg·m−3; NO 0–300 mg·m−3; H2O 0–14%vol. Other species were included at fixed concentrations: 15 mg·m−3 NH3; 30 mg·m−3 NO2; VOC mixture (9 mg·m−3 CH4/8 mg·m−3 C2H6/8.5 mg·m−3 C3H8); 10%vol CO2 and 10%vol O2. The simulator was operated at 180°C and a recirculating gas velocity of 12 m·s−1. Stack simulator gas was extracted through a 5” BSP (British Standard Port) using a titanium lined Apex heated probe connected to Greenburg-Smith borosilicate glass impingers via a PTFE insulated heated line (both the probe and PTFE line were heated to 180°C). The temperature and total volume of stack gas sampled were measured with an Apex XC-572 Metric Meter Console, and the gas was extracted using an Apex XE-0523 Method 5 pump set nominally to 16 L·min−1. Isokinetic sampling was not necessary as the simulator had previously been demonstrated as homogeneous in accordance with both EN 15267 and ISO 13528 (ISO, Citation2005) as part of NPL’s ISO 17043 (ISO, Citation2010) accreditation, which evidences competence for generating representative stack emission matrices. Also, NPL is accredited under ISO/IEC 17025 for the implementation of EN 1911, and all samplings were carried out by NPL staff certified to MCERTS Level 2 under the Environment Agency for England Personal Competency Standard (EA, Citation2018).
For each 30 min sample taken from the stack simulator, 3 borosilicate impingers were connected in series with deionized water in the first two and the third being empty for the purpose of capturing any solution spill over. At the end of each sampling, the impingers were disconnected and any spillover in the 3rd impinger was poured into the 2nd, along with rinses of the connectors between the 1st and 2nd impingers, the 2nd and 3rd impingers and of the 3rd impinger itself. The resultant solutions in the 1st and 2nd impingers were decanted and despatched separately for quantification so that it would be possible to apply the absorbance efficiency test in accordance with EN 1911, which requires that ≤5% of the total quantity of chloride measured is found in the 2nd impinger after the aforementioned rinsing procedure (this providing confidence that all the HCl sampled from the stack has been successfully captured between the two impingers). It was found (data not shown) that the absorbance efficiency test requirement was met for all samplings of the stack simulator. The real samples were despatched to 3 of the 6 laboratories (arbitrarily chosen) involved in quantifying the synthetic samples described above. In common with the above, testing of the analytical laboratories was blind and the samples were treated as if acquired from a real stack with all the usual chains of custody. Laboratories were requested to report quantitative values with associated uncertainties.
Results and discussion
For the synthetically prepared samples it is seen that out of the 120 measurements 113 (94%) deviate from the associated reference value by less than the laboratory stated k = 2 uncertainty limit (). Given that a coverage factor of k = 2 should be commensurate with a 95% probability that the “true value” resides within the stated limits, the data appear consistent with the stated uncertainties. Of the remaining seven measurements where the reported value deviates from the reference value in excess of the stated uncertainty, four reside at the 1.9 mg·m−3 test level, one at the 5.9 mg·m−3 level, two at the 9.8 mg·m−3 level: and furthermore, these are distributed across three of the six laboratories. However, it is noteworthy that three of the four results at the 1.9 mg·m−3 test level are attributable to the same laboratory, this indicating that this laboratory may have difficulty in routinely meeting its stated uncertainty at low concentrations.
Figure 1. Relative deviation of analytical laboratory measurements (■) from reference values of synthetic chloride samples prepared at 5 different emission reference concentrations, 1.9, 3.9, 5.9, 7.7 and 9.8 mg·m−3 (▬) with associated uncertainties reported by laboratories at a confidence level of 95% (k = 2). Laboratory measurements presented as a repeating sequence of laboratory a, b, c, d, e.
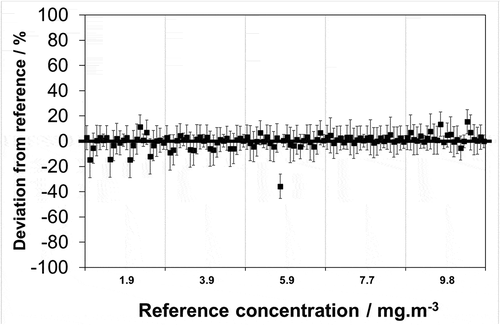
It is pertinent to also consider the uncertainty to which the synthetic samples are prepared and the stability/contamination. With respect to the former, the reference concentrations are known to an uncertainty of 2% at k = 2, this being evidenced by NPL’s ISO/IEC 17025 accreditation for the preparation of such solutions (where solution homogeneity is included in the uncertainty). Combining this with the stated laboratory uncertainties (via root sum of squares) the same level of agreement is found (results not shown), i.e. the same seven measurements deviate from the associated reference value in excess of the re-calculated uncertainties. This is unsurprising given that the reference value uncertainty is significantly lower than the uncertainties of any of the laboratories and so has only a minor influence.
With respect to sample stability/contamination, then issues due to time, sample bottle contamination and transportation/storage conditions are all considered. For temporal stability and sample bottle contamination, these issues should be systemic (as all bottles are acquired in the same batch from the same supplier) and so it is reasonable to expect excessive deviation from at least the other laboratories at the lowest test level, and possibly also at other test levels across all laboratories. However, neither of these possibilities are observed. In terms of transport/storage conditions then such samples are not light or temperature sensitive and if samples were to leak, then it is likely this would be noticed, and in any case would not alter the concentration of the solution still residing within the bottle.
In terms of the real samples, it is not possible to make exactly the same type of comparisons as discussed above as the reference values are not known or more specifically not known to a good enough uncertainty. Instead, a comparison is made to the mean of the measurements from each test. How good an estimate the mean is of the “true value” is in this instance not an issue. This is because what is being considered is the deviation between laboratories, and so if the deviation from the mean (or indeed any other value used as an estimate of the “true value”) is significant or even in excess of the maximum permissible uncertainty, this raises questions over the uncertainty that can routinely be achieved under EN 1911. In many European nations (including the UK), the regulator does not require the same organization that has sampled the stack to carry out the quantification; hence, analytical laboratories are sub-contracted for this purpose. Clearly, it would be undesirable for there to be a significant step change in an industrial site’s emission because the sampling organization changed (as is not uncommon) the sub-contracted analytical laboratory. However, even in nations where the same organization that carries out the sampling is required to carry out the quantification this is not avoided since industrial site operators often change sampling organization just as they would suppliers of any commercial service.
Across the real sample data set, it is seen that 76 out of 156 (48.7%) measurements deviate from the mean in excess of the laboratory stated k = 2 uncertainty limit (). However, the real samples span a greater concentration range than the synthetic. The latter, by design, span a 0–10 mg·m−3 range since as described in the Introduction, the emission limit value for HCl has remained at 10 mg·m−3 in EU legislation over roughly the last two decades. Extracting data across the same concentration range (0–10 mg·m−3) from the real samples, it is found that 33 out of 78 (42.3%) of the measurements deviate from the mean in excess of the laboratory uncertainty limit (). Hence, given that for the synthetic samples, the analogous result was 7 out of 120 (5.8%) it appears that analytical laboratory agreement deteriorates when challenged with solutions that in addition to chloride contain other species dissolved from the stack emissions (e.g., NH3, SO2, NO2).
Figure 2. Relative deviation from the mean of analytical laboratory measurements (■) of real chloride samples over a 0–60 mg·m−3 range extracted and collected from a stack simulator facility. Associated uncertainties reported by laboratories at a confidence level of 95% (k = 2).
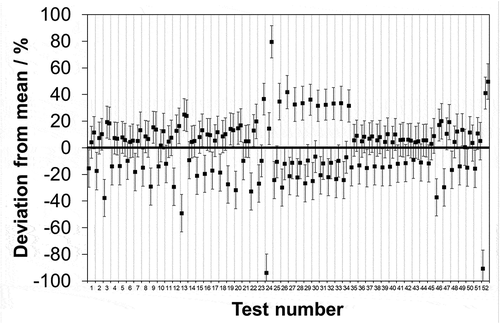
The observation above is potentially critical when the context of national proficiency testing schemes is considered. ISO/IEC 17025 stipulates in Clause 7.7.2 that a laboratory shall (i.e. it is mandatory) participate in either a proficiency testing scheme or an interlaboratory comparison other than a proficiency testing scheme where available and appropriate. Furthermore, many national regulators require participation in such schemes as to adequately police industrial sites the quality of the emissions data must be understood, i.e. to justify an intervention, financial penalty or even to pursue legal action through the courts a national regulator must have confidence in the emissions data and know that it will stand up to scrutiny. In Europe, there are stack simulator facilities with established proficiency testing schemes in Germany (Cordes, Stoffels, and Wildanger, Citation2015), France, UK (Coleman et al., Citation2013, Citation2019b), and Belgium where participation is required by the national regulator. Such facilities are the ideal infrastructure to test participant proficiency for the implementation of the SRMs (such as EN 1911) as both sampling and quantification are tested. Nations without such infrastructure generally rely on despatching samples much like has been done for the synthetic samples reported herein and as is well understood this leaves the proficiency for stack sampling un-tested. However, what is implied from the data reported here ( compared to ) is that if the despatched samples are similar to the synthetic samples here and do not contain other species that will be present in the stack (as is the case with the real samples) then not only is the proficiency of sampling excluded but the returned data may provide an overly optimistic view of quantification proficiency. Hence, it is important that despatched samples for such schemes are as representative of the real-world as possible if national regulators are to understand the quality of the analytical element of EN 1911, and quite possibly other wet chemistry-based SRMs as well. Clearly, this is also important to national accreditation bodies who use proficiency testing data to determine if accreditation can be awarded to an organization who are claiming a certain measurement capability (uncertainty).
As described in the Introduction, the role of an SRM is two-fold: to provide periodic measurements to demonstrate emissions are within the applicable emission limit (“compliance monitoring”); to annually provide a calibration/calibration check of process plant operator Automated Measuring System (AMS) via parallel measurements. Annex VI, Part 6 of the IED requires that HCl is measured by the process plant operator AMS to an uncertainty of not more than 40% of the ELV at k = 2 (95% confidence). However, the IED in Part 3, Clause 8 requires that the AMS is calibrated in accordance with CEN standards. CEN has provided EN 14181 for this purpose, where a calibration is based on a regression between 15 parallel measurements with the SRM (often of 30 min each) spread across 3 days on the stack where the AMS is installed or, for a calibration check, 5 parallel measurements. Importantly, EN 14181 requires that the AMS is type approved in accordance with EN 15267–3 (CEN, Citation2007a) where instrument manufacturers submit new products to recognized independent instrument testing laboratories for testing under this standard. Further, EN 15267–3 requires that the AMS passes a series of laboratory (e.g., cross-interference from other species, linearity) and field (a minimum of 3 months at a real process plant) tests (e.g., drift, reproducibility) meeting an uncertainty of at least 25% below that specified. So, in the case of HCl the specified requirement is 40% of the ELV, which for the purpose of passing type approval under EN 15267–3 becomes 30% of the ELV. The associated HCl SRM is aligned with this requirement as Clause 8.3 of EN 1911 stipulates that the SRM shall be carried out to an uncertainty of 30% of the ELV. It would certainly be metrologically flawed in the regulatory framework if it were permissible for the ongoing quality assurance (calibration/calibration check) of an installed AMS to be based on an SRM of poorer uncertainty than had been required of the AMS in type approval.
As the required uncertainty for both compliance measurements and AMS calibration/calibration check is 30% of the ELV, it is applicable to discuss the synthetic and real sample measurements in this context. An ELV of 10 mg·m−3 equates to an absolute uncertainty requirement of ± 3 mg·m−3: it is clear that all of the synthetic and real sample deviations from the respective reference and mean values are significantly within this limit. However, the maximum permissible uncertainty covers the entire method described in EN 1911 not only the analysis part carried out by the analytical laboratory, i.e. there are sampling uncertainty sources that should also be considered. Much as for SO2 (EN 14791 (CEN, Citation2017)) EN 1911 provides an identical list of sampling uncertainty sources in Table 1: volume of solution in impingers; volume of extracted stack gas; temperature of extracted stack gas; pressure of extracted stack gas; sampling apparatus leak rate; field blank. Whilst clearly the sampling uncertainty will vary between stack testing organizations, it is reasonable to use a representative figure for the purposes of discussion. Previously for SO2, we determined a representative sampling uncertainty of 7.2%. Given the approach to sampling in the two standards is identical, and the chemical nature of the two species has no bearing on such physical uncertainty sources the same value shall be used here. Subtracting (root of the quadrature subtraction) 7.2% of the reference and mean values for the synthetic and real samples from the maximum permissible uncertainty (30% of ELV = ± 3 mg·m−3) shows the required uncertainty portion remaining that the analytical laboratory must not exceed if the overall measurement is to be compliant. Carrying this out, it is seen that none of the 120 synthetic measurements (results not shown) and 1 out of 78 (1.3%) real measurements () deviate in excess of this limit. However, with respect to EN 14181 and AMS calibration/calibration check, some nations have more stringent requirements for the SRM. For example, the national regulator for England has stipulated that it shall be assured that the SRM uncertainty is “… well below the uncertainties specified in the IED (ideally, no more than half the uncertainties specified in the IED). If these conditions are not fulfilled, then EN 14181 will not be an effective tool for calibration of the [AMS]...” It could be argued that the required part of the above is met as the maximum permissible uncertainty in EN 1911 is 30% of ELV and this is “well below” the IED requirement of 40% of ELV. The second part of the above regulation is a recommendation (the operative word being “ideally”) so non-adherence does not constitute noncompliance: however, given that SRM measurements should be performed to “no more than half the uncertainties” otherwise “EN 14181 will not be an effective tool for calibration” the strength of the recommendation is clear. If a sampling uncertainty of 7.2% is subtracted (root of the quadrature subtraction) from the reference and mean values it is now seen that 1 of the 120 (0.8%) and 6 of the 78 (7.7%) synthetic (results not shown) and real measurements () deviate in excess of the uncertainty requirement (n.b. in absolute units whilst the uncertainty requirement is fixed (30% or 20% of the ELV) the same is not true of the sampling uncertainty as it is 7.2% of the mean value which changes across the tests, hence, the (▬) and (…) limits on are not straight lines). Whilst the rate of deviation in excess of the uncertainty requirement is almost an order of magnitude greater for real samples compared to synthetic, it is still close enough to 5% that this might be considered acceptable with respect to AMS calibration. However, whilst true, what needs to be considered is the full legislatively required measurement range, which is not 0–10 mg·m−3; hence, the discussion will return to this important point shortly.
Figure 4. Data reproduced from in units of concentration covering a 0–10 mg·m−3 range. Maximum permissible uncertainty at 95% confidence (–––) as required by EN 1911 for compliance monitoring of waste incinerators in accordance with an IED emission limit value of 10 mg·m−3; uncertainty remaining for analysis after subtracting typical uncertainties attributable to the extraction and collection of HCl from the stack from 75% of the IED requirement (▬), and 50% of the IED requirement (…).
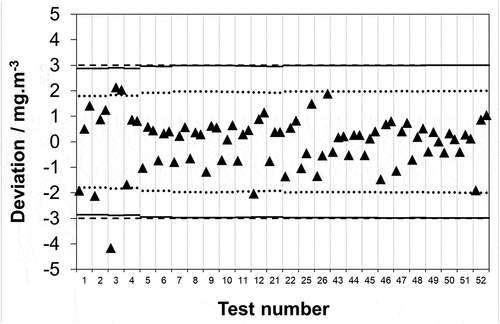
In terms of comparing the results here for HCl to those reported for SO2 in (Coleman et al., Citation2019a) there are several observations that can be made. Across the respective full concentration ranges for the two sets of real samples, it is found that the uncertainty limits of at least two laboratories do not intersect in 33 out of 52 tests (63%) for HCl and 14 out of 46 tests (30%) for SO2. Given that the five laboratories that comprise the SO2 study are all included in the study reported herein, this would seem to imply that in general uncertainties for sulfate measurement have been better estimated than for chloride. However, given that the laboratory claimed uncertainties are stated at a confidence level of 95% (k = 2) the instances of uncertainty limits not intersecting should be around 5%, so it seems as though for both species the uncertainties are underestimated.
It is also possible to compare the SO2 data to HCl in terms of AMS calibration. Just as the required measurement uncertainty and performance characteristic tests in type approval are all linked to the respective ELV, so is the required measurement range. In the case of waste incinerators, this is 1.5-fold the ELV: hence, 1.5 × 10 = 15 mg·m−3 for HCl and 1.5 × 50 = 75 mg·m−3 for SO2. Subtracting (root of the quadrature subtraction) a 7.2% of associated mean value sampling uncertainty from 75% of the required uncertainty as above shows that 10 out of 102 (9.8%) HCl () and 12 out of 96 (12.5%) SO2 test results deviate in excess of the remaining uncertainty. If, as above, this calculation is repeated using 50% of the required uncertainty (in-line with the recommendation of the national regulator for England) it is now seen that 22 out of 102 (21.6%) HCl () and 37 out of 96 (38.5%) SO2 test results deviate in excess of the remaining uncertainty. Whilst the performance of HCl is better than for SO2, it is again clear that the frequency of excess deviation for both species is significantly above the 5% that would be expected for a confidence level of 95%.
Figure 5. Data reproduced from in units of concentration covering a 0–15 mg·m−3 range. Maximum permissible uncertainty at 95% confidence (–––) as required by EN 1911 for compliance monitoring of waste incinerators in accordance with an IED emission limit value of 10 mg·m−3; uncertainty remaining for analysis after subtracting typical uncertainties attributable to the extraction and collection of HCl from the stack from 75% of the IED requirement (▬), and 50% of the IED requirement (…).
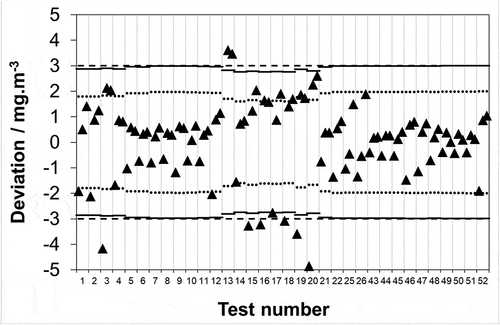
It is worth noting that the majority (particularly for HCl) of deviations in excess of the uncertainty requirement fall at concentration values above the ELV. This is partly because the ELV is fixed, whereas the mean of the measurements for each test is not. Hence, in terms of absolute units, the former provides a fixed value (3 mg·m−3 at 75% of the IED requirement or 2 mg·m−3 at 50%), whereas the latter is a variable. Consequently, at high test concentrations the sampling uncertainty gives a high absolute value that is subtracted (root of the quadrature subtraction) from the absolute required uncertainty resulting in a smaller portion remaining for the analytical laboratory than at low test concentrations. Of course, this all assumes that the absolute sampling uncertainty scales perfectly with concentration. This is less likely at low concentrations and hence in reality there may be more deviations at this end of the concentration scale in excess of the uncertainty requirement than are seen. However, as it is frequently assumed within the community that the relative uncertainty remains fixed when descending to lower concentrations the data are analyzed on this basis.
Assuming the majority of industrial processes are run within respective ELVs, then is it an issue that many excess deviations are being observed at concentrations above the ELV? There are two answers to this: Firstly, emissions that exceed the ELV need to meet all data quality requirements so national regulators have a defendable basis from which to carry out interventions or in extreme cases action through the courts; secondly, even a well-run process can in some cases justifiably emit above the ELV and legislative allowance has sensibly been made for this. The maximum permissible uncertainty in EN 1911 and the required uncertainty in the IED are both expressed as a percentage of the daily (24 h) ELV (it is the daily ELV that has been used in all the discussions above). EN 1911 is based on a 0.5 h measurement period for collecting chloride from the stack, and AMS measurements can similarly be thought of as a continuous series of 0.5 h measurements (under EN 14181 a minimum of 15 parallel measurements of 0.5 h each are required for calibration of the AMS by the SRM). But, what importantly the IED recognizes is that it would be wrong to assume that the emissions from every process are always steady state. For example, it can easily be envisaged how solid fuel being delivered into a furnace by a screw feeder will give non-laminar fuel delivery resulting in peaks and troughs in the emissions out of the stack. Consequently, for HCl in Annex 6, Part 8 of the IED it stipulates that the average daily emissions shall not exceed the daily ELV at any time, but that across the year either none of the half-hourly averages shall exceed a half-hourly ELV of 60 mg·m−3 or 97% of the half-hourly emission averages shall not exceed a limit of 10 mg·m−3. This controls emissions both on a daily basis whilst also allowing spikes over smaller time increments, albeit with a limit on the magnitude. Hence, it can be seen that for national regulators, process plant operators and indeed all stakeholders that it is just as important (if not more so) that uncertainty requirements are met at the top of the measurement range as at the bottom.
As mentioned in the Introduction, BAT Conclusions have brought in AEL ranges resulting in increasingly stringent HCl emission limits across a range of industrial sub-sectors: 2–6 mg·m−3 Waste Incineration (EC, Citation2019); 3–12 mg·m−3 Large Combustion Plants (EC, Citation2017); ≤1.5 mg·m−3 Non-ferrous Metals Industries (EC, Citation2016). The concentration test level increments used lend themselves to extracting data across a 0–4.5 mg·m−3 range, which as discussed above is the required range if the emission limit is 3 mg·m−3. It was seen above that at a range of 15 mg·m−3 subtracting (root of the quadrature subtraction) a 7.2% of associated mean value sampling uncertainty from 75% and then 50% of the IED uncertainty requirement resulted in 10 out of 102 (9.8%) and 22 out of 102 (21.6%) measurements deviating in excess of the required uncertainty (). At an ELV of 3 mg·m−3 (4.5 mg·m−3 range) these change to 10 out of 51 (19.6%) and 28 out of 51 (54.9%) (). This is a marked deterioration and raises concerns over the ability of EN 1911 to enforce BAT Conclusions-based emission limits given the current measurement infrastructure. However, in one sense, this should perhaps not be a surprise as generally policymakers legislate beyond current measurement capability. If alternatively, policymakers allowed themselves to be limited by current measurement capability emission limits would never be lowered and new measurement methods would not subsequently be innovated to meet new legislation, i.e. generally, innovation follows the legislation. However, in another sense, there should be some concern here as there is not just work needed to meet the new legislation but more concerningly there appears some doubts whether the requirements of the IED (which has been in force for some time) can be routinely met.
Figure 6. Data reproduced from in units of concentration covering a 0–4.5 mg·m−3 range. Maximum permissible uncertainty at 95% confidence (–––) as required by EN 1911 for compliance monitoring of waste incinerators in accordance with a BAT Conclusions emission limit value of 3 mg·m−3; uncertainty remaining for analysis after subtracting typical uncertainties attributable to the extraction and collection of HCl from the stack from 75% of the IED requirement (▬), and 50% of the IED requirement (…).
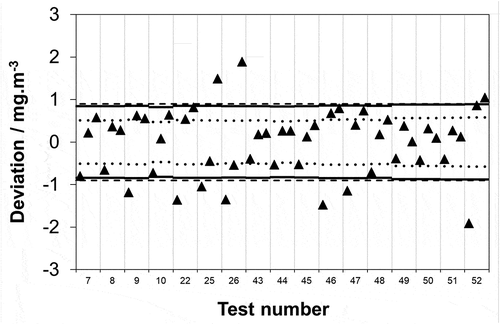
If in all of the above discussions, there was only a single laboratory showing significant deviations and all others were well within the requirements then this would imply that there was an issue with the given laboratory. As this is not the case, the implication is that the level of performance that is being observed is the best possible when following EN 1911, i.e. the standard is not providing enough control of the measurement method. Examining EN 1911 it is seen that there is much more quality control associated with the sampling element of the document than there is with the analytical element. In EN 1911, Table 1 provides performance requirements for sampling, namely: determination of the volume of the absorption solution (≤1.0%); uncertainty of sample volume (≤2.0%); uncertainty of temperature (≤2.5 K); uncertainty of absolute pressure (≤1.0%); absorption efficiency (>95%); leak in the sampling line (≤2.0%). The first four of these characteristics being included in the uncertainty budget for the overall method. Table 2 then provides the performance characteristic for the analytical laboratory, namely: standard deviation of analytical repeatability of chloride (≤2.5% of measured value). This is a comparatively superficial treatment of the analytical element of the method. Moreover, uncertainty is a combination of precision (i.e. repeatability) and bias (accuracy) and so the standard places no accuracy requirements specific to the analytical step. If a laboratory were to significantly under-read but do this consistently (i.e., to a repeatability ≤2.5%) than it would comply with Table 2 just as well as a laboratory with negligible bias.
EN 1911 could be improved if specific uncertainty requirements were included to address the analytical uncertainty. A good example of this can be found in the measurement method CEN/TC 264 “Air Quality” have recently published for HF (CEN, Citation2020) where it is listed that the following analytical uncertainty sources shall all be considered when analyzing collected samples by ion chromatography: performance characteristics of the analysis equipment; preparation of calibration standards; purity of stock standard solution and ratio of dilutions; linearity of calibration curve depending on the amplitude of the measuring range; measurement of the volume of aliquot of the solution injected for the analysis (ratio of the total absorption solution volume and the volume of the aliquot taken for injection); dilution ratio, if a dilution of the absorption solution is necessary before analysis; interferences; drift of retention time. It does not go as far as setting individual uncertainty requirements for each of these but importantly does expand Table 2 wherein addition to an analysis repeatability requirement of ≤2.5% there is an analytical uncertainty requirement of ≤37% of value.
At the next revision of EN 1911 (TC 264 standards are reviewed on a periodicity of 5 yr) there should be equal attention paid to the analytical element of the standard as the sampling element. The standard should be revised to include a list of performance characteristics potentially contributing the greatest uncertainty to each of the three permitted analytical techniques that the user must be required to consider and estimate. Ideally, there should be a required uncertainty value associated with each of the key uncertainty sources for the user to meet. Critically, there should then be in addition to a repeatability requirement of ≤2.5% an analytical uncertainty requirement. Setting specific uncertainty requirements for performance characteristics and an overall uncertainty for the analytical step will bring the analytical element of the standard into line and to a similar QA/QC level as the sampling element. This improved QA/QC of the analytical element should then lead to improved comparability of data between analytical laboratories and an overall improvement in the uncertainty of the entire method. Also, providing a list of the performance characteristics leading to the greatest uncertainty contribution to each of the three analytical techniques will help analytical laboratories in terms of knowing where to target improvements in their procedures to obtain the greatest gain. If such improvements cannot be made, then the community may need to move away from such a wet chemistry approach toward portable optical instruments (e.g., Fourier transform infrared, cavity ringdown spectroscopy) for the future enforcement of EU legislation for limiting the release of polluting substances to air.
Conclusion
Across a 0–10 mg·m−3 HCl concentration range, it was found that for the real samples 33 out of 78 (42.3%) of the measurements deviated from the mean in excess of the uncertainty quoted by the respective laboratory, whereas the analogous result for the synthetic samples was 7 out of 120 (5.8%). This contrast showed that national proficiency testing schemes based on synthetic samples – which also often underpin ISO/IEC 17025 accreditations – may be providing an overly optimistic view of the level of uncertainty that can be routinely achieved following the analytical element of EN 1911. It was also found, after allowing for typical extraction and collection uncertainties, that under the Industrial Emissions Directive (10 mg·m−3 emission limit, 0–15 mg·m−3 measurement range) and BAT Conclusions (3 mg·m−3 emission limit, 0–4.5 mg·m−3 measurement range) 22 out of 102 (21.6%) and 28 out of 51 (54.9%), respectively, of the measurements would not comply with the overall uncertainty that at least one national regulator considers as necessary for EN 1911 to be an “effective tool” for the calibration of AMSs. These observations raise questions over not only the emission community’s ability to calibrate AMSs to enforce new legislation covering some industrial sub-sectors (BAT Conclusions) but also over enforcement of the Industrial Emissions Directive that has been in place since 2013. In addition, these observations are not the result of a single laboratory where there has been some issue with the implementation of EN 1911, they are systemic: hence, it is EN 1911 itself that must be examined. Currently, EN 1911 does not consider the analytical element of the method as thoroughly as the sampling element: likely uncertainty sources are not identified, individual uncertainty requirements are not placed on key analytical uncertainty sources, and there is no overall uncertainty requirement for the analytical element. If EN 1911 is to be relied upon for calibration/calibration check of AMSs in accordance with EN 14181 to enforce EU legislation, these issues should be addressed in the next revision of this important CEN standard.
Acknowledgment
The authors gratefully acknowledge funding from the European Metrology Programme for Innovation and Research (which is jointly funded by the EMPIR participating countries within EURAMET and the European Union) and the UK’s Department for Business, Energy and Industrial Strategy National Measurement System under the Energy and Environment Program.
Disclosure statement
No potential conflict of interest was reported by the author(s).
Additional information
Funding
Notes on contributors
Marc D. Coleman
Marc D. Coleman, Matthew Ellison, Rod A. Robinson and Thomas O.M. Smith are all metrologists working in the Emissions and Atmospheric Metrology Group at the UK's National Metrology Institute (the “National Physical Laboratory”).
Matthew Ellison
Marc D. Coleman, Matthew Ellison, Rod A. Robinson and Thomas O.M. Smith are all metrologists working in the Emissions and Atmospheric Metrology Group at the UK's National Metrology Institute (the “National Physical Laboratory”).
Rod Robinson
Marc D. Coleman, Matthew Ellison, Rod A. Robinson and Thomas O.M. Smith are all metrologists working in the Emissions and Atmospheric Metrology Group at the UK's National Metrology Institute (the “National Physical Laboratory”).
References
- Coleman, M. D., M. Ellison, R. A. Robinson, T. D. Gardiner, and T. O. M. Smith. 2019a. Uncertainty requirements of the European Union’s Industrial Emissions Directive for monitoring sulfur dioxide emissions: Implications from a blind comparison of sulfate measurements by accredited laboratories. JA&WMA 69 (9):1070–78 doi:https://doi.org/10.1080/10962247.2019.1604449.
- Coleman, M. D., R. A. Robinson, M. B. Williams, M. J. Clack, and D. M. Butterfield. 2013. State of UK emissions monitoring of stacks and flues: An evaluation of proficiency testing data for SO2, NO and particulates. Accred. Qual. Assur. 18 (6):517–24. doi:https://doi.org/10.1007/s00769-013-1011-x.
- Coleman, M. D., S. Render, C. Dimopoulos, A. Lilley, R. A. Robinson, T. O. M. Smith, R. Camm, and R. Standring. 2015. Testing equivalency of an alternative method based on portable FTIR to the European standard reference methods for monitoring emissions to air of CO, NOx, SO2, HCl, and H2O. JA&WMA 65 (8):1011–19 doi:https://doi.org/10.1080/10962247.2015.1058868.
- Coleman, M. D., T. O. M. Smith, R. A. Robinson, B. Stoffels, and D. Wildanger. 2019b. Combining UK and German emissions monitoring proficiency testing data based on stack simulator facilities to determine whether increasingly stringent EU emission limits are enforceable. Accred. Qual. Assur. 24 (2):127–36. doi:https://doi.org/10.1007/s00769-018-1354-4.
- Comité Européen de Normalisation. 2007a. Air quality—Certification of automated measuring systems—Part 3: Performance criteria and test procedures for automated measuring systems for monitoring emissions from stationary sources. EN 15267–3. http://www.cen.eu.
- Comité Européen de Normalisation. 2007b. Air quality—Measurement of stationary source emissions—Requirements for measurement sections and sites and for the measurement objective, plan and report. EN 15259. http://www.cen.eu/.
- Comité Européen de Normalisation. 2010. Stationary source emissions—Determination of mass concentration of gaseous chlorides expressed as HCl—Standard reference method. EN 1911. http://www.cen.eu/.
- Comité Européen de Normalisation. 2014. Stationary source emissions—Quality assurance of automated measuring systems. EN 14181. http://www.cen.eu/.
- Comité Européen de Normalisation. 2017. Stationary source emissions—Determination of mass concentration of sulphur dioxide—Standard reference method. EN 14791. http://www.cen.eu/.
- Comité Européen de Normalisation. 2020. Stationary source emissions—Determination of mass concentration of fluorinated compounds expressed as HF—Standard reference method. CEN/TS 17340. http://www.cen.eu/.
- Cordes, J., B. Stoffels, and D. Wildanger. 2015. The question of homogeneity inside a chimney: Application of ISO 13528 to stack emission proficiency tests. Accred. Qual. Assur. 20 (4):287–95. doi:https://doi.org/10.1007/s00769-015-1139-y.
- Environment Agency for England. 2018. Personnel competency standard for manual stack-emission monitoring. Version 11. http://www.gov.uk/.
- European Commission. 2000. Directive 2000/76/EC of the European Parliament and of the Council of 4 December 2000 on the incineration of waste. Off. J. Eur. Commun. L332, 91–111.
- European Commission. 2006. Regulation (EC) No 166/2006 of the European Parliament and of the Council of 18th January 2006 Concerning the establishment of a European pollutant release and transfer register and amending Council Directives 91/689/EEC and 96/61/EC. Off. J. Eur. Union L33, 1–17.
- European Commission. 2010. Directive 2010/75/EU of the European Parliament and of the Council of 24 November 2010 on industrial emissions (integrated pollution prevention and control). Off. J. Eur. Commun. L334, 17–119.
- European Commission. 2016. COMMISSION IMPLE-MENTING DECISION (EU) 2016/1032 of 13 June 2016 establishing best available techniques (BAT) conclusions, under Directive 2010/75/EU of the European Parliament and of the Council, for the non-ferrous metals industries. Off. J. Eur. Union L174, 32–106.
- European Commission. 2017. COMMISSION IMPLE-MENTING DECISION (EU) 2017/1442 of 31 July 2017 establishing best available techniques (BAT) conclusions, under Directive 2010/75/EU of the European Parliament and of the Council, for large combustion plants. Off. J. Eur. Union L212, 1–82.
- European Commission. 2019. COMMISSION IMPLEMENTING DECISION (EU) 2019/2010 of 12 November 2019 establishing the best available techniques (BAT) conclusions, under Directive 2010/75/EU of the European Parliament and of the Council, for waste incineration. Off. J. Eur. Union L312, 55–92.
- Evans, C. D., D. T. Monteith, D. F. Fowler, J. N. Cape, and S. Brayshaw. 2011. Hydrochloric acid: An overlooked driver of environmental change. Environ. Sci. Technol. 45 (5):1887–94. doi:https://doi.org/10.1021/es103574u.
- International Organization for Standardization. 2005. Statistical methods for use in proficiency testing by interlaboratory comparisons. ISO 13528.
- International Organization for Standardization. 2010. Conformity assessment—General requirements for proficiency testing (ISO/CASCO 17043:2010). ISO 17043.
- International Organization for Standardization. 2017. General requirements for the competence of testing and calibration laboratories. ISO/IEC 17025.
- United Nations Economic Commission for Europe. 1979. Convention on long-range transboundary air pollution. Geneva, Switzerland: UNECE.
- United Nations Economic Commission for Europe. 1998. Convention on access to information, public participation in decision-making and access to justice in environmental matters, Aarhus, Denmark, June 25.
- United Nations Economic Commission for Europe. 2003. Protocol on pollutant release and transfer registers, Kiev, Ukraine, May 21.