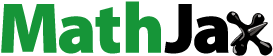
ABSTRACT
This study investigated the adsorption characteristics of the carbonaceous adsorbents for organic compounds in gas to gain technical knowledge useful for the appropriate operation and management of the incineration plants and industrial heat treatment facilities. The experiments in the study were performed using a dynamic method, employing a small column packed with test adsorbent, into which flowed the model gas, primarily over a temperature range of 150°C to 190°C. Three activated carbon materials and an activated coke were used, with specific surface areas ranging from 250 to 1,100 m2/g-adsorbent. Organic components in the gas were produced and supplied at a concentration of tens of mg/m3, and gaseous mercury was supplied at a concentration of around 100 μg/m3. The experimental results showed the following: 1) The adsorption capacity of every carbonaceous material for organic vapor decreased with increasing temperature, with roughly a three-fold difference in the capacity, between 150°C and 190°C in the dry gas. The equilibrium adsorption amount of benzene could be estimated based on the specific surface area of the adsorbent. 2) Gas moisture reduced the equilibrium adsorption amount of adsorbates by about half. 3) The estimation of the treatment performance for actual adsorption processing suggested the possibility of decreased removal efficiency at higher temperatures above 175°C. Overall, the precise process design should be made based on future practical studies.
Implications:
Adsorptive characteristics of activated carbon materials were examined for benzene and chlorobenzene in gas within the temperature range of 150°C to 190°C.
The adsorption amount of benzene at high temperature and low concentration range below 20 ppm was in the range of 103 to 104 mg/kg-adsorbent.
There was a clear difference between the adsorption parameters of activated carbon and coke for the benzene adsorption, depending on their specific surface area values and other factors.
Introduction
The emission of hazardous pollutants as a result of thermal treatment in the industrial processes and from the thermal waste thermal treatment has been an essential matter of concern in engineering for a long time. A variety of organic and inorganic compounds, including heavy metals, exist in the exhaust gases released from such processes. The concentrations of the materials range widely, from percent to ppm and even further to trace levels, such as ppt. There are a variety of pollutants that are regulated for the emission in the environment to avoid air pollution, and also, many pollutants are not regulated even if they have harmful effects after environmental emission. Sulfur dioxide (SO2) and nitrogen dioxide (NO2) have historically long been representative of gaseous inorganic pollutants in the air. Dioxins are also well known as toxic organic pollutants, and those present in flue gas have already been regulated in many countries, with a typical standard of 0.1 ng-TEQ/m3N for the incineration of municipal solid waste (MSW) as developed in Japan (Ministry of the Environment −1 Citation2021).
Volatile organic compounds (VOCs) such as benzene and toluene can be emitted from the above thermal processes in the concentration from ppb to ppm levels as well as emissions from vehicles. These are the substances that are inevitably generated from the thermal process of various substances including not only fossil fuels and natural products. Although the VOC emissions from the facilities using these chemicals for industrial productions could be regulated, the emissions from thermal processes that do not necessarily use VOCs are not usually regulated. However, a possible environmental emission caused by unintentional formation from such processes is not negligible, and there have been serious concerns about the environmental impact and the impact on human health (Cheng et al. Citation2018; Khan and Ghoshal Citation2000; Tomatis et al. Citation2016; Zhang et al. Citation2017) of VOCs. Unintentional pollutants other than dioxins can be generated from the thermal waste treatment. The production and emission of brominated dioxins and chlorinated naphthalenes, which are chemically close to dioxins and are classified as semi-VOCs rather than VOCs, is well known (Hu et al. Citation2013; Kawamoto Citation2009). The co-incineration of waste in the cement kilns is a viable waste management option. However, this can also lead to higher levels of hazardous emissions if the operation is not appropriately controlled. For example, a pollutant category of the total organic carbon, including benzene and VOCs in gas, was reported on average between 20 and 35 mg/m3 based on information from Germany and Switzerland (Waltisberg and Weber Citation2020). Indeed, the concentrations of VOCs, including benzene, ranged in ppm in flue gas of incineration of MSW as evident from several measurements in Japan (from the personal data of the author).
The emission of VOCs from many industrial sources that use these chemicals has gradually decreased after the introduction of the regulation of pollutant release and transfer register (PRTR) of Japan in 1999 (Ministry of the Environment −2 Citation2021). However, any focus has not been considered on the emission of pollutants from the thermal processes.
To control the emission of above-mentioned hazardous pollutants, effective and reliable air pollution control technology is required. This technology should be selected based on the property of the pollutant, the concentration range of the pollutants, and existing environment in the matrix. Among the potentially applied technical measures, so far, the most effective and reliable technology for exhaust gas treatment is adsorption technology using adsorbents such as activated carbon (Chan et al. Citation2009; Inoue, Yasuda, and Kawamoto Citation2009; Zhang et al. Citation2017). Activated carbon adsorbent has wide applicability for a variety of relatively low-concentration components in gas and water. A typical case of application of this technique is the treatment of exhaust gas from the waste incineration facilities. Activated powdered carbon is in practical use for the removal of dioxins, by adding it to the exhaust duct before the bag filtration.
However, there are several points for consideration before the application of adsorption technology to the exhaust gas treatment. Most notably, the flue gas is usually large in quantity at a high temperature between 150°C and 200°C typically in the case of incineration, and water vapor and a variety of compounds coexist in the gas. This temperature range is due to the acidic nature of the incineration exhaust gas on the low-temperature side, and the limitation of the fabric filter material and also the inhibition of dioxin formation on the high-temperature side. Although many studies have been conducted (Lasker et al. Citation2019; Ma et al. Citation2020; Nikam and Mandal Citation2020; Yu, Luo, and Grevillot Citation2002) with regard to adsorption of VOCs on activated carbons, most of the research has been conducted from the viewpoint of solvent treatment, targeting the temperature from normal temperature to about 100°C. The adsorption behavior of activated carbon at high temperature is not clear. Also, it is not clear whether the adsorption technique would be applicative to any substance under these optimal conditions.
The present study focused on the VOCs as target substances and investigated the adsorption characteristics of the carbonaceous adsorbents for benzene and another compound as model adsorbates of VOCs in gas. This was done to gain the technical knowledge useful for the appropriate operation and management of the incineration plants and industrial heat treatment facilities.
Experimental method
Materials
The carbonaceous materials used in the experiments, namely three activated carbons and an activated coke have been shown in , along with their relevant properties. The reason for selecting the adsorbent used in this study is that the waste incineration facility and the sintering process of iron ore are assumed as the actual place where the high-temperature exhaust gas treatment to which the adsorption treatment is applied is performed. Also, it is rational to use activated carbon, which is often used for the main purpose of removing dioxins in recent incineration facilities, and activated coke, which is used in the exhaust gas of the sintering process, as the adsorbent often used in those facilities. All the samples were coal-based and in commercial use for the flue gas treatment as mentioned above. Activated carbons A and B (The former received a sample from Cataler Corporation, Kakegawa, Japan, and the latter received a sample from company Cargon Carbon Japan, at that time, KURARAY CO., LTD, Tokyo Japan now.) were designed for dioxin removal, and the carbon C (Made by Cargon Carbon Japan, at that time, KURARAY CO., LTD, Tokyo now.) that has relatively small specific surface area was developed for the treatment of mercury in the flue gas. Activated coke that was sampled by JM Activated Coke, Inc, Kitakyushu, Japan, was used as an adsorption material and decomposition catalyst for SOx and NOx removal as it is relatively inexpensive in comparison to the activated carbon. The micropore distribution characteristics were investigated by the nitrogen gas adsorption test and by BET (Brunauer-Emmett-Teller) analysis. The relationship between the pore diameter and pore volume has been shown in Figure S1 for the representative adsorbent, activated carbon A. It was shown that micropores of 3 nm or less were dominant in the cumulative pore volume.
Table 1. Adsorbent samples used and their properties
In this study, benzene and chlorobenzene were used as the organic adsorbates, as they are very common components emitted from the thermal treatment processes. Chlorobenzene has been used as a surrogate in studies of dioxins in the past (Yan et al. Citation2010). In this study, special-grade reagent products were used for these two reagents made by FUJIFILM Wako Pure Chemical Corporation, Osaka Japan. The physical chemical properties of the substances have been shown in . The vapor pressures of benzene and chlorobenzene were found to be 1.26 × 104 Pa and 1.57 × 103 Pa at 25°C, respectively.
Table 2. Adsorbate compounds used and their properties
Experimental equipment and procedure
illustrates the adsorption test system, including the equipment for generating the organic and Hg vapors. The generation of vapor or low concentration gas was conducted using a calibration gas generation system device – Permeater PD-1B- provided by Gastec Corporation (Ayase, Japan). This system utilizes diffusion and/or permeation tubes to generate the gaseous matter for the calibration of the measuring instrument. The basic theory and mechanism of gas generation are as follows:
The liquid reagents of benzene and chlorobenzene were fed into the diffusion tube, which was kept at a constant temperature in the range of 30–50°C. The diffusion tube was selected by the design consideration on the volatilization rates of the target compounds and the outlet gas concentrations required. The structure of the diffusion tube is shown in Figure S2. The diffusive rate of the liquid compound can be calculated by the next equation.
Here,
Dr: Diffusion rate (µg/min)
M: Molecular weight of a substance
P: Atmospheric pressure at the place of a tube (mmHg)
T: Temperature at the same place (K)
R: Gas constant (62,358 mmHgcm3/(Kmol))
D: Vapor diffusion coefficient at T and P (cm2/s)
A: Cross-sectional area of diffusion tube (cm2)
L: Length of diffusion tube (cm)
Ps: Saturated vapor pressure of a substance at T (mmHg)
The value of Ps is obtained from Antoine equation: A – B/(C + t), and A, B, C are Antoine constants at temperature t (°C). Then, the produced gas concentration (ppm) can be obtained by the next equation,
Here,
Cgas: Substance concentration targeted in produced gas (ppm)
F: Flow rate of dilution gas in the Permeater (mL/min)
K: Constant calculated from next eq.: K =
The generated gas in this device was allowed to flow out of the device and then entered a bottle that acted as a buffer to control the gas flow rate. The gas containing a target substance for the adsorption test line was suctioned by a vacuum pump and a needle valve from the buffer tank to keep the constant flow rate at around 500 mL/min. In case of benzene, the value of Dr was calculated to be 41.1 µg/min based on the use of a diffusion tube, which had the dimensions of 0.053cm2 for A and 5 cm for L in Equationeq. (1)(1)
(1) at 35°C. If the flow rate of the dilution gas is 1 L/min, the concentration of the benzene in the gas prepared by the Permeater device is 41.1 mg/min and this mass concentration corresponds to 13.3 ppm (V/V) at the above temperature. It has been shown that low concentrations of standard gas can also be prepared. In addition, various types of diffusion tubes were used to adjust the respective gas concentrations of benzene and chlorobenzene.
The test method using the adsorption test device has been further described. The outlet gas was intermittently sampled over a designated time period to measure the dynamic breakthrough characteristics under various conditions. Thereafter, a breakthrough curve was plotted. The outlet gas was collected in n-hexane solvent, and the gas concentrations of benzene and chlorobenzene were determined based on the solution concentration and gas sample volume. The test flow is shown in .
The amount of adsorbent used in the experiments was packed in the glass tube in the range of 0.1 to 0.2 g according to the experimental condition, and the packed length was 4 mm with an internal diameter of 10 mm. The space velocity (SV) in a packed device was determined by the next equation:
Where, Q is the gas flow rate (L · h−1) and V is the packed bed volume (L). Based on the above data of the packed tube, the SV values in the experiments ranged from 7,000 to 62,000 h−1. The setting of this SV value is based on the fact that the SV of activated carbon adsorption treatment in actual exhaust gas treatment is usually set to a few thousand to tens of thousands h−1. In addition, even in the treatment of high-temperature incinerator exhaust gas, the SV value is usually set to several thousand h−1. In this experiment, the value was made even larger because it was an accelerated test.
The experiments were conducted within the temperature range of 100°C to 200°C. It was done because the flue gas cleaning in the process of the incineration of municipal solid waste is usually performed in the range of 150°C to 200°C and at around 100°C in the area of industrial thermal processes. Since those exhaust gases contain much of water vapor, hence the conditions for the coexistence of water vapor were also set as the experimental conditions. The value was set to about 20% (V/V), which was a typical value, but it was measured at the time of each test.
Analytical method of adsorption parameters and measurement method of chemicals
The breakthrough curve was obtained by evaluating the correlation between the gas supply time and C/C0 ratio (outlet gas concentration C to inlet gas concentration C0) by intermittently measuring the outlet gas concentration. The outlet gas sampling was carried out by estimating the transition and progress of the outlet concentration and adsorption breakthrough, for example, by performing for about 5 minutes every 1 to several hours. The equilibrium adsorption amount was calculated by integrating the breakthrough curve according to the method as reported previously (Inoue and Kawamoto Citation2005). To evaluate the progress of breakthrough, the time when C/C0 ratio reaches 0.05 to 0.1 was used as an index. Because the concentration change data was not acquired in the real time in this study, the breakthrough characteristic was evaluated using the time t50 that meant C/C0 ratio reached 0.5.
The hexane solution that collected organic adsorbate after the adsorption test was conditioned with the volumetric flask and subjected to instrumental analysis. The concentration of these substances in hexane solvent was in the range of 0.1 to several mg/L. The analysis for benzene and chlorobenzene was performed using a low-resolution gas chromatography: GC6890N (Agilent Technologies Japan, Ltd.) and mass spectrometry: JMS K9/B (JEOL Ltd., Japan) system. This system used a capillary column of DB-624UI (Agilent Technologies Japan, Ltd.), and the analysis was carried out using the SIM mode.
Before taking data on adsorption breakthrough curve, we checked if the gas containing the substance was supplied at a constant concentration. A result for benzene was shown in Figure S3 in Supplement Materials. The result showed that the substance was supplied at a substantially constant concentration in the experiment. The supply concentration was measured in every adsorption test.
Results and discussion
Adsorption breakthrough characteristics of benzene and chlorobenzene and influence of gas temperature
The time change of adsorbate concentration in the outlet gas from the adsorption test column was measured in this study at various temperatures and under other conditions. Based on the results obtained, adsorption isothermal lines were determined. To obtain the data, experiments were conducted initially for benzene. The adsorption breakthrough curves, with activated carbon B, at temperatures of 150°C, 175°C, and 190°C in dry gas have been shown in . The data indicated that the time to adsorption saturation decreased with increasing temperature – lower the temperature, longer the time to reach breakthrough, with a roughly three-fold difference in the complete breakthrough time, between the lowest and highest temperature values. The value of t50 was about 4 times different and ranged from 12 min for 190°C to 47 min for 150°C. Thus, the effect of temperature was large, and it was probably due to the endothermic nature of the adsorption process/reaction.
Figure 2. Breakthrough curves of benzene with activated carbon B under three temperature conditions (supply concentration: 37 mg/m3; SV: 28,000 h−1).
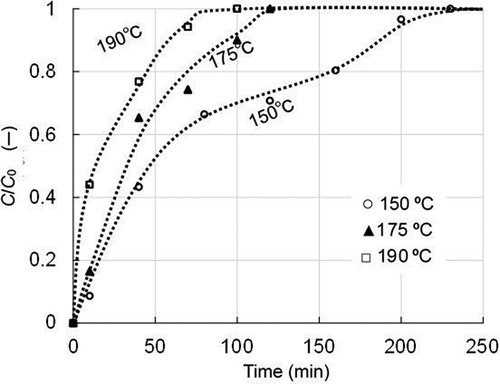
Here, another result obtained under the same conditions is shown in Figure S4 in Supplement Materials to demonstrate the reproducibility of the experiment. This is the data for benzene in dry gas condition at 150°C. The result is almost the same as the data at the same temperature in , and the reproducibility of the experiment was considered to be satisfactory.
Similar curves to were also observed in the cases of the other three adsorbents. However, the breakthrough time with the activated coke was less than with the activated carbons because of its smaller specific surface area under the same temperature conditions. Yangyang et al. (Citation2016) have tested the adsorption breakthrough properties on dioxin surrogates including benzene by a method similar to the present study. The test conditions they used were as follows: 0.1 g of activated carbon sample was placed on the column bed, the temperature was 120°C, 100 ppm benzene-containing gas was supplied at a flow rate of 300 mL/min, and therefore the SV was 72,000 h−1. The results show that the complete breakthrough was reached in 40 min. Although the concentration of benzene supplied was higher than the setting of this study and the SV was slightly higher, and the temperature was slightly lower than the setting range of this study, the results of this study and them showed almost reasonable agreement depending on the difference in each condition.
The results for the chlorobenzene with the same adsorbent have been shown in . The breakthrough times at all three temperatures for this compound were relatively long compared to benzene. The value of t50 was about 3 times higher than that for benzene and ranged from 50 min for 190°C to 175 min for 150°C. The result must reflect the difference in the vapor pressure properties between the two compounds as shown in .
Figure 3. Breakthrough curves of chlorobenzene with activated carbon B under three temperature conditions (supply concentration: 55 mg/m3; SV: 28,000 h−1).
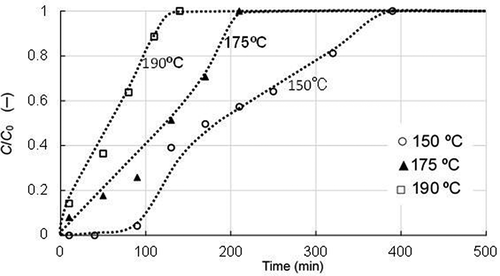
Influence of moisture content and adsorbate supply concentration
The effect of moisture as a process factor in the gas was also examined because it behaved competitively with adsorbate compounds. shows the change of breakthrough curves of benzene by different moisture concentrations at 150°C with activated carbon A. Actual moisture concentration was determined to be 26% (V/V) at these runs. From this result, at 150°C, the value of time t50 was 30 min for the dry conditions and 13 min for the moisture coexistence conditions. At 170°C, the values were 25 min and 5.3 min, respectively. The rate of decrease in t50 values due to moisture coexistence was 0.43 at 150°C and 0.21 at 170°C, and the rate of decrease was greater at lower temperatures. It can be said that low-temperature conditions are more susceptible to moisture. The property of polarity of the molecule may affect the adsorption behavior of the respective compounds, and since the actual flue gas contains moisture in many cases, care must therefore be taken in the application of such adsorption techniques.
Figure 4. Change of breakthrough curves of benzene by different moisture concentration at 150°C (Adsorbent: Activated carbon A, Supply concentration: 24 mg/m3, SV: 60,000 h−1, Moisture: 26%(V/V).
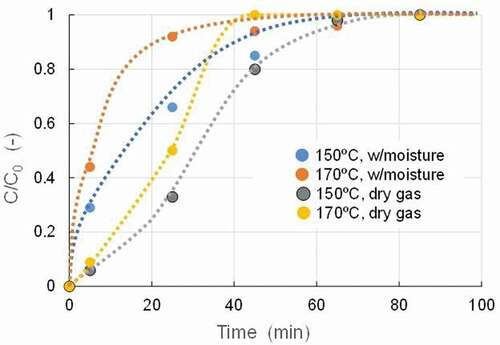
The supplied concentration also affected the adsorption breakthrough time as shown in . The results for two concentrations of benzene, 14 and 37 mg/m3, gave t50 values of 47 and 20 minutes, respectively, which showed the higher the supply concentration, the faster the breakthrough. This is a reasonable data reflecting the progressive increase in adsorption.
Figure 5. Breakthrough curves for two conditions with different supply concentrations of benzene (Dry gas, Adsorbent: Activated carbon A, Temperature: 150°C).

Analysis of adsorption equilibrium of benzene
Based on the breakthrough curve data, the equilibrium adsorption amount of adsorbate was calculated by integrating the area between the curve and the maximum horizon at the top of the figure. On confirming the consistency with both the Freundlich’s formula (Inoue and Kawamoto Citation2005) and the Langmuir formula for the data, the both results are shown in . From two figures and data observation, the results of the two analyses were generally comparable, but there was a discrepancy in the results of the Langmuir equation analysis for the activated coke. Therefore, it was considered appropriate to understand the experimental data as following the Freundlich’s formula:
Figure 6. Adsorption isotherms of four adsorbents for benzene in gas at 150°C – Results of data analysis using Freundlich (a) and Langmuir (b) equations.
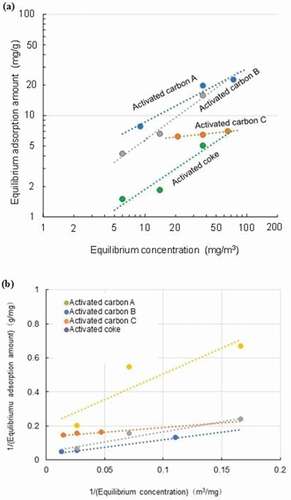
where q is the equilibrium adsorption amount at an equilibrium concentration of 1 mg/m3 of adsorbate, and a value of 1/n represents linearity. Hung and Lin (Citation2007) reported that the Dubinin-Radushkevich-Langmuir model could be applied to predict the equilibrium capacity at relatively low pressures of VOCs such as benzene and toluene. According to their study, the Freundlich equation did not predict the capacity precisely at the low concentration levels. However, the temperature range used for examination in their study was room temperature which was much different from the present study. It is generally pointed out that the Freundlich equation applies well in a limited range of conditions, and this was observed in the results from our previous studies as well (Inoue and Kawamoto Citation2005, Citation2008).
The adsorption mechanism in physical adsorption relies on relatively weak forces, such as van der Waals forces and forces caused by hydrogen bonds. In addition, the adsorption capacity also depends on the size of the specific surface area, which determines the amount of adsorption sites present. It is believed that this is also true for adsorption at high temperatures, which is the target of this study. Benzene is a typical nonpolar compound because it contains only C – C and C – H bonds with a flat shape, and each C–H bond exists in exactly the opposite direction. If the substance to be adsorbed is hydrophobic, neutral, and nonpolar, it should be typical for the adsorption properties to follow the physical adsorption mechanism of activated carbon. This suggests that the benzene molecules are physically adsorbed by these forces.
Activated carbons A and B have large specific surface areas over 1,000 m2/g, while that of the activated coke is only 256 m2/g (), and the results clearly reflected this difference. There is no significant difference in the slope 1/n value of the Equationequation (4)(4)
(4) between the three adsorbents (0.73 for activated A, 0.53 for activated B and 0.79 for activated coke). However, activated carbon C exhibited a unique result, and the non-linearity expressed by 1/n value (0.11) was more pronounced than that of the three materials. The equilibrium adsorption amount did not increase even in the region where the adsorbate concentration was high. It is not clear whether this is related to the fact that this material is manufactured specifically for mercury adsorption; however, it is suggested that this material has different properties from the usual mechanistic properties where the specific surface area and pore volume values have the strongest effect on the adsorption capacity.
The results in at 150°C showed that when the equilibrium concentration in the gas was around 10 mg/m3, the equilibrium adsorption amount was about several mg to 10 mg/g-adsorbent even for the activated carbon having a large specific surface area. This value was considerably smaller than the value at room temperature, for example, 437 mg/g-adsorbent (Baytar et al. Citation2020), which may clearly indicate that the temperature is an important influential factor on adsorption.
A study using low concentrations of 1,2,3,4-tetrachlorobenzene in gas showed that the adsorption ability for the neutral organic compounds onto carbonaceous adsorbent basically depends on the amount of the specific surface area and volume derived from micropores of the adsorbent (Inou, and Kawamoto Citation2005). Indeed, a clear relationship exists between the specific surface area and equilibrium adsorption amount in each condition as shown in , and at every temperature, the larger the area, the larger the amount, though the values are otherwise rather disparate. Based on this figure, the equation for estimating the equilibrium adsorption amount from the specific surface area value of the adsorbent at any given temperature was derived to be Equationequation (5)(5)
(5) ;
Figure 7. Relationship between the specific surface area and equilibrium adsorption amount for each tested adsorbent.

where, Tf is the flue gas temperature (°C), and Sa is the specific surface area value (m2/g-adsorbent) of the adsorbent.
Application of adsorption process to actual flue gas treatment
The following assumptions are made in order to estimate the adsorptive removal of benzene in actual flue gas treatment. In such processing, the adsorption technique typically involves the spray injection of powdered material into the duct, with the average usage of powdered activated carbon being 100 mg/m3N for flue gas from incineration (Inoue, and Kawamoto Citation2009; Li et al. Citation2018). Based on this data, the effect of activated carbon used for actual flue gas treatment was estimated. The specific surface area of used activated carbon should be around 1,000 m2/g, and the concentration of benzene in gas should be 1 mg/m3N. The concentration may be slightly higher than it actually is, but it is broadly reasonable. In addition, the exhaust gas volume is set to 40,000 m3N/h, and this is a possible value for a waste incineration facility with a capacity of about 200 tons per day. The temperature should be based on 175°C.
Under these conditions, the benzene load per unit activated carbon volume is calculated to be 10 mg/g. On the other hand, the amount of benzene that can be adsorbed at this temperature is estimated to be 9.5 mg/g according to the Equationequation (5)(5)
(5) . Based on this estimation, the benzene removal efficiency at 175°C or below was almost 100%, but the efficiency gradually decreased above that temperature, becoming roughly 50% at 190°C. In addition, it is assumed that unburned carbonaceous materials with adsorption potential will coexist in practice; therefore, there will be extra capacity for adsorption removal.
Finally, from the viewpoint of material cost, relatively inexpensive materials, such as the activated coke investigated in this study, may be worth using because their performance in adsorption treatment was comparable to that of activated carbon, depending on the amount applied.
Summary
The experimental results of the study support the following conclusions:
The adsorption capacity of every carbonaceous material for the organic vapor decreased with the increasing temperature, with a roughly three-fold difference in the capacity, between 150°C and 190°C in dry gas. The adsorption amount of benzene in the high temperature and in low concentration range below 20 ppm was in the range 103 to 104 mg/kg-adsorbent.
The equilibrium adsorption amount of benzene and other hydrophobic organics can be calculated based on the specific surface area of the adsorbent under certain environmental conditions. Gas moisture reduced the equilibrium adsorption amount of the adsorbate by about half.
Based on the data of this study, the removal effect of benzene in an actual plant was estimated under slightly higher concentration conditions. According to this, it was pointed out that the adsorption and removal may be insufficient above 175°C. The exact process design should be made based on the scientific findings to be obtained in future practical studies.
Figure_S4..jpg
Download JPEG Image (16.9 KB)Figure_S3..jpg
Download JPEG Image (11.7 KB)Figure_S2..jpg
Download JPEG Image (4.6 KB)Figure_S1..jpg
Download JPEG Image (36.1 KB)Acknowledgment
The execution of experiments and data analysis in this study depended on the work ofXu Jiaxing and Hou Jianwei in graduate school of Environmental and Life Science at Okayama University. The author would like to thank them as well.
Disclosure statement
No potential conflict of interest was reported by the author(s).
Supplementary material
Supplemental data for this paper can be accessed on the publisher’s website.
Data availability statement
The author confirms that the data supporting the findings of this study are available within the article [and/or] its supplementary materials.
Additional information
Funding
Notes on contributors
Katsuya Kawamoto
Katsuya Kawamoto is a professor emeritus at Okayama University, Japan and was a professor in the Graduate School of Environmental and Life Science at the same University. He has made a major contribution in the field of environmental science and technology. His research was conducted in three main areas; 1) Study on environmental fate assessment of synthetic chemicals, which was conducted for halogenated organic compounds such as trichloroethylene and further organochlorine pesticides, 2) Study on dioxin formation and control in the process of thermal treatment of wastes, and 3) Development of a new thermal gasification and catalytic reforming process of biomass waste for recovering more energy and materials such as hydrogen.
References
- Baytar, O., Ö. Şahin, S. Horoz, and S. Kutluay. 2020. High-performance Gas-phase Adsorption of benzene and toluene on Activated Carbon: Response Surface Optimization, Reusability, Equilibrium, Kinetic, and Competitive Adsorption Studies. Environmental Science and Pollution Research 27 (21):26191–210. doi:https://doi.org/10.1007/s11356-020-08848-4.
- Chang, Y.-M., C.-Y. Hung, I.-H. Chen, C.-T. Chang, and C.-H. Chen. 2009. Minimum feeding rate of activated carbon to control dioxin emissions from a large-scale municipal solid waste incinerator. Journal of Hazardous Materials 161 (2–3):1436–43. doi:https://doi.org/10.1016/j.jhazmat.2008.04.128.
- Chen, J., Y. Zhang, T. Wang, H. Xu, P. Norris, and W.-P. Pan. 2018. Emission of volatile organic compounds (VOCs) during coal combustion at different heating rates. Fuel 225:554–62. doi:https://doi.org/10.1016/j.fuel.2018.03.185.
- Hu, J., M. Zheng, W. Liu, C. Li, Z. Nie, G. Liu, B. Zhang, K. Xiao, and L. Gao. 2013. Characterization of polychlorinated naphthalenes in stack gas emissions from waste incinerators. Environmental Science and Pollution Research 20 (5):2905–11. doi:https://doi.org/10.1007/s11356-012-1218-0.
- Hung, H.-W., and T.-F. Lin. 2007. Prediction of the adsorption capacity for volatile organic compounds onto activated carbons by the Dubinin-Raushkevich-Langmuir Model. Journal of the Air & Waste Management Association 57 (4):497–506. doi:https://doi.org/10.3155/1047-3289.57.4.497.
- Inoue, K., and K. Kawamoto. 2005. Fundamental adsorption characteristics of carbonaceous adsorbents for 1,2,3,4-tetrachlorobenzene in a model fas of an incineration plant. Environmental Science & Technology 39 (15):5844–50. doi:https://doi.org/10.1021/es0489745.
- Inoue, K., and K. Kawamoto. 2008. Adsorption characteristics of carbonaceous adsorbents for organic pollutants in a model incineration exhaust gas. Chemosphere 70 (3):349–57. doi:https://doi.org/10.1016/j.chemosphere.2007.07.067.
- Inoue, K., K. Yasuda, and K. Kawamoto. 2009. Atmospheric pollutants discharged from municipal solid waste incineration and gasification-melting facilities in Japan. Waste Management & Research: The Journal for a Sustainable Circular Economy 27 (6):617–22. doi:https://doi.org/10.1177/0734242X08096530.
- Kawamoto, K. 2009. Potential formation of PCDD/Fs and related bromine-substituted compounds from heating processes for ashes. Journal of Hazardous Materials 168 (2–3):641–48. doi:https://doi.org/10.1016/j.jhazmat.2009.02.068.
- Khan, F. I., and A. K. Ghoshal. 2000. Removal of volatile organic compounds from polluted air. Journal of Loss Prevention in the Process Industries 13 (6):527–45. doi:https://doi.org/10.1016/S0950-4230(00)00007-3.
- Lasker, I. I., Z. Hashisho, J. H. Phillips, J. E. Anderson, and M. Nochols. 2019. Modeling the effect of relative humidity on adsorption dynamics of volatile organic compound onto activated carbon. Environmental Science & Technology 53 (5):2647–59. doi:https://doi.org/10.1021/acs.est.8b05664.
- Li, G., Q. Wu, S. Wang, Z. Duan, H. Su, L. Zhang, Z. Li, Y. Tang, M. Zhao, L. Chen, et al. 2018. Improving flue gas mercury removal in waste incinerators by optimization of carbon injection rate. Environmental Science & Technology 52 (4):1940–45. doi:https://doi.org/10.1021/acs.est.7b05560.
- Ma, X., Z. Zhang, H. Wu, J. Li, and L. Yang. 2020. Adsorption of volatile organic compounds at medium-high temperature conditions by activated carbons. Energy & Fuels 34 (3):3679–90. doi:https://doi.org/10.1021/acs.energyfuels.9b03292.
- Ministry of the Environment Government of Japan −1, Dioxins 2012. (2021). Retrieved September 7, 2021, from http://www.env.go.jp/en/chemi/dioxins/brochure2012.pdf
- Ministry of the Environment Government of Japan −2, PRTR information plaza Japan. (2021). Retrieved September 7, 2021, from https://www.env.go.jp/en/chemi/prtr/prtr.html
- Nikam, S., and D. Mandal. 2020. Experimental study of the effect of different parameters on the adsorption and desorption of trichloroethylene vapor on activated carbon particles. ACS Omega 5 (43):28080–87. doi:https://doi.org/10.1021/acsomega.0c03648.
- Tomatis, M., H.-H. Xu, J. He, and X.-D. Zhang. 2016. Recent development of catalysts for removal of volatile organic compounds in flue gas by combustion: A review. Journal of Chemistry 8324826:15. Article ID.
- Waltisberg, J., and J. Weber. 2020. Disposal of waste-based fuels and raw materials in cement plants in Germany and Switzerland – What can be learned for global co-incineration practice and policy? Emerging Contaminants 6:93–102. doi:https://doi.org/10.1016/j.emcon.2020.02.001.
- Yan, M., X. Li, T. Chen, S. Lu, J. Yan, and K. Cen. 2010. Effect of temperature and oxygen on the formation of chlorobenzene as the indicator of PCDD/Fs. Journal of Environmental Sciences 22 (10):1637–42. doi:https://doi.org/10.1016/S1001-0742(09)60300-4.
- Yangyang, G., L. Yuran, Z. Tingyu, W. Jian, and Y. Meng. 2016. Modeling of dioxin adsorption on activated carbon. Chemical Engineering Journal 283:1210–15. doi:https://doi.org/10.1016/j.cej.2015.08.067.
- Yu, F. D., L. A. Luo, and G. Grevillot. 2002. Adsorption isotherms of VOCs onto and activated carbon monolith: Experimental measurement and correlation with different models. Journal of Chemical & Engineering Data 47 (3):467–73. doi:https://doi.org/10.1021/je010183k.
- Zhang, X., B. Gao, A. E. Creamer, C. Cao, and Y. Li. 2017. Adsorption of VOCs onto engineered carbon materials: A review. Journal of Hazardous Materials 338:102–23. doi:https://doi.org/10.1016/j.jhazmat.2017.05.013.