ABSTRACT
Leachate from landfills can be a significant challenge to manage and treat due to conventional contaminants. The addition of emerging contaminants such as per- and polyfluorinatedalkyl substances (PFASs) makes treatment even more complex. PFASs enter landfills through consumer waste and have been detected in landfill leachates at varying concentrations. The design and decision-making on leachate treatment require essential information since it depends on local factors, e.g. climate, proximity to wastewater treatment plants, and waste type. This study conducted a survey on actively operated public municipal solid waste (MSW) landfills in the Eastern and Northwestern regions of the US to understand the current leachate treatment practices and views from public MSW landfill managers on PFAS treatment. The survey aims to explore the possible adaptations from the industry to the pending regulatory guidelines for the potential PFASs treatment. Results show the majority of the landfills are currently using off-site disposal (72% of the responses), followed by complete onsite treatment (18% of the responses) and pre-treatment onsite and off-site disposal methods (10% of the responses). The factors that guided the selection of treatment methods included climate, economics, and future regulations. Evaporation and recirculation were the most prevalent onsite treatment technologies for public landfills, which reduced the leachate quantity for treatment. The public landfills expressed awareness of the potential impact of PFASs on the changes in leachate treatment. The current state-level regulation, potential federal PFAS regulation, and treatment costs are raising awareness of the onsite treatment for PFASs. The results of this study will benefit the improvement of PFAS awareness and provide critical information that will directly affect the leachate treatment process for PFASs.
Implications: This study presents a survey on the current leachate treatment process in the public municipal solid waste landfills in the eastern and northwestern U.S. and their potential process improvement on the impact of PFASs. This study is relevant to the topic of the JA&WMA because the research falls directly within the scope of this journal, and it documents the leachate treatment of landfills, and the results of this study will immediately contribute to our understanding of the waste treatment, benefiting the improvement of PFASs awareness, and providing critical information that will directly affect the leachate treatment process.
Introduction
Landfill leachate is the wastewater resulting from the percolation of rainfall through the waste, which combines with the moisture in the waste during landfill disposal and operation (Masoner et al. Citation2020). Landfill leachate is known to have a very complex composition, including (but not limited to) organic matter, ammonium, salts, and heavy metals (Martin et al. Citation2021; Vaverková et al. Citation2020). Due to its complexity, research on landfill leachate requires a multipronged approach, and various innovative techniques are being developed to address the complex nature of leachate. Co-treatment of leachate and municipal wastewater can be used to produce electricity between 50 and 527 mW/m3 (Saeed, Yadav, and Miah Citation2022). Numerical models are also implemented in parallel with experimental studies to develop cheaper and more efficient methods for landfill leachate and wastewater treatment (Boni et al. Citation2021). In addition to conventional contaminants, recently per- and polyfluoroalkyl substances (PFASs), also known as “forever chemicals”, were detected in landfill leachate (Masoner et al. Citation2020; Singh et al. Citation2021). PFASs are a large class of chemicals, typically defined by a fluorine atom bonded to the carbon atom in an organic molecule with carbon chains (Buck et al. Citation2011). The disposal of PFAS-containing products has become a major issue globally since it has been associated with several important human health and environmental issues (Brown et al. Citation2020). Due to the high mechanical, thermal, and chemical stability of PFASs, it is challenging to degrade naturally, causing them to be present in the environment for many years (Kwiatkowski et al. Citation2020; Masoner et al. Citation2020).
PFASs have been widely used in industrial and commercial applications such as surfactants, coatings, water repellents for leather and textiles, metal plating, and fire-fighting foams since the 1950s (Ritscher et al. Citation2018; Wei, Xu, and Zhao Citation2019). Consumer products and non-hazardous industrial wastes containing PFAS are discarded in municipal solid waste (MSW) landfills, while many PFAS-containing products eventually end up in landfills via direct disposal or wastewater treatment plants (WWTP) biosolids. Consequently, a range of PFAS concentrations have been reported in landfill leachate (Benskin et al. Citation2012; Li et al. Citation2012; Lindstrom et al. Citation2011; Yan et al. Citation2015). A study reported that perfluorooctanoic acid (PFOA) concentrations ranged between 0.15 and 9.2 μg L-1 in a US landfill leachate (Huset et al. Citation2011; Lang et al. Citation2017; Reinhart, Bolyard, and Chen Citation2023). Landfill leachate contains a complex mixture of PFASs and pharmaceutical chemicals, and leachate from landfills has been found to have the highest concentration of PFAS among environmental waters (Allred et al. Citation2014). Leachate in the US is commonly treated onsite or redirected to wastewater treatment plants (WWTPs) with or without pre-treatment, and the extent of treatment will depend on the landfill location, volume, quality, surrounding water bodies (i.e., direct discharge options), and availability of WWTPs (i.e., off-site treatment) (Masoner et al. Citation2020; Singh et al. Citation2021).
Although there was an interim guidance document published by the United States Environmental Protection Agency (EPA) in 2021 for non-consumer products, as of the survey date, there were no regulations for PFASs concentrations in landfill leachate before discharge to the disposal facilities, nor are there regulations to manage the disposal and destruction of these molecules (EPA Citation2022). Some of the short-chain PFASs are considered to be potential source of contamination due to higher uptake than longer chains. Recently, EPA announced the proposed National Primary Drinking Water Regulation (NPDWR) for 6 PFAS, including PFOA (MCL = 4 ppt), perfluorooctane sulfonic acid (PFOS, MCL = 4 ppt), perfluorononanoic acid (PFNA, hazardous index = 1), hexafluoropropylene oxide dimer acid (HFPO-DA, hazardous index = 1), perfluorohexane sulfonic acid (PFHxS, hazardous index = 1), and perfluorobutane sulfonic acid (PFBS, hazardous index = 1) (EPA Citation2023). These lower limits could eventually impact the leachate discharge levels upstream of WWTPs, and such change could begin that affect leachate treatment decisions by landfills and impact the costs of landfill operations.
Landfill leachate treatment practices, which may or may not include WWTPs, are essential in creating an outlet for PFASs from the ecosystem, which could be considered to be in alignment with the central restriction directive in the PFASs Strategic Roadmap of U.S. EPA (Citation2021). But the design and decision-making on leachate treatment, in general, requires essential information since it depends on local factors, e.g., climate, proximity to wastewater treatment plants and waste type (Viotti et al. Citation2020). Currently, there is a lack of information on the current landfill awareness and adaptation to the potential impact on the leachate treatment due to PFAS. Therefore, this study incorporated a treatment technology survey targeting the Eastern and Northwestern US to explore the possible adaptations from the industry to the pending regulatory guidelines for the potential PFAS treatment. The survey was aimed to collect information on leachate generation and treatment, including quantity, treatment type, and costs, and the potential impact of PFAS on treatment decisions. The collected information provides essential knowledge on the current leachate treatment techniques in the Eastern and Northwestern US and the adaptability of the landfill to the potential requirement for treatment PFAS. Furthermore, this study covers the preliminary requirement of understanding if the approaches of industries to treat leachate are changing and improving to meet the current or pending EPA regulations smoothly and sufficiently (EPA Citation2023).
Survey method
The target landfills were selected from the database compiled by the Landfill Methane Outreach Program (LMOP) of U.S. EPA. The LMOP database tracks key data for MSW landfills in the US, and it is a data repository consisting of more than 2,600 public and private MSW landfills that are either accepting MSW or closed in the past few decades. Landfills in the Eastern US (i.e., east of the Mississippi River) and the Northwestern US were selected to represent precipitation conditions of the US (total of 338 landfills). In the current survey, only public landfills under operation (i.e., no closed landfill) were surveyed. Five regions were defined based on the geological locations () and included: Northeast (including the states of Connecticut, Massachusetts, Maine, and New Hampshire), Mid-Atlantic (including the states of Delaware, Virginia, Pennsylvania, Maryland New Jersey, New York, and West Virginia), Midwest (including the states of Illinois, Indiana, Kentucky, Michigan, Ohio, and Wisconsin), Southeast (including the states of Alabama, Florida, Georgia, North Carolina, South Carolina, and Tennessee), and Northwest (including the states of Idaho, Oregon, and Washington). The survey was designed to focus on the following questions: 1) how much leachate was generated in 2021, 2) how the leachate was managed (treatment or disposal), 3) what the cost of leachate treatment or disposal was, and 4) how PFAS has changed the perspective of landfill managers on the onsite leachate treatment. The logical flow of the survey on the current leachate treatments of public MSW landfills and the potential PFAS impact in the Eastern and Northwestern US is shown in .
Figure 1. The five regions were surveyed during this study. The numbers by the region indicate the total number of landfills and the response rate of that region. Nine landfills did not provide their locations.
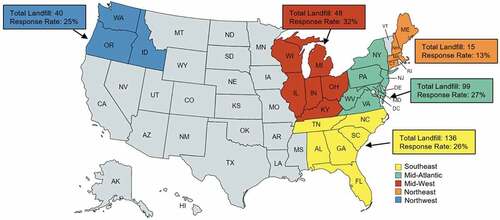
Figure 2. The logical flow of the survey on the current leachate treatments of public municipal solid waste landfills and the potential PFAS impact in the Eastern and Northwestern United States.
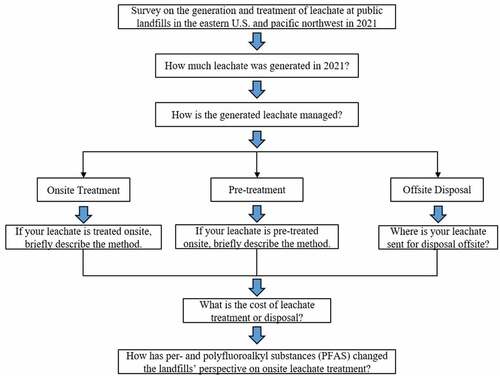
The questionnaires were created using Qualtrics (through the subscription by the University of Central Florida) and delivered to the landfill contacts via an e-mail with the link to the questionnaire. Follow-up e-mails were sent weekly to ensure a response rate, data collection, and quality. The responses from the survey were reviewed and analyzed using qualitative (e.g., treatment options and PFAS impact) and quantitative (e.g., cost of treatment and generation of leachate) evaluations. Qualitative analysis classified data without any quantitative value and tracked how many respondents chose each option and which option was selected mostly. Quantitative analysis was used to depict both the order and difference between values. This information was used to discuss trends and observations collectively across the Eastern and Northwestern US. shows the logical flow of the survey, and the survey questions and options are listed in the boxes in hierarchical order.
Results and discussion
Response rate breakdown by regions
In this survey, 101 landfills provided an effective response (i.e., the managers at least responded to the leachate generated, treatment methods, and cost questions), with the response rate from each participating region illustrated in . The overall response rate among the 338 analyzed MSW landfills was 30%. All five regions had a response rate higher than 25%, with the Northeast having the highest response rate (46%), augmented by the direct agency responses in addition to the survey responses. Additionally, nine landfills did not provide their locations. The overall response rate and the total number of calculations provided a confidence interval of 95% with an 8% error margin.
Leachate generation breakdown by regions
The leachate generated by landfills in each region is shown in . The leachate generated in 2021 was provided by the landfill managers and normalized with the tonnage of landfill waste-in-place (data from the LMOP) for 2020. Overall, 75 of the 101 landfills participating in the survey had the waste-in-place information available in the LMOP outreach program, with an average of 5,092,118 million tons. The Orange County landfill, Florida, had the maximum waste in place amount of nearly 34 million tons, while the Botetourt County landfill of Virginia accounted for the least amount of waste in place, which was 0.2 million tons. The landfills in the Southeast, Northwest, and Midwest showed a considerable amount of leachate produced with a mean value of 1.5 to 2.2 gallons per ton of waste; however, the Northwest had the lowest leachate production with ~ 0.5 gallons per ton of waste. No effective leachate production data were reported for the landfills in the Northeast due to the limited number of responses and the LMOP databases being out of date (i.e., waste-in-place data was not updated to 2020).
Figure 3. The leachate generated per ton of waste from the landfills in the five regions in the year 2021.
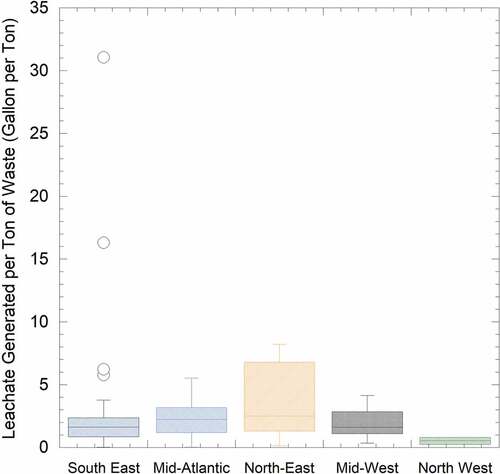
Several factors, other than landfill size and cover conditions, could have affected leachate production, such as climate, precipitation, and surface runoff (Adhikari, Dahal, and Nath Khanal Citation2008). For example, the mean total precipitation along the east coast of the United States showed relatively higher precipitation of 30 to 100 inches yr−1, especially in the Southeastern US (70 to 100 inches yr−1, SWANA Citation2014). However, out of the surveyed regions, the Northwest had a relatively drier climate with precipitation as low as 5 inches yr−1. Low precipitation could have been a key reason for the landfills in this region generating the lowest amount of leachate among all the other regions in addition to site-specific cover conditions ().
The disposal method of leachate in the public landfills
Three methods to treat leachate (i.e., complete onsite treatment, off-site treatment, and pre-treatment onsite and disposal off-site) were offered to the landfills in this survey. Based on the effective responses, 72% of the landfills selected off-site disposal as the principal leachate treatment method, 10% preferred the pre-treatment onsite and disposal off-site as the treatment of choice, while 18% of the landfills selected complete onsite treatment (). These results are consistent with the study report by the Environmental Research & Education Foundation on 445 landfills, (a), where approximately 72% of landfills are using off-site treatment, considering the standard error and confidence interval of the current study (Bolyard and Staley Citation2018).
Responded to onsite treatment technologies and costs
Among all the participating landfills, 17% of landfills (i.e., 17 landfills) opted for complete leachate treatment onsite. The treatment technologies included natural evaporation, thermal evaporation, recirculation, deep well injection, biological/ultrafiltration (UF) or reverse osmosis (RO), mixing with stormwater, and stabilization lagoon (). One of the most prevalent onsite treatment methods was natural evaporation (53% of the landfills used this onsite treatment method). Natural evaporation was primarily used in landfills located in the Northwest (blue regions in ) due to the relatively drier climate conditions. The treatment method of evaporation is a moderately cost-effective and low-energy solution for drier climate conditions and can vastly reduce the leachate quantity (Benyoucef et al. Citation2016). This data is consistent with previous findings by Wally, Jain, and Townsend (Citation2022) that reported landfills across the US (including privately- and public-owned landfills), where 21% of the landfills used natural evaporation as the leachate treatment method. This is especially prevalent in arid states, such as Idaho, where there are no incidents of leachate management other than climatic evaporation. Recirculation was the second most common treatment method, where 11% of the landfills used this onsite treatment approach. Other treatment methods, including leachate mixing with stormwater and deep well injection, were also found to relate to climate since these methods were reported in the Southeast of the US, where the precipitation is relatively high. Stormwater will dilute the leachate before discharge to the environment (Masoner et al. Citation2019).
presents the unit costs for each onsite treatment method as indicated by the participating landfills. The values were compared with the leachate treatment survey conducted by the Solid Waste Association-North America (SWANA Citation2014). The data from the current survey did not present comparable data to those reported by SWANA(Citation2014). Lower costs were reported in this study for natural evaporation, lagoon, and biological/UF/RO, while higher costs were reported for heat evaporation. Based on the return of the responders, the data listed here only included the operational cost, while capital cost may not have been considered or provided during the survey. Therefore, the returned cost data is likely to underestimate the actual cost of the leachate treatment methods. For the treatment cost comparisons, there was no inflation adjustment between the years 2014 and 2021.
Figure 6. Cost per gallon for each onsite application compared with data in SWANA (Citation2014).
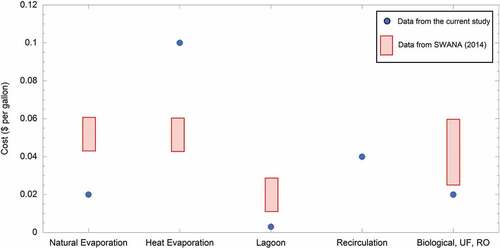
Responded to pre-treatment technologies and costs
Of the landfills that provided feedback, 10% preferred to pretreat their leachate onsite, followed by off-site discharge for further treatment. The strategies for the pre-treatment methods included mixing, aeration, pH adjustment, evaporation, sequencing batch reactor (SBR), biological treatment, and settling (). These techniques were used to reduce the heavy metals and nitrogen before discharging the leachate to the WWTPs or publicly owned treatment works (POTW) (Bolyard and Reinhart Citation2017; Renou et al. Citation2008). According to the returned cost data, the cost of pre-treatment varies with the technique and locations, ranging from 0.0038 to 0.25 dollars per gallon. Like the onsite treatment cost, most reported data did not provide detailed cost information. Therefore, the provided cost data should ideally include the operational and capital costs from onsite pre-treatment and charges from the off-site disposal or only the operational cost.
Table 1. The breakdown of pre-treatment technologies and the associated cost.
Responded disposal off-site and costs
Landfills that do not perform onsite treatment or pre-treatment with off-site disposal send their leachate to WWTPs, also referred to as “Off-site Treatment” in the survey. Landfills that selected off-site disposal were primarily affected by economic and regulatory considerations. Based on the feedback from the landfills, several managers indicated that their landfills are county-owned and can dispose of the leachate to the county-owned WWTPs at minimum or zero cost. Other responses also indicated site-specific situations in that the publicly owned WWTPs trade the biosolids with the landfill leachate. For off-site disposal, landfill costs typically included transpiration fees (either by truck or pipeline) and disposal costs to the WWTPs, which ranged from 0 to 0.21 dollars per gallon (). The transportation costs constituted the majority of the “Off-site Treatment” process costs, which ranged from 0 to 0.21 dollars per gallon (mean value of 0.03 dollars per gallon). This value was lower than that reported by SWANA (Citation2014), which ranged from 0.05 to 0.19 dollars per gallon for both public- and privately-owned landfills. The disposal cost in this study ranged from 0 to 0.1 dollars per gallon (mean value of 0.01 dollars per gallon), which was slightly higher than that reported by SWANA (Citation2014) of 0 to 0.02 dollars per gallon. The overall disposal cost for off-site treatment was 0 to 0.21 dollars per gallon based on the data from this survey.
Potential PFASs impact landfill leachate treatment in the Eastern and Northwestern US
The landfill managers responded to the question of whether PFASs changed the perspective regarding onsite treatment from landfill managers and 79% of the landfills offered effective responses ().
Figure 8. The responses from landfill managers on the potential PFASs impact on the landfill leachate treatment.
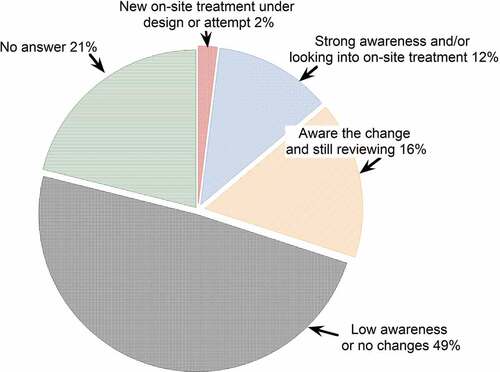
The responses were divided into four categories based on the comments and attitudes of the landfill managers. These categories included: A) landfills with new onsite treatment under design or attempt, B) landfills with solid awareness and/or looking into onsite treatment, C) landfills that are aware of the change and still reviewing the technologies/methods, and D) landfills with low awareness or no changes. In general, 30% of the landfills presented awareness of PFASs for leachate treatment (including categories A, B, and C), indicating that public landfills have noticed the potential impact of PFASs on leachate treatment. Only 2% of the landfills that responded to this survey were grouped into category A (i.e., new onsite treatment under design or attempt). The typical response was “currently under a design, permit and construct for onsite leachate treatment using a leachate thermal evaporator,” indicating a few public landfills are taking action for PFASs. Moreover, 12% of landfills that responded to this survey were grouped into category b (i.e., strong awareness and/or looking into onsite treatment). Typical responses included “We are now considering forms of onsite treatment as a risk management strategy,” “An evaporation pond appears to be the best option for us, even more so now with PFASs concerns,” and “PFASs are presently in our leachate and the Department of Environmental Protection (DEP) is beginning to indicate that onsite pre-treatment may soon be required.” These responses indicate potential regulation influences by the various state DEPs that are changing the leachate treatment options of landfills. A total of 16% of landfills that responded to this survey were grouped into category c (i.e., aware of the change and still reviewing). The typical responses were, “We are still studying the effects of PFAS,” “I think pre-treatment will be required within 2–5 years,” and “Sewage sludge was accepted until 1990. Grits accepted until 2009. Our current understanding of PFASs makes us very cautious about acceptance of WWTP solids ever again,” and “Our site has not to date incurred any cost for disposal or installed any pre-treatment systems for PFASs that may change following any regulatory changes.” Approximately half of the participating landfills had relatively low or no awareness of the PFAS problems. Typical responses included “PFASs have not yet impacted our treatment operations,” “Don’t know much about PFASs,” and “Nothing yet.”, “Until we understand what regulators will require, there is not much that can be done.” These responses indicate that the surveyed landfills might not have considered the impact of PFAS or may not want to change their attitude/opinion of the potential PFAS impacts.
Current PFASs treatment technologies
Currently, various research is conducted to evaluate treatment methods for PFAS in landfill leachate. Although landfill leachate is considered to be treated correctly (Benskin et al. Citation2012; Yan et al. Citation2015), it is unknown if this practice has measurable effects on PFAS concentrations and loads on receiving WWTPs and the corresponding effluents discharged into surface water (Masoner et al. Citation2020). This is likely due to the limited number of studies that have considered the fate of PFASs throughout leachate handling processes (Busch et al. Citation2010; Yan et al. Citation2015).
Typically, landfill leachate is treated off-site through conventional activated sludge processes in WWTPs. However, biological processes are ineffective at degrading PFASs (Allred et al. Citation2015; Schultz et al. Citation2006). While membrane bioreactors have been commonly employed in treating landfill leachate, they are also inefficient at eliminating PFASs from landfill leachate (Fuertes et al. Citation2017). PFASs are persistent against oxidation-reduction, volatilization, and photolytic, microbial, and metabolic degradations (Schultz, Barofsky, and Field Citation2003; Takagi et al. Citation2011; Xiao, Simcik, and Gulliver Citation2013). Thus, conventional treatment processes are not efficient in removing PFASs from water (Eschauzier et al. Citation2012; Takagi et al. Citation2011; Xiao, Simcik, and Gulliver Citation2013). Membrane processes can serve as effective barriers to PFASs and inorganic contaminants. Reverse osmosis and nanofiltration are highly effective in separating PFASs regardless of chain length (Boo et al. Citation2018) and are also expected to be effective in removing many types of PFAS precursors. However, membrane systems are expensive and typically employed for large-scale drinking water systems. Among the available treatment technologies, sorption methods such as granulated activated carbon (GAC) and ion exchange (IX) have been widely applied to eliminate various PFASs from water. However, they merely collect and concentrate the compounds rather than degrading or altering them, requiring disposal of the environmental matrices (Niu et al. Citation2016). Mainly, IXs are effective for either long- or short-chain PFASs, and more novel resins are reported to have higher sorption capacities for PFASs compared with GAC (Zaggia et al. Citation2016). However, they are more expensive than GAC per unit weight and often require pre-treatment. The RO, GAC, and IX technologies separate the contaminants rather than destroying them, which brings up the issue of the disposal of residuals and, in turn, the transfer of concentrated biosolids to solid waste disposal (Gallen et al. Citation2018; Hamid, Li, and Grace Citation2018; Mahinroosta and Senevirathna Citation2020; Shoeib et al. Citation2016). Therefore, it is crucial to continuously develop new treatment technologies that are technologically and cost-effective.
Conclusion
In this study, a survey was conducted on actively operated public MSW landfills in the Eastern and Northwestern regions of the US to understand the current leachate treatment techniques, views of public MSW landfill management on PFAS treatment, and to explore the possible adaptations from the industry to the pending regulatory guidelines for the potential PFASs treatment. Additionally, this survey aims to provide a frame for researchers who intend to develop methods for effective PFAS destruction. Public landfills in the Eastern and Northwestern regions of US were selected and contacted from the database compiled by the LMOP of U.S. EPA. The survey was designed to focus on the leachate generation, treatment method, treatment cost, and the view of the landfill manager on the treatment of PFAS. Based on the results of the current survey, several conclusions can be drawn:
The majority of the landfills in the Eastern and Northwestern regions of the US use off-site disposal. This decision is affected by the climate conditions, economics, and regulations.
Evaporation and recirculation are the most prevalent onsite treatment technologies for public landfills. This is consistent with a current EPA study where natural evaporation and recirculation were widely used in public and private landfills.
Off-site disposal costs, in general, agreed with the literature. However, the transportation cost may have been underestimated by the site managers in the current study. Onsite treatment costs from this survey did not match well with the literature, likely due to underestimating the capital cost for onsite treatment technologies.
The public landfills expressed awareness of the potential impact of PFASs on the changes in leachate treatment. Based on the comments from the landfill manager, the pressure from the current state-level regulations, potential federal PFAS regulation, and treatment costs are raising awareness of the onsite treatment for PFASs.
Nomenclature
DEP | = | Department of Environmental Protection |
EREF | = | Environmental Research & Education Foundation |
GAC | = | Granulated Activated Carbon |
HFPO-DA | = | Hexafluoropropylene Oxide Dimer Acid |
IX | = | Ion Exchange |
LMOP | = | Landfill Methane Outreach Program |
MSW | = | Municipal Solid Waste |
NPDES | = | National Pollutant Discharge Elimination System |
PFASs | = | Polyfluorinatedalkyl Substances |
PFBS | = | Perfluorobutane Sulfonic Acid |
PFOA | = | Perfluorooctanoic Acid |
PFOS | = | Perfluorooctane Sulfonic Acid |
POTW | = | Publicly Owned Treatment Works |
RO | = | Reverse Osmosis |
SBR | = | Sequencing Batch Reactor |
SWANA | = | Solid Waste Associationtion of North America |
UF | = | Ultrafiltration |
U.S. EPA | = | United States Environmental Protection Agency |
WWTP | = | Wastewater Treatment Plant |
Disclosure statement
No potential conflict of interest was reported by the author(s).
Data availability statement
The data that support the findings of this study are available on request from the corresponding author [JC]. The data are not publicly available since it contains information that could compromise the privacy of research participants.
Additional information
Notes on contributors
Mert Gokgoz
Mert Gokgoz is a Ph.D. student in the Department of Civil, Environmental, and Construction Engineering, University of Central Florida, Orlando, FL.
Wuhuan Zhang
Wuhuan Zhang is a Ph.D. candidate in the Department of Civil and Environmental Engineering, University of Virginia, Charlottesville, VA.
Nimna Manage
Nimna Manage is an M.S. student in the Department of Civil, Environmental, and Construction Engineering, University of Central Florida, Orlando, FL.
Mery Mbengue
Mery Mbengue is an undergraduate student in the Department of Civil, Environmental, and Construction Engineering, University of Central Florida, Orlando, FL.
Stephanie Bolyard
Stephanie Bolyard is a Senior Engineer to the Assistant Secretary of the North Carolina Department of Environmental Quality, Raleigh, NC.
Jiannan Chen
Jiannan Chen is an Assistant Professor in the Department of Civil, Environmental, and Construction Engineering, University of Central Florida, Orlando, FL.
References
- Adhikari, B., K.R. Dahal, and S. Nath Khanal. 2008. A review of factors affecting the composition of municipal solid waste landfill leachate reconstruction project view project anaerobic digestion of waste view project a review of factors affecting the composition of municipal solid waste landfill leachate. Certified Int. J. Eng. Sci. Innovative Technol. (IJESIT) 9001 (5). https://www.researchgate.net/publication/269397323.
- Allred, B.M., J.R. Lang, M.A. Barlaz, and J.A. Field. 2015. Physical And Biological Release of Poly-and Perfluoroalkyl Substances (PFASs) from Municipal Solid Waste In Anaerobic Model Landfill Reactors. http://pubs.acs.org.
- Allred, B.M.K., J.R. Lang, M.A. Barlaz, and J.A. Field. 2014. Orthogonal zirconium diol/C18 liquid chromatography-Tandem mass spectrometry analysis of poly and perfluoroalkyl substances in landfill leachate. J. Chromatogr. A 1359:202–11. doi:10.1016/j.chroma.2014.07.056.
- Benskin, J.P., B. Li, M.G. Ikonomou, J.R. Grace, and L.Y. Li. 2012. Per- and polyfluoroalkyl substances in landfill leachate: Patterns, time trends, and sources. Environ. Sci. Technol. 46 (21):11532–40. doi:10.1021/es302471n.
- Benyoucef, F., A. Makan, A. El Ghmari, and A. Ouatmane. 2016. Optimized evaporation technique for leachate treatment: Small scale implementation. J. Environ. Manage. 170:131–35. doi:10.1016/j.jenvman.2015.12.004.
- Bolyard, S.C., and D.R. Reinhart. 2017. Evaluation of leachate dissolved organic nitrogen discharge effect on wastewater effluent quality. Waste Manage. 65:47–53. doi:10.1016/j.wasman.2017.03.025.
- Bolyard, S.C., and B. Staley. 2018. State of Practice of Leachate Management and Treatment in the US, A presentation at the Global Waste Management Symposium, Indian Wells, California, February 11–14.
- Boni, M.R., S. Marzeddu, F. Tatti, M. Raboni, G. Mancini, A. Luciano, and P. Viotti. 2021. Experimental and numerical study of biochar fixed bed column for the adsorption of arsenic from aqueous solutions. Water (Switzerland) 13 (7):915. doi:10.3390/w13070915.
- Boo, C., Y. Wang, I. Zucker, Y. Choo, C.O. Osuji, and M. Elimelech. 2018. High performance nanofiltration membrane for effective removal of perfluoroalkyl substances at high water recovery. Environ. Sci. Technol. 52 (13):7279–88. doi:10.1021/acs.est.8b01040.
- Brown, J.B., J.M. Conder, J.A. Arblaster, and C.P. Higgins. 2020. Assessing human health risks from per-and polyfluoroalkyl substance (PFAS)-impacted vegetable consumption: A tiered modeling approach. Environ. Sci. Technol. 54 (23):15202–14. doi:10.1021/acs.est.0c03411.
- Buck, R.C., J. Franklin, U. Berger, J.M. Conder, I.T. Cousins, V.P. De, A.A. Jensen, K. Kannan, S.A. Mabury, and S.P.J. van Leeuwen. 2011. Perfluoroalkyl and polyfluoroalkyl substances in the environment: Terminology, classification, and origins. Integr. Environ. Assess. Manage. 7 (4):513–41. doi:10.1002/ieam.258.
- Busch, J., L. Ahrens, R. Sturm, and R. Ebinghaus. 2010. Polyfluoroalkyl compounds in landfill leachates. Environ. Pollut. 158 (5):1467–71. doi:10.1016/j.envpol.2009.12.031.
- Eschauzier, C., E. Beerendonk, P. Scholte-Veenendaal, and P. De Voogt. 2012. Impact of treatment processes on the removal of perfluoroalkyl acids from the drinking water production chain. Environ. Sci. Technol. 46 (3):1708–15. doi:10.1021/es201662b.
- Fuertes, I., S. Gómez-Lavín, M.P. Elizalde, and A. Urtiaga. 2017. Perfluorinated alkyl substances (PFASs) in northern Spain municipal solid waste landfill leachates. Chemosphere 168:399–407. doi:10.1016/j.chemosphere.2016.10.072.
- Gallen, C., G. Eaglesham, D. Drage, T.H. Nguyen, and J.F. Mueller. 2018. A mass estimate of perfluoroalkyl substance (PFAS) release from Australian wastewater treatment plants. Chemosphere 208:975–83. doi:10.1016/j.chemosphere.2018.06.024.
- Hamid, H., L.Y. Li, and J.R. Grace. 2018. Review of the fate and transformation of per- and polyfluoroalkyl substances (PFASs) in landfills. Environ. Poll., 235:74–84. Elsevier Ltd. doi:10.1016/j.envpol.2017.12.030.
- Huset, C.A., M.A. Barlaz, D.F. Barofsky, and J.A. Field. 2011. Quantitative determination of fluorochemicals in municipal landfill leachates. Chemosphere 82 (10):1380–86. doi:10.1016/j.chemosphere.2010.11.072.
- Kwiatkowski, C.F., D.Q. Andrews, L.S. Birnbaum, T.A. Bruton, J.C. Dewitt, D.R.U. Knappe, M.V. Maffini, M.F. Miller, K.E. Pelch, A. Reade, et al. 2020. Scientific basis for managing PFAS as a chemical class. Environ. Sci. Technol. Lett. Am. Chem. Soc. 7 (8):532–43. doi:10.1021/acs.estlett.0c00255.
- Lang, J.R., B.M.K. Allred, J.A. Field, J.W. Levis, and M.A. Barlaz. 2017. National Estimate of Per- and Polyfluoroalkyl Substance (PFAS) release to US municipal landfill leachate. Environ. Sci. Technol. 51 (4):2197–205. doi:10.1021/acs.est.6b05005.
- Li, B., M.N. Danon-Schaffer, L.Y. Li, M.G. Ikonomou, and J.R. Grace. 2012. Occurrence of PFCs and PBDEs in landfill leachates from across Canada. Water Air Soil Pollut. 223 (6):3365–72. doi:10.1007/s11270-012-1115-7.
- Lindstrom, A.B., M.J. Strynar, A.D. Delinsky, S.F. Nakayama, L. McMillan, E.L. Libelo, M. Neill, and L. Thomas. 2011. Application of WWTP biosolids and resulting perfluorinated compound contamination of surface and well water in Decatur, Alabama, USA. Environ. Sci. Technol. 45 (19):8015–21. doi:10.1021/es1039425.
- Mahinroosta, R., and L. Senevirathna. 2020. A review of the emerging treatment technologies for PFAS contaminated soils. J. Environ. Manage., 255. Academic Press. doi:10.1016/j.jenvman.2019.109896.
- Martin, K.R., N.M. Robey, S. Ma, L.C. Powers, A. Heyes, P. Schmitt-Kopplin, W.J. Cooper, T.G. Townsend, and M. Gonsior. 2021. Characterization of landfill leachate molecular composition using ultrahigh resolution mass spectrometry. Environ. Sci. 7 (7):1250–66. doi:10.1039/D1EW00020A.
- Masoner, J.R., D.W. Kolpin, I.M. Cozzarelli, L.B. Barber, D.S. Burden, W.T. Foreman, K.J. Forshay, E.T. Furlong, J.F. Groves, M.L. Hladik, et al. 2019. Urban stormwater: An overlooked pathway of extensive mixed contaminants to surface and groundwaters in the United States. Environ. Sci. Technol. 53 (17):10070–81. doi:10.1021/acs.est.9b02867.
- Masoner, J.R., D.W. Kolpin, I.M. Cozzarelli, K.L. Smalling, S.C. Bolyard, J.A. Field, E.T. Furlong, J.L. Gray, D. Lozinski, D. Reinhart, et al. 2020. Landfill leachate contributes per-/poly-fluoroalkyl substances (PFAS) and pharmaceuticals to municipal wastewater. Environ. Sci. 6 (5):1300–11. doi:10.1039/d0ew00045k.
- Niu, J., Y. Li, E. Shang, Z. Xu, and J. Liu. 2016. Electrochemical oxidation of perfluorinated compounds in water. Chemosphere, 146:526–38. Elsevier Ltd. doi:10.1016/j.chemosphere.2015.11.115.
- Reinhart, D.R., S.C. Bolyard, and J. Chen. 2023. Fate of per- and polyfluoroalkyl substances in postconsumer products during waste management. J. Environ. Eng. 149 (4). doi: 10.1061/joeedu.eeeng-7060.
- Renou, S., J.G. Givaudan, S. Poulain, F. Dirassouyan, and P. Moulin. 2008. Landfill leachate treatment: Review and opportunity. J. Hazard. Mater. 150 (3):468–93. doi:10.1016/j.jhazmat.2007.09.077.
- Ritscher, A., Z. Wang, M. Scheringer, J.M. Boucher, L. Ahrens, U. Berger, S. Bintein, S.K. Bopp, D. Borg, A.M. Buser, et al. 2018. Zürich statement on future actions on per-and polyfluoroalkyl substances (PFASs). Environ. Health Perspect. 126 (8). doi:10.1289/EHP4158.
- Saeed, T., A.K. Yadav, and M.J. Miah. 2022. Landfill leachate and municipal wastewater co-treatment in microbial fuel cell integrated unsaturated and partially saturated tidal flow constructed wetlands. J. Water Process Eng. 46:102633. doi:10.1016/j.jwpe.2022.102633.
- Schultz, M.M., D.F. Barofsky, and J.A. Field. 2003. Fluorinated alkyl surfactants. Environ. Eng. Sci. 20 (5):487–501. doi:10.1089/109287503768335959.
- Schultz, M.M., C.P. Higgins, C.A. Huset, R.G. Luthy, D.F. Barofsky, and J.A. Field. 2006. Fluorochemical mass flows in a municipal wastewater treatment facility. Environ. Sci. Technol. 40 (23):7350–57. doi:10.1021/es061025m.
- Shoeib, T., Y. Hassan, C. Rauert, and T. Harner. 2016. Poly- and perfluoroalkyl substances (PFASs) in indoor dust and food packaging materials in Egypt: Trends in developed and developing countries. Chemosphere 144:1573–81. doi:10.1016/j.chemosphere.2015.08.066.
- Singh, R.K., E. Brown, S. Mededovic Thagard, and T.M. Holsen. 2021. Treatment of PFAS-containing landfill leachate using an enhanced contact plasma reactor. J. Hazard. Mater. 408:124452. doi:10.1016/j.jhazmat.2020.124452.
- SWANA Applied Research Foundation. 2014. Onsite Treatment of Landfill Leachate. Solid Waste Association of North America. https://store.swana.org/store/detail.aspx?id=ARF14OSTLL.
- Takagi, S., F. Adachi, K. Miyano, Y. Koizumi, H. Tanaka, I. Watanabe, S. Tanabe, and K. Kannan. 2011. Fate of Perfluorooctanesulfonate and perfluorooctanoate in drinking water treatment processes. Water Res. 45 (13):3925–32. doi:10.1016/j.watres.2011.04.052.
- U.S. Environmental Protection Agency. 2021. PFAS Strategic Roadmap: Epa’s Commitments To Action 2021–2024. https://www.epa.gov/system/files/documents/2021-10/pfas-roadmap_final-508.pdf.
- U.S. Environmental Protection Agency. 2022. Addressing PFAS Discharges in NPDES Permits and Through The Pre-Treatment Program and Monitoring Programs. https://www.epa.gov/system/files/documents/2022-12/NPDES_PFAS_State%20Memo_December_2022.pdf.
- U.S. Environmental Protection Agency. 2023. Proposed PFAS National Primary Drinking Water Regulation. https://www.epa.gov/sdwa/and-polyfluoroalkyl-substances-pfas.
- Vaverková, M.D., J. Elbl, E. Koda, D. Adamcová, A. Bilgin, V. Lukas, A. Podlasek, A. Kintl, M. Wdowska, M. Brtnický, et al. 2020. Chemical composition and hazardous effects of leachate from the active municipal solid waste landfill surrounded by farmlands. Sustainability (Switzerland) 12 (11):4531. doi:10.3390/su12114531.
- Viotti, P., F. Tatti, A. Rossi, A. Luciano, S. Marzeddu, G. Mancini, and M.R. Boni. 2020. An eco-balanced and integrated approach for a more-sustainable MSW management. Waste Biomass Valorization 11 (10):5139–50. doi:10.1007/s12649-020-01091-5.
- Wally, J., P. Jain, and T. Townsend. 2022. State of the practice of onsite leachate treatment at municipal solid waste landfills. SWANA FL Summer Conference, Naples, FL, July 25.
- Wei, Z., T. Xu, and D. Zhao. 2019. Treatment of per- and polyfluoroalkyl substances in landfill leachate: Status, chemistry and prospects. Environ. Sci. 5 (11):1814–35. doi:10.1039/c9ew00645a.
- Xiao, F., M.F. Simcik, and J.S. Gulliver. 2013. Mechanisms for removal of perfluorooctane sulfonate (PFOS) and perfluorooctanoate (PFOA) from drinking water by conventional and enhanced coagulation. Water Res. 47 (1):49–56. doi:10.1016/j.watres.2012.09.024.
- Yan, H., I.T. Cousins, C. Zhang, and Q. Zhou. 2015. Perfluoroalkyl acids in municipal landfill leachates from China: Occurrence, fate during leachate treatment and potential impact on groundwater. Sci. Total Environ. 524–525:23–31. doi:10.1016/j.scitotenv.2015.03.111.
- Zaggia, A., L. Conte, L. Falletti, M. Fant, and A. Chiorboli. 2016. Use of strong anion exchange resins for the removal of perfluoroalkylated substances from contaminated drinking water in batch and continuous pilot plants. Water Res. 91:137–46. doi:10.1016/j.watres.2015.12.039.