ABSTRACT
This paper presents a review on more than hundred years of palaeoenvironmental research in Sweden; from early descriptions of peat and tufa deposits in the late nineteenth and early twentieth centuries to multiproxy transfer function studies in the late twentieth and early twenty-first centuries CE. Research on Holocene climate variability has a long history in Sweden and many ideas and concepts about changes in temperature and precipitation during the Holocene originated in Fennoscandia. The Holocene climate evolution in Sweden follows a pattern in common for many northern latitude records with a rapid warming starting at the Pleistocene–Holocene boundary at c. 11 650 cal a BP, followed by the middle Holocene thermal maximum between c. 8000 and 5000 cal a BP. A change to colder and wetter conditions starts c. 4000 cal a BP and lasts until the late 1800 s CE. There is evidence for climatic anomalies such as the 8.2 and 4.2 ka BP events and the Little Ice Age (LIA) but only inconclusive evidence for other events, such as the 10.3 ka BP event. The main pattern of Holocene climate and environmental evolution is well known for most parts of Sweden, but the present review also shows that several research questions remain to be addressed.
Introduction
Research on Holocene climate variability goes back more than hundred years in Sweden, and many of the ideas and concepts about changes in temperature and precipitation during the Holocene originated in Fennoscandia (Birks & Seppä Citation2010). An early source of palaeoenvironmental information was tufa deposits, particularly because of their records of well-preserved mega and macrofossils (Hulth Citation1895; Sernander Citation1915; Gedda Citation2006), but today many tufa deposits have been completely excavated. The early part of the 1900s produced a number of studies of peat-bogs and lakes that are now considered classic and influential works (e.g., Sernander Citation1908; von Post Citation1924; Granlund Citation1932; Lundqvist Citation1940). The Holocene vegetational development was first reconstructed by macrofossil analysis (Andersson Citation1909) and later on through the newly developed pollen analysis (von Post Citation1916). After the Second World War, studies of tree-rings, speleothems, lake sediments, bogs, glaciers and other proxy methods have provided more information about the Holocene climate evolution in Sweden, and dating methods such as radiocarbon, tree-rings, lacustrine varves, optically-stimulated luminescence (OSL) and terrestrial cosmogenic nuclide (TCN) dating have allowed the climate fluctuations and events to be dated. During the c. last 20 years, transfer-function-based methods have provided quantitative climate estimates to many lake and bog records (e.g., Rosén et al. Citation2001; Bigler et al. Citation2002).
The Holocene climate evolution in Sweden follows a pattern in common for many northern latitude records with a rapid warming starting at the Pleistocene–Holocene boundary at c. 11 650 cal a BP, followed by an early to mid-Holocene thermal maximum (HTM) between c. 8000 and 5000 cal a BP (e.g., Mayewski et al. Citation2004; Snowball et al. Citation2004). A transition to colder and wetter conditions started at c. 5500 cal a BP and culminated during the Little Ice Age c. 1300-late 1800s CE, followed by a rapid warming during the last c. 100–120 years. Short climate events are superimposed on these general trends, e.g., the 8.2 ka BP event and the Medieval Warm Period between c. 800 and 1300 CE.
The aim of the paper is to present a broad review of Holocene climate and environmental changes in Sweden, based on more than 125 years of palaeoenvironmental research including some archaeological and historical data. Most studies have focused on one or two proxy methods in a restricted area, but this is an attempt to include data from several different methods and regions in order to present a review of the Holocene evolution of Sweden. For historical reviews of Late Quaternary research in Fennoscandia and Sweden in particular, the reader is also referred to the works of Lundqvist (Citation1958) and Birks & Seppä (Citation2010).
The postglacial development of the Baltic Sea still affects countries surrounding the Baltic Sea basin. Low-lying areas have gradually been uplifted above the sea surface during the Holocene, and this development will continue, especially in the northern part of the basin where the glacioisostatic uplift is higher than the Global Sea level rise (e.g., Vestøl et al. Citation2019). The postglacial development of the Baltic Sea will not, however, be treated in the present study and the reader is referred to comprehensive reviews by Björck (Citation1995) and Andrén et al. (Citation2011).
The sub-division of the Holocene in this paper follows the formal stratigraphic division by the International Commission on Stratigraphy (ICS) (; Walker et al. Citation2019). Accordingly, the Holocene is divided into three stages, the Early Holocene (Greenlandian; 11 700–8236 a b2k), the Middle Holocene (Northgrippian; 8236–4250 a b2k) and the late Holocene (Meghalayan; 4250 a b2k-present). The literature review is thus divided into three chapters. Age brackets in Walker et al. (Citation2019) are reported as relative to a baseline of 2000 CE (b2k), but in the present paper calendar years BP (cal a relative to 1950 CE) will be used consistently since the vast majority of papers have reported ages using this timescale. All radiocarbon dates from published sources have been calibrated to calendar years using OxCal v4.4.2. (Bronk Ramsey Citation2013), applying the IntCal20 calibration curve (Reimer et al. Citation2020). Ages > 10 000 years BP are generally given as “kiloyears = ka”, while ages < 10 000 years are given with their full numbers, with exceptions for established events such as the 8.2 ka BP event.
Figure 1. Different subdivisions of the Holocene Epoch. A. July insolation at 60°N. B. The Blytt–Sernander scheme for postglacial climatic changes and the Younger Dryas as defined by Mangerud et al. (Citation1974). Boundaries originally defined in radiocarbon years have been calibrated to calendar years BP. C. Archaeological periods in south Sweden (Price Citation2015). The Stone Age is divided in three parts, the Palaeolithic (c. 12 000–9500 BCE), the Mesolithic (c. 9500–4000 BCE) and the Neolithic (c. 4000–1700 BCE); the Bronze Age (1700-1500 BCE); the Iron Age (500 BCE-1050 CE), including the Viking Age (c 800–1066 CE). The last c. 1000 years can be divided into the Medieval period (c. 1066–1500 CE), the Post-Medieval period (c. 1500-c. 1800 CE) and the industrial/modern time (c. 1800 CE-present). D. Cold events during the Holocene (see text for explanation). E. Warm events during the Holocene (see text for explanation). F. The Holocene stages defined by ICS (Walker et al. Citation2018).
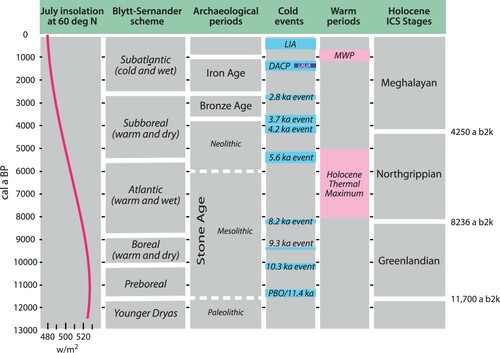
Present-day climate and environmental settings
Sweden has a temperate climate, despite its northern latitude and approximately 15% of the land area of 450 000 km2 is situated north of the Arctic circle. This means that several climate zones and forest zones are represented, with a mainly oceanic climate in the south, humid continental in the central parts and subarctic climate or tundra in the north. Sweden is influenced by the Gulf Stream and the North Atlantic Current and the predominantly westerly winds. The climate is in general mild for its latitude but vary from the south to the north due to the vast latitudinal difference. Forests in southern Sweden belong to the nemoral or boreo-nemoral zones, and middle and north Sweden are part of the boreal forests, also known as the northern taiga. The Scandinavian Mountains or the Scandes run through the Scandinavian Peninsula, and the highest peak in Sweden is Kebnekaise at c. 2100 m a.s.l. Mountain glaciers occur from the county of Jämtland in the south to the Torneträsk area in the north, but a majority have retreated from their maximum Holocene positions. The present tree-line ranges from c. 1150 to 1200 m a.s.l. in northern Dalarna to 550–600 m a.s.l. in northernmost Sweden (Andersson et al. Citation1983; Kullman Citation2004). Tundra with permafrost peatlands are found north of c. 67°N (Sannel et al. Citation2018).
The Early Holocene
Southern Sweden, south of the Younger Dryas end-moraine zone, was deglaciated between c. 17 and 12 ka BP (e.g., Berglund Citation1979; Lundqvist & Wohlfarth Citation2000; Stroeven et al. Citation2016), but the climate and environmental changes during this dynamic period are outside the scope in this study.
The Younger Dryas in Sweden ended shortly after the final drainage of the Baltic Ice Lake at Mt. Billingen () to the sea-level c. 11.7 ka BP, and was followed by a rapid retreat of the Fennoscandian Ice Sheet (FIS) and a distinct increase in sedimentation rate and varve thickness of glacial clays during the Early Holocene (e.g., Andrén et al. Citation2011). A common view is that the general warming at the Younger Dryas-Holocene boundary spread synchronously over the North Atlantic region (e.g., Björck et al. Citation1996; Gulliksen et al. Citation1998). This view has, however, recently been challenged by e.g., Wohlfarth et al. (Citation2017) suggesting more complex spatial and temporal environmental and hydrological changes in northern Europe across this transition.
Figure 2. (Colour online) Sites in southern Sweden with evidence for the early Holocene warming and/or the PBO/11.4 ka BP event. Light blue = ice margin at c. 11.7 ka BP; light grey = areas above the Baltic Ice Lake and the sea-level at c. 11.7 ka BP.
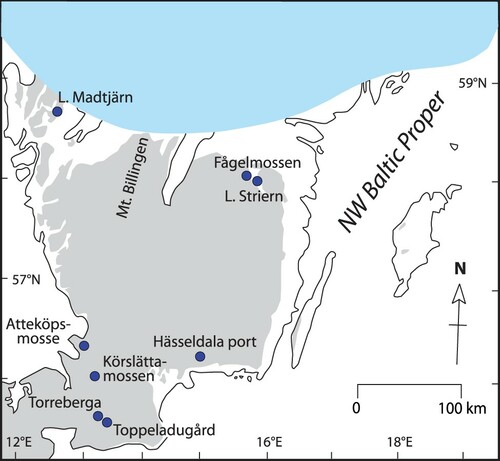
The timing of the Holocene warming and whether it was time-transgressive or not has been discussed extensively recently (e.g., Pearce et al. Citation2013; Wohlfarth et al. Citation2017). Some of the best and most complete records of the early Holocene warming in Sweden have been inferred from beetle records from southern Sweden, showing a mean summer temperature increase from c. 10° to 14–16° C within a few decades and a rapid replacement of Arctic-Subarctic species by Boreal species (Lemdahl Citation1991; Hammarlund & Lemdahl Citation1994). It has been suggested that the warming occurred at the Younger Dryas-Early Holocene boundary but with some chronological uncertainties. The Toppeladugård site, S Sweden () investigated by Lemdahl (Citation1991), is, however, relatively poorly dated (). A chronologically better constrained site, Hässeldala port, SE Sweden (; Wohlfarth et al. Citation2017) shows a similar response to the warming but with an offset of several hundred years to the end of the GS-1Footnote1 (approximately equivalent to the Younger Dryas) on Greenland (). The warming occurs at ca. 11.9 ka BP at Hässeldala port, c. 200 years earlier than the transition into the Holocene on Greenland (Walker et al. Citation2019). At this site, chironomid-inferred summer temperatures rose from 10° to >12°C at c. 11.9 ka BP. A similar chironomid-based summer temperature reconstruction from Atteköpsmosse in SW Sweden () shows an increase in summer temperatures from 10 to 14° C at 11.6–11.5 ka BP (Wohlfarth et al. Citation2018). It is uncertain, however, if the different timings of the response of the early Holocene warming are real or due to chronological inaccuracies. The Hässeldalen tephra, c. 11.4 ka BP (Davies et al. Citation2003) could provide an independent tool to assess the response to the early Holocene warming; i.e., whether the climate warming was synchronous or time-transgressive across southern Scandinavia. This tephra has been reported from both sites, but minor offsets in geochemistry between published records of the Hässeldalen tephra at the type site Hässeldala port and the tephra at Atteköpsmosse cast some doubts over the tephra identification at the latter site. However, a recent study focusing on climate indicator species (Iversen Citation1954) shows that July temperatures were surprisingly high in southern Sweden and Denmark with c. 16° during the pre-Bølling, Allerød, Younger Dryas and Preboreal (Schenk et al. Citation2020), several degrees warmer than previous beetle-based temperature estimates (; e.g., Lemdahl Citation1991; Hammarlund & Lemdahl Citation1994). The onset of the Holocene was marked by an abrupt decrease in cold-adapted plants rather than an increase in “warm” species. Further to the East, there is evidence for a delayed onset of the early Holocene warming in NW Russia and the eastern Baltic region compared with southern Swedish records (Subetto et al. Citation2002).
Table 1. Examples of studies reporting evidence for the early Holocene warming in Sweden (from south to north). *age based on correlation with the regional pollen stratigraphy and/or an assumption of a synchronous Younger Dryas-Preboreal transition; **10 740 + 100/−250 clay-varve years BP (Västergötland). An error of c. 875 years have been identified in the Swedish varve chronology (e.g., Andrén et al. Citation1999) which means that the calendar year age for the warming is around 11.5 ka.
The early Preboreal warming also affected the melting of the FIS, with a general increase in ice margin retreat from 50 to 75 m a−1 in the Late Younger Dryas to c. 100–200 m/year in the Early Holocene (; Strömberg Citation1994; Stroeven et al. Citation2016). A marked change in clay varve thickness, silt content in the summer layers and a colour change have all been interpreted as a response to the increased melting and rapid retreat of the ice sheet following the final drainage of the Baltic Ice Lake (Strömberg Citation1994; Brunnberg Citation1995; Andrén et al. Citation1999, Citation2002).
The deglaciation of the Baltic Sea, following the drainage of the Baltic Ice Lake, occurred during the Yoldia Sea (11.7–10.7 ka BP) and Ancylus Lake (10.7–9.8 ka BP) phases (Björck Citation1995), with build-up of large subaqueous eskers as the ice sheet retreated towards north (e.g., Lundqvist Citation1986). Due to isostatic uplift and changing outlets, many areas experienced a transition from sub-aquatic to supra-aquatic deglaciation patterns between c. 11 and 10 ka BP, with the formation of deltas built up to the Highest coastline in Central Sweden (e.g., Lundqvist Citation2003; Bernhardson & Alexanderson Citation2017). Dune fields developed on many of these deltas following the deglaciation between c. 10 500 cal a BP until c. 9000 cal a BP when the dunes were stabilised by vegetation and soil formation (e.g., Bernhardson & Alexanderson Citation2017). The location of the last ice remnant of the FIS has historically been placed either east of the water divide (e.g., Högbom Citation1892; Frödin Citation1921) or in the high mountains of e.g., Kebnekaise, Sarek and Sulitelma (e.g., Hoppe Citation1959; Lundqvist Citation1986). In their reconstruction of the deglaciation of the FIS, Stroeven et al. (Citation2016) placed the last remnant of the FIS in the eastern Sarek mountains at c. 9700 cal a BP, but an alternative model was proposed by Regnéll et al. (Citation2019) who argued that the last ice remnants of the FIS melted away east of the Scandes mountains after 10.3–9.9 ka BP, more in line with the early investigations in the late 1800 s and early 1900 s.
The early Holocene warming was interrupted by several short cold climatic events (), mainly due to weakenings of the North-Atlantic thermohaline circulation resulting from meltwater outbursts from the melting Laurentide ice sheet and/or solar events (Björck et al. Citation2001; Fisher et al. Citation2002; Teller et al. Citation2002; Rasmussen et al. Citation2007). These events are usually referred to as the Preboreal Oscillation (or the 11.4 ka BP event), the 10.3 ka BP event, the 9.3 ka BP event and the most prominent and possibly global 8.2 ka BP event (Alley et al. Citation1997). All these events are recognised in the Greenland ice-core records except for the 10.3 ka BP event (Rasmussen et al. Citation2007). The 10.3 ka BP event has potentially been linked to the drainage of the lake Agassiz McCauleyville stage that may have triggered a cooling episode across much of the Northern Hemisphere with local glacial readvances in Norway, known as the Erdalen events (e.g., Dahl et al. Citation2002), an IRD event in the North Atlantic (Bond et al. Citation1997) and terrestrial records on the Faroe Islands and Orkney, Scotland showing a cooling at this time (Björck et al. Citation2001; Timms et al. Citation2021). The Erdalen event and other Early Holocene glacial fluctuations have been described from Norway as morphological end-moraine ridges (e.g., Andersen Citation1980; Shakesby et al. Citation2020), but evidence from Sweden is mostly based on litho- and biostratigraphy in lake sediment records. It is generally assumed that all remnants of the FIS were melted before c. 9700 cal a BP and did not survive the early Holocene warming (Stroeven et al. Citation2016; Regnéll et al. Citation2019). Recent results, however, show that some glaciers in northern Norway may have survived the early Holocene and even the HTM (Bakke et al. Citation2010). Reactivation of these glaciers in Norway during the Erdalen and Finse events (10.3–10.0 and 8.5–8.0 ka BP; Nesje Citation2009) can be correlated with the 10.3 ka BP and 8.2 ka BP events, but no conclusive evidence exists in the Swedish part of the Scandes mountains for glacier advances before ca. 6000 cal a BP or a survival of glaciers during the HTM (Rosqvist et al. Citation2004; Nesje Citation2009).
There is only weak evidence for a standstill or oscillation of the ice margin in Sweden during the Preboreal oscillation, in comparison with Norway where a c. 200-year topographically controlled ice-margin standstill has been observed in many places (e.g., Andersen Citation1980). The continuation in south-western Sweden of the Norwegian Aker moraines (Sørensen Citation1979; Lundqvist Citation1988; Wastegård Citation1998), however, indicates that one or more climatic oscillations may have affected the retreat of the ice-margin in the early Preboreal at c. 11.5–11.1 ka BP (). However, it is uncertain if the moraines are climatically or topographically caused, and there are no absolute dates for the moraines, although dates of marine fossils provide minimum ages (Fredén Citation1988; Wastegård Citation1998). The ingression of marine water into the Baltic Basin during the Yoldia Sea phase coincides with the Preboreal Oscillation (Schoning Citation2001; Björck et al. Citation2002), and it has been hypothesised that a temporary slowdown of the ice margin retreat and a decreased input of meltwater may have facilitated an increased inflow of saline waters into the Baltic Basin (Björck et al. Citation1996; Andrén et al. Citation2002).
Table 2. Examples of studies reporting evidence for the Preboreal Oscillation/11.4 ka BP event in Sweden (from south to north). *An error of c. 875 years have been identified in the Swedish varve chronology (Andrén et al. Citation1999) which means that the calendar year age for the PBO is around 11 315–11 195 cal a BP; **age based on interpolation between the Younger Dryas- Preboreal boundary and the radiocarbon age of the rational limit of Corylus; ***age based on correlation with moraines in SE Norway (Sørensen Citation1979).
Some lake records from southern Sweden show that at least one, and possibly two, short cold events followed soon after the early Holocene warming (; Göransson Citation1977; Björck et al. Citation1996; Björck & Wastegård Citation1999). These oscillations were defined by small decreases in organic carbon content and a return of herb pollen species such as Artemisia and Chenopodiaceae. The Lake Madtjärn record in south-west Sweden () also shows an increase in herb and shrub pollen correlated with the Preboreal Oscillation (Björck et al. Citation1996). Here it correlates with a short-lived period of lower CO2 constructed from stomatal index data, dated to 11.35–11.2 ka BP (Rundgren & Björck Citation2003).
The Early Holocene and especially the Preboreal was also a period characterised by a dry continental climate, especially in southern Sweden, where past lake-level fluctuations show a distinct low at c. 11.1–10.2 ka BP (Digerfeldt Citation1988). Tufa formation also reflects low groundwater levels, and the most favourable conditions occurred between c. 11.5 and 8.8 ka BP in southern Sweden (Gedda Citation2006). There is, however, little or no evidence for the 10.3 ka BP and 9.3 ka BP events in Sweden. A possible climatic perturbation was described from Lake Stentjärn in Jämtland at c. 10.3 ka BP (; Bergman Citation2005), but chronological uncertainties and the proglacial setting of the site makes this uncertain. Investigations of dune fields in south-central Sweden indicate a generally unstable Early Holocene climate until c. 9.5 ka BP when groundwater levels rose and the dunes stabilised due to a denser vegetation cover (Bernhardson et al. Citation2019).
Figure 3. Sites in central and north Sweden mentioned in the text. Light grey = areas above the Baltic Sea at c. 7500 cal a BP (during the early Littorina Sea phase). The approximate position of provinces (Swe: landskap) in bold italics.
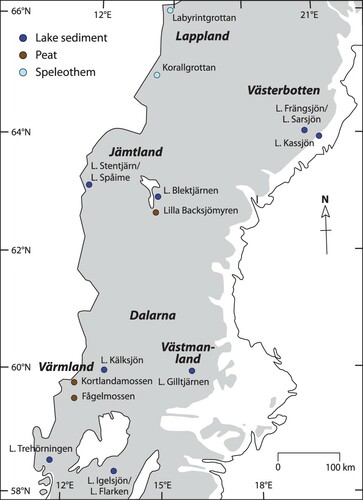
Palaeobotanical investigations from the Scandinavian mountains show that the Early Holocene was warm with summer temperatures at least 1–1.3°C higher than today at c. 9500–8000 cal a BP (Kullman Citation2002; Paus & Haugland Citation2017) and about 2°C higher until c. 7500 cal a BP in Northern Finland (Väliranta et al. Citation2015). This is in agreement with a gradually decreasing summer insolation after 11 ka cal BP (; Miller et al. Citation2010). Pollen-inferred annual mean temperatures in central and southern Sweden show a slightly different pattern with gradually increasing temperatures interrupted by the cold event at c. 8300–7900 cal a BP (Antonsson Citation2006). Studies from the Scandinavian mountains suggest that the pine-tree limit was 100–300 m above the present pine tree-limit between 9500 and 8050 cal a BP (Karlén & Kuylenstierna Citation1996). Öberg & Kullman (2012) showed that the birch treeline was nearly 600 m above the present treeline at c. 9500–8500 cal a BP.
The 8.2 ka BP event was first recognised as the Misox oscillation by the Swiss botanist Zoller (Citation1960) and is now considered “a near-global episode that is reflected in a wide range of proxy records” (Walker et al. Citation2019). The 8.2 ka BP event now defines the transition from the Early to the Middle Holocene or the Greenlandian to Northgrippian stages with a GSSP (Global Boundary Stratotype Section and Point) in the Greenland NGRIP1 ice core at 8236 y b2k or 8186 cal a BP (Walker et al. Citation2019).
The most convincing evidence in Sweden for cooling in connection with the 8.2 ka BP event comes from pollen records (). The dates vary, however, and it is difficult to determine if this is due to chronological problems or that the event was asynchronous and/or time-transgressive in Sweden. Several pollen-inferred temperature records from southern and central Sweden show a clear response to a cooling centred at c. 8200 cal a BP (8300–7900 cal a BP), with a cooling of around 1–1.5°C followed by a warming of c. 2–3°C (Seppä et al. Citation2005; Antonsson & Seppä Citation2007). A close inspection of the sites investigated by Antonsson & Seppä (Citation2007) reveals that the timing of the cooling may differ by several hundred years, which may be explained by small discrepancies in the age-depth models (e.g., Antonsson et al. Citation2006). There are also inconsistent temperature reconstructions from pollen and chironomids (Antonsson et al. Citation2006) which further complicates the interpretation of the event. A cooling has also been noted in northern Sweden but at 7950–7650 cal a BP as an increase in winter-snow accumulation and a decrease in total pollen influx (Snowball et al. Citation2002). This record, along with another varve-dated lake sediment record from southern Sweden (Snowball et al. Citation2010), show that the response to the 8.2 ka BP event may have been delayed in Sweden compared with Greenland (Rasmussen et al. Citation2006). In addition, the pollen-inferred cooling at Lake Gilltjärnen, south-central Sweden () has a similar age compared with the sites dated by varve chronology (Antonsson et al. Citation2008). It is possible that the response to the 8.2 ka BP event was asynchronous in Sweden, but a difference of several hundred years is difficult to explain and can be due to chronological issues in one or more of the records. On the other hand, a climate deterioration was found in lake records in Jämtland at 8500 cal a BP, well before the 8.2 ka BP event (Bergman et al. Citation2005). These records, along with varve-dated sites studied by Snowball et al. (Citation2002; Citation2010) are more consistent with the hypothesis of a broad climate anomaly at 8600–8000 cal a BP, encompassing the distinct cold event at 8200 cal a BP, as proposed by Rohling & Pälike (Citation2005). A stalagmite record from Jämtland (Sundqvist et al. Citation2007) also shows a more complex response to the cooling around 8.2 ka BP, with two or three cold events rather than one single “8.2 ka BP event”. A similar result, with two or three colder episodes around 8000 cal a BP has also been reported from a varved lake in Finland (Ojala et al. Citation2008).
Table 3. Examples of studies reporting evidence for cooling (or coolings) around the 8.2 ka BP event in Sweden (L. = Lake) (from south to north).
The Lake Igelsjön lake sediment sequence from south-central Sweden () shows a response to the 8.2 ka BP event in the stable oxygen record, which is correlated to a pronounced cooling of c. 1.5°C as inferred from pollen-stratigraphy in the nearby Lake Flarken (Seppä et al. Citation2005). The Lake Igelsjön record indicates a general increase in effective humidity between ca. 8300 and 7900 cal a BP as a response to the 8.2 ka BP event (Seppä et al. Citation2005).
There is some support for the 8.2 ka BP event in the Scandes mountains. Karlén (Citation1988) and Karlén & Kuylenstierna (Citation1996) suggested that a glacier advance may have occurred at 8500–7900 cal a BP, but later investigations found no convincing evidence for glacier advances before ca. 5000 cal a BP (Snowball & Sandgren Citation1996; Rosqvist et al. Citation2004). Tree-line studies, on the other hand, point to an elevational decrease of c. 150 m at 8400–8000 cal a BP (Öberg & Kullman 2012).
The Middle Holocene
The Middle Holocene stage is characterised by a generally warm and dry climate that has been referred to as the HTM (c. 8000–5000 cal a BP) or the Holocene Climate Optimum. It should be noted, however, that the duration of the HTM does not exactly correspond to the Middle Holocene stage, as defined by the GSSPs to 8236–4250 a b2k (Walker et al. Citation2019). The timing and duration of the HTM, however, was not consistent throughout the Northern Hemisphere, for example, Alaska and northwest Canada experienced the HTM between c. 11 and 9 ka BP, about 4000 year prior to the HTM in northeast Canada and Scandinavia (e.g., Kaufman et al. Citation2004).
The onset and duration of the HTM has been discussed in Scandinavia since the early 1900s CE, but in general temperature reconstructions in Fennoscandia place it between c. 8000 and 5000 cal a BP, with maximum temperatures c. 7500–6000 years ago (e.g., Seppä et al. Citation2005). Some records show a longer duration, between c. 9000 and 4000 cal a BP, interrupted by the 8.2 ka BP event. Temperature reconstructions based on bioproxies (diatoms, pollen, macrofossils) all point to summer temperatures 1.5–3°C higher than the early 1900s CE. Several records show a more or less abrupt cooling between 6000 and 5500 cal a BP (e.g., Snowball et al. Citation2004), coinciding with a change to wetter bog conditions in southern Sweden (Mallon Citation2012; Kylander et al. Citation2013).
Studies related to the HTM have a long history in Sweden, and pioneering studies were conducted by Gunnar Andersson and others in the early 1900 s CE. The dating and the nature of the HTM were the subject of an acrimonious debate between Gunnar Andersson and Rutger Sernander in the early decades of the 1900 s. Andersson, on one side, was stressing a warm period in the early to middle Holocene, followed by a gradual cooling, while Sernander was in favour of shifts between wet and dry phases, in line with the Blytt–Sernander climatostratigraphic scheme of Holocene climate variation (). A comprehensive review of the debate can be found in Birks & Seppä (Citation2010).
Gunnar Andersson can be considered as the first quantitative palaeoecologist in the Nordic countries and one of the first to use bioproxy analysis. His main focus was on taxa that indicated warmer climates in the past, and in particular fossil nuts of Corylus avellana as an indicator species for the HTM (Andersson Citation1909; see also Birks & Seppä Citation2010). Later on, von Post (Citation1924, Citation1930) in Sweden and Iversen (Citation1944) in Denmark presented conclusive evidence for the HTM based on other warm indicator taxa, such as Claudium mariscus, Trapa natans and Ilex aquifolium. The duration of the HTM was still an issue, however, and von Post first supported Sernander's idea that the HTM lasted until the Subboreal-Subatlantic transition in the Blytt–Sernander scheme at c. 2500 cal a BP (). Later on, however, he changed his opinion (von Post Citation1924; Birks & Seppä Citation2010) and concluded that the peak of the HTM took place earlier and was followed by a cooling trend, in agreement with Andersson's interpretation 15 years earlier.
Pollen and macrofossil analysis are classic methods in Sweden for reconstructing climate and environmental change during the Holocene, often in conjuction with peat-stratigraphical studies. The general pattern with a warm and dry HTM, followed by a cooling trend towards the present has been confirmed by many different methods and proxies since the pioneering studies by Gunnar Andersson, Lennart von Post, Erik Granlund and others.
Pollen-based temperature reconstructions from southern and middle Sweden point to the highest Holocene temperatures during the HTM, peaking at c. 7500–6000 cal a BP (). At this time mixed oak forests (Quercetum mixtum) dominated in southern and middle Sweden (e.g., von Post Citation1924; Bergström Citation1959). The presence of the pond turtle (Emys orbicularis) in southern Sweden also coincides approximately with the HTM (Sommer et al. Citation2009). Proxy-data from Northern Sweden suggest that the peak summer temperature was reached at c. 7100 cal a BP (Rosén et al. Citation2001) followed by a decreasing trend inferred from diatoms and pollen-based temperature reconstructions (from 6200 cal a BP). Other proxy records from the Scandes Mountains show similar patterns although with slightly different timing between the different proxies (Bigler et al. Citation2002). Palaeobotanical evidence indicates optimal conditions for pine growth between 6300 and 4500 cal a BP (Barnekow Citation2000). A recent record from L. Flocktjärn in NE Sweden () shows a chironomid-inferred HTM between 7700 and 5260 cal a BP (Engels Citation2021), and it was noted that there is a general lack of palaeoecological records from that part of Sweden.
Table 4. Examples of studies reporting evidence for the HTM in Sweden (from south to north).
Cave deposits
Cave dripstones are relatively rare in Sweden, and there are only a few available records of stable isotopes from stalagmites (Sundqvist et al. Citation2007). Warmer conditions than today are inferred between 7800 and 5900 cal a BP by two stalagmites from Korallgrottan in northern Jämtland (), but the records end at 5900 cal a BP so the exact duration of the HTM cannot be deduced from Swedish speleothem records.
Dendroclimatology
Tree rings have a potential to provide information about temperature as well as hydroclimate variation (e.g., Linderholm et al. Citation2018). The longest continuous dendrochronology from Sweden is the Torneträsk pine chronology which extends back 7400 years (; Grudd et al. Citation2002). Long-term trends, however, are usually not well preserved in tree-ring records. The Torneträsk chronology is no exception, and the HTM followed by a cooling trend is not well expressed in the tree-ring data.
Evidence for summer temperatures higher than today can be found in studies of tree-limit changes in the Scandinavian mountains. An altitudinal expansion of Alnus sp. coincides with peaks in Alnus pollen and macrofossils in lake sediments from Jämtland around 7500–7000 cal a BP, which probably reflects mild winters and summers (Bergman Citation2005). Shortly after ca. 6000 cal a BP, Alnus disappears in the area, possibly as a result of a long-term decline in summer insolation (Bergman Citation2005). In the Torneträsk area, northern Sweden, maximum altitudes of forest limits were reached between c. 6300 and 4500 cal a BP, implying a temperature 1.5–2.0° warmer than today (Barnekow Citation2000). High pine-tree limits were also recorded at 7900–5100 cal a BP (Karlén & Kuylenstierna Citation1996) and until c. 4800 cal a BP by Kullman (Citation2013).
Glaciers
Swedish mountain glaciers have been less studied compared with their Norwegian counterparts, where most mountain areas show small or absent glaciers between 9500 and 5000 cal a BP (Nesje Citation2009). The Norwegian glaciers generally reached their minimum extent between 6600 and 6000 cal a BP. Snowball & Sandgren (Citation1996) and Rosqvist et al. (Citation2004) found no conclusive evidence for glacier growth before c. 3600 cal a BP and 5000 cal a BP, respectively. Periods with soil formation between 7800–7500 and 6300–4080 cal a BP in the Kebnekaise mountains also suggest that glaciers were generally absent in Sweden during the HTM (Hormes et al. Citation2004). However, glacier advances in Sweden during the Early and Middle Holocene have been proposed by Karlén (Citation1988) and Karlén & Kuylenstierna (Citation1996), for example at 7400–7200 and 6300–6100 cal a BP, based on indirect evidence from dendroclimatology, lichenometry and lake sediments.
Glaciers started to grow again during the mid-Holocene cooling c. 6000–5000 cal a BP, as a response to a wetter and colder climate and have since been continuously present in the Scandes Mountains. Swedish glaciers respond primarily to changes in summer temperature (Holmlund et al. Citation1996) in contrast to glaciers in western Norway that are more influenced by changes in the winter precipitation (Nesje et al. Citation2001). Glacier growth is also affected by postglacial land uplift, which is believed to have been around 150 m in the mountains of northern Sweden since the early Holocene deglaciation (Karlén & Kuylenstierna Citation1996). This is an important contribution to neoglaciation and the suppression of tree-lines since c. 6000 cal a BP.
Lake sediment and lake level changes
Sweden has a rich variety of lakes and a long history of studies of lake sediment and lake levels, dating back to the early decades of the 1900s (e.g., Lundqvist Citation1924, Citation1940; von Post Citation1934). Gunnar Digerfeldt's studies of lake level changes in southern Sweden are now considered classic palaeolimnological studies (e.g., Digerfeldt Citation1988; Harrison & Digerfeldt Citation1993). Fluctuations in lake levels may indicate a change in humidity caused by temperature or precipitation, or a combination of both. Lake levels in south and central Sweden were generally low during the HTM but with some differences in timing. Lake levels in northern Sweden also reached a minimum during the HTM, due to dry and warm conditions (Barnekow Citation2000).
Most proxy-based reconstructions point to a warm and generally dry HTM in Sweden. The Lake Igelsjön () record in south-central Sweden is a typical example, and stable warm and dry conditions were reconstructed here from c. 8000 to 4300 cal a BP (Hammarlund et al. Citation2003; Seppä et al. Citation2005). This came to an abrupt end in agreement with many other climate reconstructions from Boreal Europe (Seppä et al. Citation2005).
Peat
The use of peat stratigraphy for palaeoclimate reconstructions has a long history in Sweden dating back to pioneering studies in the early 1900s by Sernander, Granlund, von Post and others. One of the first systematic studies of ombrotrophic peat records was presented by Erik Granlund in his thesis (Granlund Citation1932). Granlund investigated the stratigraphy and lateral expansion of bogs in southern Sweden and coined the term “recurrence surfaces” for sharp transitions in peat humification, assumed to represent climate shifts from dry and warm to wet and cold conditions. Today, these transitions are usually referred to as “wet shifts” (e.g., Mauquoy & Barber Citation2002). Water-table reconstructions from Holocene peatlands are increasingly being used as indicators of terrestrial palaeoclimate, and today a multi-proxy approach is often strived for, rather than using a single proxy (e.g., Mauquoy & Barber Citation2002; Vorren et al. Citation2007; Kylander et al. Citation2013).
With the exception of a few peat sites in Jämtland, northern Sweden dating back to ca 11 000–10 500 cal a BP, the vast majority of peat-based studies show that peat initiation started later in Sweden, between c. 10 000 and 5000 cal a BP (Borgmark & Wastegård Citation2008; Rundgren Citation2008; Kylander et al. Citation2013). A first peat initiation maximum is recorded in northern Sweden at 9500–8000 cal a BP. This is largely in agreement with MacDonald et al.’s (Citation2006) review of peatland initiation throughout northern Europe, although the maximum in the European dataset begins already at 10 500 cal a BP. A second, less pronounced maximum is seen in the Swedish records between 6000 and 5500 cal a BP, mainly in Värmland (; Rundgren Citation2008). Both periods in Sweden are dominated by paludification which indicates that the peat initiation maxima were primarily due to climatic causes (Rundgren Citation2008). Bergman (Citation2005) also noted an episode of increasing humidity between 5800 and 4800 cal a BP in a bog record from Jämtland. A transition from minerotrophic to ombrotrophic peat occurs in many areas around c. 6500–6000 cal a BP, and a trend to wetter and colder conditions can be seen in many records from ca. 5500 to 5000 cal a BP (Rundgren Citation2008). Peat initiation in southern Sweden started later, around 8500 cal a BP (Franzén Citation2002), possibly as a result of increased humidity after the early Holocene dry period. It should be noted, however, that the lower-lying coastal areas of southern and middle Sweden were inundated by the Baltic Sea in the East and the North Atlantic in the West during the Early Holocene ( and ), meaning that peat initiation could not start in these areas until sometime after the land emerged from the sea.
Peat records from the HTM in Sweden are usually dominated by minerotrophic fen peat, which is less suitable for palaeoclimatic reconstructions than ombrotrophic peat (e.g., Barber & Charman Citation2003). One of few published records dominated by Sphagnum peat during the HTM, Kortlandamossen in Värmland, SW Sweden (), shows consistently high humification values between c. 7500 and 5000 cal a BP (), which is in line with warm and dry conditions and low local groundwater levels (Borgmark & Wastegård Citation2008). A peat record from Skåne, Viss Mosse (; Edvardsson et al. Citation2012), shows dry bog-surface conditions at 7300–6500 cal a BP.
Figure 5. (Colour online) Examples of Swedish palaeoclimate reconstructions for the last 10 000 years. A. July insolation at 60°N. B. Pollen-based annual temperature curve from Lake Gilltjärnen (; N60.08°; E15.79°; dark green curve to the left) and Lake Trehörningen (; N58.56°; E11.61°; dark purple curve to the right) from Antonsson et al. (Citation2006) and Antonsson & Seppä (Citation2007). C. δ18Odiatom records from two lakes in northern Sweden, Vuolep Allakasjaure (; N68.17°; E18.17°; yellow curve to the left, upper axis) and Lake 850 (; N68.30°; E19.12°; black curve to the right, lower axis) from Shemesh et al. (Citation2001) and Rosqvist et al. (Citation2004). δ18Odiatom minima are interpreted as glacier advances. D. Glacier variations in northern Sweden (Nesje Citation2009 and references therein). E. Lake level records from Lake Bysjön (; N55.68°; E13.55°; orange curve, lower axis) (Digerfeldt Citation1988) and Lake Ljustjärnen (; N59.76°; E14.48°; blue curve, upper axis) (Almquist-Jacobson Citation1995), as presented in Kylander et al. (Citation2013). F. Peat humification record from Kortlandamossen, SW Sweden (; N59.85°; E12.28°; brown curve) (Borgmark & Wastegård Citation2008); brown bars to the right show peat initiation maxima in northern Sweden (9500–8000 cal a BP) and southern Sweden (6000–5500 cal a BP); blue bars show widespread periods with high bog water tables in Swedish bogs (Rundgren Citation2008).
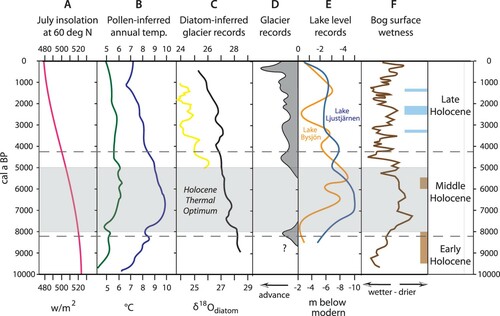
The Store Mosse peat record (), one of the best-studied bogs in southern Sweden, suggests that warm and dry conditions prevailed between 8140 and 6605 cal a BP, with the most extreme warm and dry conditions at c. 6900–6600 cal a BP (Kylander et al. Citation2013). Wetter conditions dominate at Store Mosse from 5500 to 4980 cal a BP.
The Late Holocene
A change to colder and wetter conditions is evident in many Swedish records around the Mid- to Late Holocene transition at c. 4500–3500 cal a BP. The GSSP defining the base of the Late Holocene is currently defined at 4200 cal a BP, and there were several arguments for placing the boundary at the “4.2 ka event”, including droughts in the Eastern Mediterranean, Mesopotamia, the Indus Valley and the Yangtse River Valley, which coincide with cultural shifts (Walker et al. Citation2019). This has recently been challenged by e.g., Bradley & Bakke (Citation2019) who found no compelling evidence for a significant and widespread climatic anomaly in the Northern North Atlantic region at c. 4.2 ka BP, rather that this event was one of many of a series of fluctuations superimposed on an overall decline in temperature.
Several Swedish records show a trend towards wetter and colder conditions during the Late Holocene with short-term smaller fluctuations superimposed on a general trend ( and ). In many records this trend started already with a cooling at c. 6000–5500 cal a BP and can to some extent be explained by a decreasing summer insolation in the northern Hemisphere (). Shorter fluctuations have, among others, been explained by weakening of the AMOC (Atlantic Meridional Overturning Circulation), volcanic eruptions and changes in the solar output (Mayewski et al. Citation2004; Wanner et al. Citation2008). It is, however, clear that climate variability became more regional and complex as the Holocene progressed, and comparison of records through this transition is therefore more difficult over time and space (O’Brien et al. Citation1995).
Figure 6. (Colour online) Examples of Swedish palaeoclimate reconstructions for the last 5000 years: The dashed line shows the Middle/Late Holocene boundary: A. Tann record from Lake Flarken (; N58.56°; E13.67°; Seppä et al. Citation2005). B. Carbonate δ18O record from Lake Igelsjön (; N58.55°; E13.67°; Hammarlund et al. Citation2003). C. Water table reconstruction from Lilla Backsjömyren (; N62.59°; E14.54°; Andersson & Schoning Citation2010). D. Glacier variations in northern Sweden (Nesje Citation2009 and references therein). E. Lake level records from Lake Bysjön (; N55.68°; E13.55°; orange curve, lower axis) (Digerfeldt Citation1988) and Lake Ljustjärnen (; N59.76°; E14.48°; blue curve, upper axis) (Almquist-Jacobson Citation1995), as presented in Kylander et al. (Citation2013). F. Oxygen isotope record from Lake Blektjärnen (Fig, 3; N62.98°; E14.65°; Andersson et al. Citation2010). G. Peat humification record from Fågelmossen, Värmland (; N59.53°; E12.17°; Borgmark & Wastegård Citation2008).
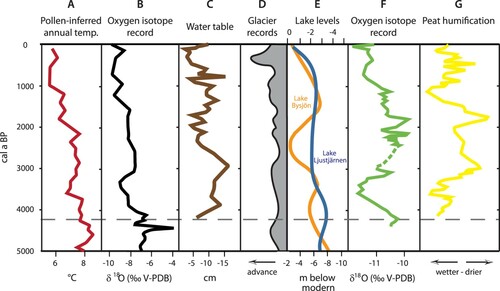
Aeolian deposits
Aeolian dune formation in Sweden was more or less completed at c. 9 ka BP some hundred years after vegetation was established, but Alexanderson & Bernhardson (Citation2016) also noted periods with sand drift in Dalarna () in connection to regional climatic changes at c. 4100 cal a BP, 1570–1410 cal a BP and c. 1045 CE (970 ± 60 cal a BP) ().
Table 5. Examples from Sweden of cold and/or wet climate events in the Late Holocene (4200 cal a BP-c. 1880 CE).
A further proxy for sand drift is Aeolian Sand Influx (ASI) in peat-bogs, investigated in Sweden by Björck & Clemmensen (Citation2004) and de Jong et al. (Citation2006, Citation2007). High ASIs were recorded at 4800, 4200, 2800–2500, 1500, 1100 and 400–50 cal a BP in bogs in Halland by de Jong et al. (Citation2006), indicating several cold and stormy periods during the Late Holocene ().
Archaeology and historical records
Archaeological records from the Bronze and Iron ages in Scandinavia (; c. 3700–900 cal a BP) have often been interpreted as reflecting climate-induced changes to the societies. The Subboreal-Subatlantic boundary at c. 2600 cal a BP in the Blytt–Sernander scheme coincides with the Bronze Age-Iron Age transition in Scandinavia () and has been interpreted as a change to more maritime conditions with colder summers and an increased humidity (e.g., van Geel et al. Citation1996). This transition is regarded as a period of crisis in the development of the cultural landscape in southern Scandinavia, due to a combination of overpopulation, overexploitation and climate change (Berglund et al. Citation1991). Rising groundwater levels caused expansion of wetlands, flooding and formation of lakes in southern Sweden. This period coincides with one of the most pronounced drops in solar insolation in the Holocene (van Geel et al. Citation1983) and, for example, led to the abandonments of settlements in the Netherlands due to raising water tables. The Subboreal-Subatlantic transition has traditionally been connected to the “Fimbul Winter” in the Nordic mythology (Sernander Citation1908). This was, however, questioned by Bergeron et al. (Citation1956), and more recently Gräslund (Citation2007) suggested that the “Fimbul Winter”, as described in Nordic Sagas, was a real event starting at 536 CE. The onset of this event, now also known as the LALIA (; Late Antique Little Ice Age; 536–660 CE, Büntgen et al. Citation2016), followed a cluster of large volcanic eruptions and a solar minimum (Büntgen et al. Citation2016; Toohey et al. Citation2016). In Sweden, this event was followed by farm and village abandonment, resulting in the greatest change in settlement patterns over the last 6500 years (Gräslund & Price Citation2012). Interestingly, human genetic bottlenecks have been suggested in Finland at 4100–3800 cal a BP and 1500–1300 cal a BP, which coincides with the earliest part of the Late Holocene and the LALIA (Sundell et al. Citation2014).
Climate information from historical sources can be traced back to the early 1400s CE in Sweden and some secondary sources date back to the late eleventh century CE (Retsö & Söderberg Citation2019). Information about both winter/spring and summer conditions can be deduced, e.g., ice break-up times in the spring and suitable conditions for winter warfare (Retsö Citation2002; Leijonhufvud et al. Citation2008) and extreme rainfall events and crop failures in summer (Retsö Citation2015). A general problem with historical sources is a bias towards extreme weather events, such as storms and rainstorms, and towards “negative” evidence, such as lack of snow in winter and harvest failures (Retsö & Leijonhufvud Citation2020). A winter/spring temperature reconstruction for the last 500 years, based on documentary sources such as harbour documents (sea tolls, harbour fees, etc.) and instrumental data (from 1756 CE), shows that the coldest winter conditions occurred in Stockholm during the Little Ice Age (LIA) at the 1500–1600s and early 1800s CE (Leijonhufvud et al. Citation2008; Citation2010). Perhaps more surprisingly, summer conditions at the “height of the Little Ice Age”, between c. 1570 and 1630 CE, were characterised by a high variation with some years being extremely wet and some years extremely warm (Retsö & Leijonhufvud Citation2020). Wet years become more uncommon from the 1660 s CE and most years were either dry or very dry, especially from the mid-1700 s onwards.
Cave deposits
A stalagmite from the cave Korallgrottan in Jämtland, NW Sweden () shows an enrichment of both carbon and oxygen isotopes and decreasing speleothem growth rates over the last 4000 years. This has been interpreted as a response to the general cooling trend (Sundqvist et al. Citation2010). Isotopic shifts at 800–1000 CE and 1300–1700 CE are interpreted as responses to the MWP and LIA, respectively.
Dendroclimatology
Tree growth in northern Scandinavia has a strong correlation to summer temperature, and tree ring-width and maximum density data from the Torneträsk area () show a negative trend of −0.3° over the last 1500 years but with a considerably warm Medieval Warm Period, ca. 900–1100 CE (c. 1050–850 cal a BP) centred around 1000 CE (Grudd Citation2008). Warm periods are also recorded around 750, 1400 and 1750 CE (1200, 550 and 200 cal a BP). A highly variable, but generally cold climate is indicated between c. 2600 and 1950 cal a BP (Grudd Citation2008). There is a generally good agreement between the Torneträsk chronology and a dendrochronology for the last 3600 year in Jämtland (), some 600 km SW of Torneträsk (Linderholm & Gunnarson Citation2005). A warm Medieval Warm Period is also well defined in Jämtland but confined to the tenth century CE (c. 1050–950 cal a BP).
Gunnarson et al. (Citation2003), Linderholm & Gunnarson (Citation2005) and Gunnarson (Citation2008) have also utilised tree-rings as a proxy of hydroclimate reconstructions. Dendrochronological records of submerged trunks have been coupled to changes in past lake levels, due to hydroclimatic changes. Several wet and dry periods have been reconstructed, some of which have been correlated with wet shifts in bogs (Gunnarson et al. Citation2003), e.g., at 4150–4050, 2050–1900 and 1150–1050 cal a BP.
A colder climate with longer periods of snow-cover is indicated during the last c. 4500–4000 years by a lowering of tree-lines in the Scandes Mountains (e.g., Kullman Citation1995; Barnekow Citation2000). A retreat of continuous forest is dated to 3700–3500 cal a BP in the catchment of Lake Spåime in Jämtland, where the regional forest-limit was suppressed by increasing net precipitation and a late-melting snow-cover (Bergman Citation2005). A second significant lowering of birch (Betula pubescens) tree lines at c. 600 cal a BP is associated with the Little Ice Age cooling (Bergman Citation2005). A significant break in the long-term Holocene tree-line regression is apparent during the last c. 50–100 years with an elevational rise in tree-lines up to 200 m and a substantial increase in tree and shrub cover is due to global warming (Kullman Citation2002; Kullman & Öberg Citation2009; Rundqvist et al. Citation2011).
Glaciers
It is generally assumed that Swedish glaciers reached their maximum Holocene positions during the Little Ice Age (Denton & Karlén Citation1973; Karlén Citation1988), but glacier advances have also been described at 4400, 3000, 2000 and after 1200 cal a BP (Rosqvist et al. Citation2004). Warmer conditions, favourable for soil formation in frontal moraines, have been dated to 2450–2000 and 1170–740 cal a BP, respectively (; Hormes et al. Citation2004). One of the best studied glaciers in Sweden is the Kårsa glacier in northern Sweden (). A multiproxy study of lake sediment in the Kårsa valley suggests a complete disappearance of the Kårsa glacier during the Early and Middle Holocene, and that the glacier started to reform at c. 3200 cal a BP (Snowball & Sandgren Citation1996). In his extensive survey of Holocene glacier advances in Scandinavia, (Karlén Citation1988) described significant glacier advances at c. 8300, 5900–5200, 3400–2900, 2200–1800 and 1500–1000 cal a BP. Many records, however, were based only on one glacier, or one glacier-fed lake, and may reflect local conditions rather than the regional climate.
Table 6. Examples of warm and/or dry climate anomalies in Sweden in the Late Holocene (4200 cal a BP-c. 1880 CE).
Lake sediment and lake level changes
Several lake sediment records that contain deposits from the Late Holocene have been investigated, with many examples from the Scandes Mountains where anthropogenic disturbances are less severe than in lakes situated at more densely populated lower latitudes (e.g., Berntsson et al. Citation2015). Lake sediment sequences have been employed for palaeoclimate reconstructions using transfer functions based on diatoms, pollen and chironomid data (e.g., Barnekow Citation2000; Rosén et al. Citation2001; Bigler et al. Citation2002).
Lake level variations in southern and middle Sweden were generally more subdued compared with the Early and Middle Holocene (). In the Lake Bysjön record (Digerfeldt Citation1988), a high-water stand is recorded between c. 2600 and 2300 cal a BP followed by a lowering that culminated between c. 1750 and 1050 cal a BP (). A similar, but more subdued trend can be seen in the Lake Ljustjärnen record from central Sweden (; Almquist-Jacobson Citation1995). Lake records in northern Sweden show a rise in levels at c. 4500 BP, indicating a cooler and more humid climate during the Late Holocene (Barnekow Citation2000). Increased variability and enhanced erosion have been observed around many alpine lakes between c. 2900 and 2600 cal a BP (Rubensdotter & Rosqvist Citation2003; Berntsson et al. Citation2015), possibly related to the climate event at c. 2650 cal a BP (van Geel et al. Citation1996).
The transition from the relatively warm Middle Holocene to a cooler and more unstable Late Holocene has been reconstructed in sediments from Lake Igeltjärn, south-central Sweden (: Jessen et al. Citation2005). The transition occurred in two steps: the first at 4450–4350 cal a BP and the second between 4000 and 3800 cal a BP (), followed by a CO2 concentration minimum between 3650 and 3500 cal a BP inferred from leaf stomatal index analysis. Proxies, such as stable isotopes, pollen analysis and organic carbon indicate a progressively cooler and/or wetter climate from 4450 to 3450 cal a BP, followed by a more stable environment between 3450 and 2750 cal a BP (Jessen et al. Citation2005). A general depletion of δ18Osed characterises the last 3000 years (), indicating successively more humid conditions due to a continuous increase in net precipitation, rising groundwater and decreasing evaporation (Hammarlund et al. Citation2003).
Pollen-inferred temperature reconstructions from lake sediments in southern and middle Sweden show a long-term temperature decrease starting at c. 4500 cal a BP ( and ; Antonsson Citation2006). Multiproxy studies from the Scandes Mountains also suggest a gradual lowering of July temperatures but without any clear evidence for the Medieval Warm Period and the Little Ice Age (e.g., Rosén et al. Citation2001; Bigler et al. Citation2002). A climatic and vegetational change is recorded in lake sediment from Jämtland at c. 3500 cal a BP (Bergman et al. Citation2005) followed by “near-modern” climatic conditions. The second episode of increased effective humidity and lower summer temperatures has an onset shortly after 2000 cal a BP (Bergman Citation2005).
Reconstructions of past changes in stable isotope composition of lake waters in northern Sweden show a rapid and synchronous response to a major change in precipitation patterns after 4200 cal a BP (Andersson et al. Citation2010; Larsson et al. Citation2012). This climate deterioration lasted until c. 3200 cal a BP and has been explained by an increase in winter precipitation due to a shorter ice and snow-free period. This was followed by a period with a drier climate between c. 2500 and 1750 cal a BP, followed by progressively more negative isotope values suggesting a wetting climate (; Andersson et al. Citation2010). A slight enrichment of Chara δ18O values between c. 500 and 100 cal a BP may indicate a drier and cooler climate during the Little Ice Age (; Andersson et al. Citation2010).
Some alpine lakes show midge-inferred increases in summer temperatures around 1000 CE (950 cal a BP) but most reconstructions are based on only a few samples (Hammarlund et al. Citation2004; Berntsson et al. Citation2014). A compilation of temperature reconstructions from 11 lakes in northern Fennoscandia and the Kola Peninsula, including one lake in northern Sweden (L. Vuoskkujavri; Bigler et al. Citation2002), shows no clear indication of a warm MWP. Instead, they suggest cooler conditions between c. 750 and 50 cal a BP in general agreement with LIA cooling (Bjune et al. Citation2009). A reduction of diatom diversity at around 350 cal a BP (c. 1600 CE) has been observed in annually laminated sediment from the northern Lake Kassjön (; Anderson et al. Citation1996) and may be associated with the LIA. Lake sediment studies from eastern and southern Finland, however, show clear anomalies during the last 700 years based on chironomid-inferred temperatures (Luoto Citation2013). Almost 2°C colder temperatures were reconstructed at c. 1700 CE compared with the present. No such detailed lake records exist from Sweden, however.
Peat
Several periods of increased wetness are inferred from Late Holocene peat records in Sweden. A compilation of 61 sites in central and northern Sweden showed that regional and more widespread periods with high bog water tables occurred at 3300, 2500–2100 and 1300 cal a BP (Rundgren Citation2008). Wet shifts were also found in the Store Mosse bog (), southern Sweden at c. 2600 and 1200 cal a BP (Svensson Citation1988) and at Undarsmosse in south-west Sweden at 2800, 2350 and 1500 cal a BP (; de Jong et al. Citation2006). These shifts are largely in agreement with the three most distinct recurrences surfaces (“RY”) described by Granlund (Citation1932) in bogs in southern Sweden (RYIV c. 3150 cal a BP; RYIII c. 2500 cal a BP and RYII c. 1600 cal a BP). Possible responses to the 4.2 ka BP event can be found in peat humification data from Värmland, where Borgmark & Wastegård (Citation2008) showed the most pronounced wetting of the Kortlandamossen bog () at c. 4500–4000 cal a BP (). A similar shift was also found at Gällseredsmossen, Halland () at 4400–4150 BP (Mallon Citation2012) and at Undarsmosse in Halland () at c. 4300 BP (de Jong et al. Citation2006). Fewer bog records have been investigated in northern Sweden compared to southern and middle Sweden, but a major change towards more ombrotrophic conditions was noted by Andersson & Schoning (Citation2010) at 2600 cal a BP () in a peat record from Lilla Backsjömyren, Jämtland (). This was followed by a further ombrotrophication during the last millennium and a change to more minerotrophic conditions at ca. 300 cal a BP, suggesting an increased inflow of groundwater from the surroundings. A recent drying over the last c. 300 years seems to have affected many European peatlands, including Sweden (de Jong et al. Citation2006; Swindles et al. Citation2019), due to climatic drying and warming, as well as human impact on peatlands. Peatlands in northernmost Sweden are today affected by permafrost that developed during the Little Ice Age (c. 600–100 cal a BP; Zuidhoff Citation2003; Sannel et al. Citation2018), but there is no evidence for permafrost inception before the Little Ice Age.
Discussion and synthesis
The early part of the Holocene
The Holocene climate evolution in Sweden follows a pattern in common for many northern latitude records with a rapid warming starting at the Pleistocene–Holocene boundary at c. 11 650 cal a BP, followed by the HTM between c. 8000 and 5000 cal a BP. The early Holocene warming triggered a marked increase in the ice recession rate of the FIS (e.g., Andrén et al. Citation1999) and an abrupt decrease of cold-adapted plants in southern Sweden (Schenk et al. Citation2020). It has often been assumed that climate oscillations during the Late Glacial-Interglacial transition (ca. 15–10 ka BP) were broadly synchronous (Björck et al. Citation1996), but recent results from NW Europe suggest that some of these events were asynchronous, such as the inception and the duration of the Younger Dryas cold reversal and the mid-Younger Dryas climate amelioration (Lane et al. Citation2013; Muschitiello & Wohlfarth Citation2015; Wohlfarth et al. Citation2017). A further problem when comparing the timing of the early Holocene warming is that different methods have been used for dating the deglaciation, e.g., clay-varves in the Baltic Sea, TCN dating of moraines and radiocarbon dating of sediments and macrofossils (e.g., Lundqvist & Wohlfarth Citation2000; Stroeven et al. Citation2016) and that the results often are difficult to compare. Efforts to synchronise clay-varve-based timescales with radiocarbon-dated records, by using terrestrial macrofossils in varves, have been successful to some extent (Wohlfarth et al. Citation1998; Björck et al. Citation2001) but have often proven difficult due to a scarcity of macrofossils in the glacial varves and wide error margins of radiocarbon dates performed in the 1980s and 1990s. A further problem with radiocarbon-based chronologies is the radiocarbon plateaux centred at ca. 10 000, 9600 and 8900 14C years BP, which makes exact dating of climate fluctuations during the Early Holocene challenging (e.g., Björck et al. Citation1996). A potential method for synchronising records from the last deglaciation is tephrochronology. There are promising results for Younger Dryas varves from south-east Sweden using the Vedde Ash as a time-synchronous marker (MacLeod et al. Citation2014; Muschitiello et al. Citation2016), and there are several widespread tephras from the early Holocene that have the potential for constraining the rapid warming transition at the beginning of the Holocene and the Preboreal Oscillation, e.g., the Hässeldalen, Askja-S and Fosen tephras (e.g., Davies et al. Citation2012; Lind et al. Citation2013).
The Northern Hemisphere summer insolation peaked at ca. 11 ka, followed by a steady decrease to the current level of 9% less than the early Holocene maximum (; Berger & Loutre Citation1991). Proxy-based reconstructions from the Northern Hemisphere show, however, that the timing and magnitude of the warming differ between regions, suggesting that the early Holocene climate was highly sensitive to small changes in ice-sheet configuration (Kaufman et al. Citation2004; Renssen et al. Citation2009; Briner et al. Citation2016). Sweden is no exception, and most proxy-based reconstructions show that the highest temperatures were reached here some 3000–4000 years into the Holocene (; e.g., Seppä et al. Citation2005).
Several cold reversals punctuated the early Holocene warming, but only weak or no evidence exists for the Preboreal Oscillation/11.4 ka and the 10.3 and 9.3 ka events in Sweden. This is in contrast to Norway, where Preboreal moraines occur in multiple locations at both low and high elevations (e.g., Andersen Citation1980), and to Finland where the youngest of the distinct Salpausselkä moraines, Salpausselkä III has been correlated with the Preboreal Oscillation (Björck et al. Citation1997; Stroeven et al. Citation2016) and a further ice-marginal formation, the Central Finland ice-marginal formation has been dated around 11 ka cal BP (Rainio et al. Citation1995). Moraines dated to the early Preboreal in Värmland, SW Sweden (Lundqvist Citation1988) may be an indication of a temporary slow-down of the ice retreat, but an alternative explanation is that the moraines are topographically induced and were deposited when the ice retreated from a sub-aquatic to supra-aquatic environment. Ice recessional lines north of the Younger Dryas moraines in Sweden indicate a rather rapid and uninterrupted deglaciation until the last ice remnants were melted at c. 10 ka BP (Stroeven et al. Citation2016; Regnéll et al. Citation2019). There are, however, conflicting ages for the deglaciation chronology of northern Sweden, where some results point to a deglaciation that is earlier by some 500 years (Lindén et al. Citation2006; Möller et al. Citation2013) than the deglaciation chronology of Stroeven et al. (Citation2016).
The last and most prominent cold reversal before the HTM, the 8.2 ka BP event, is more clearly registered in Swedish records compared with other events during the Early Holocene, despite no conclusive evidence in Sweden of glacier advances similar to the Norwegian Finse event (c. 8.5–8.0 ka BP; Nesje et al. Citation2001; Nesje & Dahl Citation2001). However, radiocarbon and varve ages encompassing the event differ by several hundred years, which may reflect dating difficulties, or that the responses in Sweden are more consistent with the hypothesis of a broad climate anomaly at 8600–8000 cal a BP rather than one single event (e.g., Rohling & Pälike Citation2005). Many pollen-based records, however, and especially the Lake Flarken record (Seppä et al. Citation2005), show a sudden and distinct cooling of 1.5°C at 8300 cal a BP that is correlated to a pronounced decrease in δ18O at the nearby Lake Igelsjön (). Corylus, and to a lesser extent Ulmus and Alnus, show a decline simultaneously with an increase in Betula pollen.
The Holocene Thermal Maximum
Most investigations show a clear HTM in Sweden with warm and generally dry conditions between c. 8000 and 5000 cal a BP (), some 3000–6000 years after the peak in Northern Hemisphere insolation. The warmest and driest part of the HTM is reconstructed at c. 7500–6000 cal a BP in many records, which agrees with several other European records (e.g., Davis et al. Citation2003; Heikkilä & Seppä Citation2003). In contrast, scattered finds of tree megafossils on high elevations in the Scandes Mountains, dated to c. 9500–9100 cal a BP, suggest that maximum summer temperatures were reached already in the Early Holocene (Kullman Citation1999). Summer temperatures peaking in the Early Holocene have also been reconstructed from lake sites in northern Fennoscandia, including the Lake Loitsana sediments in northern Finland (Shala et al. Citation2017). Here, aquatic/wetland macrofossil assemblages indicate higher than present summer temperatures at the peak of summer insolation at ca. 11–10.5 ka BP, while peak summer temperatures reconstructed from chironomids and pollen are dated to ca. 10 ka BP and 7000 cal a BP, respectively. The absence of an early Holocene temperature peak in southern Sweden has not been extensively discussed in the literature (cf. Paus Citation2013) and most temperature reconstructions in southern Sweden are based on pollen records, which often show a delayed response of the terrestrial ecosystem compared with aquatic ecosystems. Lake records in south Sweden also infer more maritime conditions with cool and moist conditions after the distinct low lake level stands at c. 11.1–10.2 ka BP (Digerfeldt Citation1988; Seppä et al. Citation2005). The presence of warmth-demanding plants Hedera and Viscum in southern Norway already in the Early Holocene (c. 10 ka BP), however, suggests that the spread of deciduous trees like Tilia was delayed by reasons other than unfavourable July temperatures.
A middle Holocene cold event
A transition to colder and wetter conditions is seen in many Swedish records at c. 6000–5500 cal a BP, while other records were less affected and show warm and stable conditions until c. 4200 cal a BP (e.g., Hammarlund et al. Citation2003; St. Amour et al. Citation2010). Scandinavian glaciers started to advance (e.g., Nesje Citation2009), and many southern Swedish ombrotrophic peat records show the first distinct Holocene wet shift (Sandegren Citation1937; Mallon Citation2012; Kylander et al. Citation2013). The cooling coincides with an ice-rafting episode in the North Atlantic (c. 5900 cal BP; Bond et al. Citation1997) and a significant cold period in Greenland, evidenced by increased sea salt and terrestrial dust concentrations in the GISP2 record (c. 6000–5000 cal a BP; O’Brien et al. Citation1995). This middle Holocene climate event is thought to be the result of a combination of different factors including orbital forcing, changes in ocean circulation, and variations in solar activity (Magny & Haas Citation2004). The event follows after a summer temperature maximum over northern Europe (Davis et al. Citation2003) and a peak in hunter-gatherer population in Finland (Tallavaara & Seppä Citation2012), which coincides with a peak in lacustrine and Baltic Sea productivity. Pollen records from northern Europe show that the last 5000 years have been characterised by a roughly linear cooling trend and that proxies reflecting temperature and humidity show a remarkable consistency (Seppä et al. Citation2009).
The 4.2 ka BP event
The recent formalisation of the 4.2 ka BP event as the Mid- to Late Holocene boundary (Walker et al. Citation2012, Citation2019) has sparked new interest in this climate event, also recognised as one of the Holocene IRD events in the North Atlantic (Bond et al. Citation1997). The GSSP for the Meghalayan stage at 4250 cal a b2k or 4200 cal a BP is located in a speleothem from the Mawmluh cave, Meghalaya, northeast India (Walker et al. Citation2018). Bradley & Bakke (Citation2019) found no compelling evidence for a significant and widespread climatic anomaly in northern Europe at c. 4.2 ka BP and argued that this event was one of many in a series of fluctuations superimposed on an overall decline in temperature. However, in a comment to Bradley and Bakke's paper, Weiss (Citation2019) presented convincing evidence for the 4.2 ka BP event in the northern North Atlantic region, including several Swedish bog and lake records (e.g., Hammarlund et al. Citation2003; Antonsson & Seppä Citation2007; Gunnarson Citation2008). Several bog records in southern and western Sweden display a wet shift around 4200 cal a BP (Granlund Citation1932; de Jong et al. Citation2006; Borgmark & Wastegård Citation2008; Mallon Citation2012) in agreement with many other European bog records at this time (e.g., Hughes et al. Citation2000; Langdon & Barber Citation2005; Vorren et al. Citation2007). Others, however, found no compelling evidence to support high bog water-tables during the 4.2 ka BP event (Roland et al. Citation2014). High humidity and high lake water levels are also inferred from dendroclimatology records at this time in Central Sweden (Gunnarson et al. Citation2003), and a glacier advance is reconstructed at ca. 4400 cal a BP (Rosqvist et al. Citation2004). All these records, along with many others, point to the onset of a significantly more variable climate between c. 4500 and 4000 cal a BP, possibly in connection with the 4.2 ka event (). This time period also coincides with the European Storm period II (4500–3950 cal a BP; Sorrel et al. Citation2012), and peat and aeolian records from southern and central Sweden suggest increased storminess at this time (de Jong et al. Citation2006; Alexanderson & Bernhardson Citation2016). Although it is difficult to manifest the significance of the 4.2 ka event in Sweden, many climate proxies infer a change to wetter and/or cooler climate at this time. Whether this climate anomaly was more prominent than other Late Holocene events remains an open question.
Climate events during the latter part of the Holocene
In addition to the 4.2 ka BP event which in part is the basis for defining the base of the Late Holocene (), there are several other climate events during the Late Holocene. Pollen records from northern Europe show a continuous cooling trend with cold anomalies identified at 3800–3000 cal a BP and at 500–100 cal a BP (Seppä et al. Citation2009). This trend can to some extent be explained by a decreasing summer insolation in the northern Hemisphere with shorter fluctuations due to weakening of the AMOC, volcanic eruptions and changes in the solar output (Mayewski et al. Citation2004; Wanner et al. Citation2008). A climate anomaly is demonstrated in many Swedish records between ca. 3700 and 3200 cal a BP (). Snowball et al. (Citation1999) noted a sudden increase in catchment erosion in the Lake Sarsjön record in northern Sweden at c. 3700 cal a BP, and many other records show a rapid transition to a more variable climate at this time, with glacier expansions in the mountains, wet shifts in bogs and a lowering of treelines in the mountains (e.g., Snowball & Sandgren Citation1996; Bergman Citation2005; Borgmark & Wastegård Citation2008). Several other records from northern Europe show a cold and wet period ca. 3800–3000 cal a BP, peaking at 3500 cal a BP (Seppä et al. Citation2009). Pronounced shifts to wet conditions are indicated in many European bogs at c. 3800–3400 cal a BP, indicating that this was a regional event, possibly triggered by changes in solar variability (Anderson et al. Citation1998; Blaauw et al. Citation2004; Charman et al. Citation2006). Lake isotope records from southern Sweden and the Scandes mountains show a change to more winter precipitation and cooler and more humid summers from c. 4200 cal a BP, peaking at c. 3500 cal a BP, due to a significant and persistent change in atmospheric circulation (e.g., Larsson et al. Citation2012). Thus, the “3.5 ka BP event” in Sweden may be as prominent as the 4.2 ka BP event or even more pronounced.
This period coincides with the early part of the Scandinavian Bronze age (; c. 3800–2500 cal a BP) which was a climatically complex period with cold and wet conditions before 3000 cal a BP, followed by a warm/dry phase around c. 3000 cal a BP, and finally a change to cold and wet conditions again around 2800 cal a BP (Berglund Citation2003). Stable climate conditions are inferred from the isotope record from Lake Igelsjön, southern Sweden at c. 3450–2750 cal a BP (; Hammarlund et al. Citation2003), and several other records show that the period around 3000 cal a BP was relatively warm and dry in Sweden (; Antonsson et al. Citation2006; Borgmark & Wastegård Citation2008; Andersson et al. Citation2010). Glacier advances in the mountains around 3200–2800 cal a BP (Snowball et al. Citation1999; Rosqvist et al. Citation2004) may to some extent be due to a delayed response to the “3.5 ka BP event”.
The possibly most prominent Late Holocene climatic event has been inferred at c. 2800–2600 cal a BP (e.g., van Geel et al. Citation1996). This event is sometimes referred to as the “2.8 ka BP event”, and many bogs in North-west Europe show a change from dark, highly humified peat to light poorly humified peat at this time in parallel with changes in Sphagnum assemblages, indicating a transition from a relatively continental to a more oceanic climate regime (e.g., van Geel et al. Citation1996). This change is known as the Subboreal-Subatlantic boundary in the Blytt–Sernander scheme () and coincides with the Bronze Age-Iron Age transition in Scandinavia and Granlund's third and possibly most conspicuous recurrence surface in Swedish bogs, RYIII. A worldwide climate event around c. 2800 cal a BP is well established in the literature and has been interpreted as a response to an abrupt decrease in solar activity around 2900–2800 cal a BP (van Geel et al. Citation2000). Also, a period of maximum IRD influx occurred in the North Atlantic at this time (Bond et al. Citation1997). Many Swedish bog records show a wet shift at this time (Granlund Citation1932; Svensson Citation1988; de Jong et al. Citation2006; Andersson & Schoning Citation2010; Mellström et al. Citation2015), and a wetter climate is also inferred from increasing lake-levels and groundwater levels in southern Sweden (; Digerfeldt Citation1988; Berglund et al. Citation1991). According to Widgren (Citation2012), however, this event had no clear effect on societies and settlements in Sweden, unlike in the Netherlands where inundation of coastal settlements peaked at 2.8 ka BP, due to a combination of a global sea-level rise and the 2.8 ka BP event (e.g., van Geel et al. Citation2020). Lake records in northern Sweden show increased variability and erosion due to excessive precipitation events (Berntsson et al. Citation2015). Temperature-inferred records, however, show rather stable temperatures between c. 3000 and 2500 cal a BP (; e.g., Antonsson Citation2006), so it is probable that the event caused an increased humidity in Sweden, rather than a cooling of the climate.
The “2.8 ka BP” event was followed by more stable and relatively dry conditions in Scandinavia until c. 1500 cal a BP (1700-1300 cal a BP) when many records show a more or less abrupt shift to wetter and colder conditions (; ). Several other Northern Hemisphere records indicate cold conditions between c. 1550 and 1135 cal a BP (400–815 CE), often under the term “Dark Ages Cold Period” (DACP; e.g., Helama et al. Citation2017). This event also stands out as an IRD event in the North Atlantic (c. 1400 cal a BP; Bond et al. Citation1997). There is, however, no evidence for a synchronous multi-centennial cold event in the North Atlantic region, which makes the structure of the DACP similar to the Little Ice Age (Helama et al. Citation2017). A cold period, partly overlapping within DACP was recently invoked, the so-called Late Antique Little Ice Age (“LALIA”; Büntgen et al. Citation2016; 536–660 CE; c. 1414–1290 cal a BP). It was suggested that this cold event, triggered by three large volcanic eruptions and sustained by a solar minimum and feedback effects, caused societal reorganisations in Europe and Asia. Archaeological records from Sweden suggest land and settlement desertion at this time with unprecedented farm abandonments (Widgren Citation2012). The LALIA event overlaps with the DACP, and a recent review indicates that the coldest conditions during the DACP (509–865 CE; c. 1441–1085 cal a BP) partly overlaps with the LALIA event (Helama et al. Citation2017). It can be questioned, however, why the LALIA event was proposed, since the concept of the Dark Ages Cold Period has been established at least since the 1980s (Lamb Citation1977, Citation1982).
Some of the most detailed and best-dated palaeoclimate Late Holocene records from Sweden are more in line with a longer DACP than a shorter LALIA event. Some changes appear to start before LALIA, e.g., wet shifts in peat records from Värmland and Halland (1800-1500 cal a BP; e.g., de Jong et al. Citation2006; Borgmark & Wastegård Citation2008), sand drift events in Dalarna (1570–1410 cal a BP; Alexanderson & Bernhardson Citation2016) and lake oxygen isotopes from Jämtland (c. 1800 cal a BP; Andersson et al. Citation2010). Other changes occur after LALIA, such as glacier advances in the Scandes Mountains (1200 cal a BP; Rosqvist et al. Citation2004), increased lake levels in Jämtland (1150–1050 cal a BP; Gunnarson Citation2008), and wet shifts in the Store Mosse peat bog (1200 cal a BP; Svensson Citation1988) (). A delayed response, however, can be explained by e.g., thresholds in the climate system and response times of glaciers.
One of the first to describe a climate event in the first millennium CE was Granlund, who found distinct wet shifts in several southern Swedish bogs around c. 1600 cal a BP (RYII; Granlund Citation1932). His observations were later confirmed by several peat-based studies in Sweden (de Jong et al. Citation2006; Borgmark & Wastegård Citation2008; Rundgren Citation2008) but with dates ranging from c. 1700 to 1300 cal a BP, suggesting a more complex picture than Granlund's original concept of regionally synchronous wet shifts. Granlund dated the recurrence surfaces by means of pollen analysis and archaeological correlation, and many dates were probably imprecise and overgeneralised. Also bogs on the British Isles show evidence for increased surface wetness at c. 1400 cal a BP (Blackford & Chambers Citation1991).
Several tree-ring records from the northern Hemisphere show narrow tree-rings at the inception of the LALIA in the 530–540 s CE (e.g., Baillie & Munro Citation1988; Büntgen et al. Citation2016), but it does not stand out as an extremely cold period in Swedish tree-ring records from Torneträsk and Jämtland (Linderholm & Gunnarson Citation2005; Grudd Citation2008). The coldest conditions during the DACP are reconstructed in the Torneträsk area around 650 CE (1300 cal a BP; Grudd Citation2008). Clearly, there is a need for more detailed analyses of climate variability during the first millennium CE (Helama et al. Citation2017) and how the DACP and LALIA affected the climate and Late Iron Age communities in Sweden.
The Medieval Warm Period (MWP; or Medieval Climate Anomaly) and the Little Ice Age (LIA) are well-known concepts around the North Atlantic region where several records and proxies show a distinct warm period (MWP; c. 1150-650 cal a BP; c. 800–1300 CE;) followed by colder conditions (LIA; c. 650–50 cal a BP c. 1300–1900 CE). The most detailed and best-resolved record of the last millennium's climate in Sweden is probably the tree-ring record from Torneträsk, northern Sweden (). This high-latitude record shows a clear MWP around 1000 CE (c. 900–1100 CE; 1050–850 cal a BP), followed by a cooling trend until c. 1900 CE (Grudd Citation2008).
Other proxy-based reconstructions from the Northern Hemisphere show a temperature variability on centennial timescales exceeding 0.6°C over the last 1000 years (e.g., Moberg et al. Citation2005; Ljungqvist Citation2009). Marcott et al. (Citation2013) showed that the cooling in the Northern Hemisphere that started at c. 5000 cal a BP culminated in the coolest temperatures during the LIA some 200 years ago. Proxy records from Sweden largely confirm this pattern, with a cold and/or wet DACP followed by a warmer and drier MWP, and a cold but variable LIA (e.g., Grudd Citation2008). Proxy-based records often have a poor resolution to fully capture climate variability on centennial time-scales, however. This concerns many pollen and chironomid-inferred temperature records and speleothem isotope records. Several Swedish glaciers advanced during the LIA to their maximum Holocene positions, but their positions during the DACP and MWP are not well known, and it is possible that Swedish glaciers were contracted during the MWP similar to many Norwegian glaciers (Nesje et al. Citation2008).
Historical records suggest that the LIA in Sweden can be divided into three parts, with the coldest winter conditions at 1500–1600s and the early 1800s CE, and warmer conditions in-between (Leijonhufvud et al. Citation2008; Citation2010). They also suggest that the LIA was characterised by highly variable climate conditions (Retsö & Leijonhufvud Citation2020).
Conclusions
More than 125 years of research have produced a wealth of information on Holocene climate variability in Sweden. The main features of Holocene climate variation were already known in the early part of the 1900s, but later investigations have provided more detailed information on the timing and magnitude of climate events. The Blytt–Sernander scheme, originally developed in the early 1900s by Sernander (Citation1908), building on Blytt's studies of Danish peat bogs (Blytt Citation1876), is still in use but should be avoided in favour of the new formalised tripartite subdivision of the Holocene (Walker et al. Citation2019). The last decades have seen development of many new proxies and methods as well as new applications of traditional methods. Multi-proxy analyses are becoming increasingly common in palaeoclimatology and many recent examples from Sweden show that strengths and weaknesses in palaeoclimate reconstructions can be identified and assessed. The present review, however, shows that several research questions remain unresolved and need to be addressed in the future:
Was the early Holocene climate warming synchronous over Sweden (and with NW Europe)?
Is there evidence in Sweden for other early Holocene events than the PBO/11.4 and 8.2 ka BP events?
How reliable is the varve-based deglaciation chronology for Sweden (and where are the “missing varves”)?
When and where did the last remnants of the Scandinavian ice-sheet melt?
What characterises the 8.2 ka BP event in Sweden?
When did Swedish glaciers start to reform in the Middle Holocene (or earlier?)
Why was the HTM in southern Sweden delayed in relation to the summer insolation maximum?
When did the HTM come to an end in Sweden, c. 5500 or 4200 cal a BP?
Was the 4.2 ka BP event significant in Sweden?
Is there a link between Holocene climate events and human population bottlenecks in Fennoscandia?
Which late-Holocene event was most prominent and had the strongest effect on human societies in Sweden?
When did permafrost inception in northern peatlands start in the Late Holocene, before LIA or at the inception of LIA?
Acknowledgements
I am grateful to Freya Sykes who made a thorough language review of a previous version of the manuscript. I also thank Antti Ojala and one anonymous reviewer whose comments and suggestions helped to improve and clarify the paper. The paper is a contribution to the Bolin Centre for Climate Research. I dedicate the paper to my PhD supervisor Professor Jan Lundqvist who has been a continuous source of wisdom and knowledge since I first met him 40 years ago.
Disclosure statement
No potential conflict of interest was reported by the author(s).
Notes
1 GS-1 (Greeland Stadial-1) refers to the most recent cold event in the Greenland ice-cores, broadly equivalent to the Younger Dryas stadial- It was originally suggested by the INTIMATE group for the isotopically-defined equivalent of the Younger Dryas cold event in the Greenland ice-cores (Björck et al., 1998; Walker et al., 1999).
References
- Alexanderson, H. & Bernhardson, M., 2016: OSL dating and luminescence characteristics of aeolian deposits and their source material in Dalarna, central Sweden. Boreas 45, 876–893. https://doi.org/10.1111/bor.12197.
- Alley, R.B., Mayewski, P.A., Sowers, T., Stuiver, M., Taylor, K.C. & Clark, P.U., 1997: Holocene climatic instability: a prominent, widespread event 8200 yr ago. Geology 25, 483–486. https://doi.org/10.1130/0091-7613(1997)025<0483:HCIAPW>2.3.CO;2.
- Almquist-Jacobson, H., 1995: Lake-level fluctuations at Ljustjärnen, central Sweden and their implications for the Holocene climate of Scandinavia. Palaeogeography, Palaeoclimatology, Palaeoecology 118, 269–290. https://doi.org/10.1016/0031-0182(95)00002-2.
- Andersen, B.G., 1980: The deglaciation of Norway after 10,000 B.P. Boreas 9, 211–216. https://doi.org/10.1111/j.1502-3885.1980.tb00697.x.
- Anderson, D.E., Binney, H.A. & Smith, M.A., 1998: Evidence for abrupt climatic change in northern Scotland between 3900 and 3500 calendar years BP. The Holocene 8, 97–103. https://doi.org/10.1191/095968398669507604.
- Anderson, N.J., Odgaard, B.V., Segerström, U. & Renberg, I., 1996: Climate-lake interactions recorded in varved sediments from a Swedish boreal forest lake. Global Change Biology 2, 399–403. https://doi.org/10.1111/j.1365-2486.1996.tb00090.x.
- Andersson, G., 1909: The climate of Sweden in the late-Quaternary period. Sveriges Geologiska Undersökning C3, 1–87.
- Andersson, L., Rafstedt, T. & von Sydow, U., 1983: Vegetationskarta över de svenska fjällen – Kartblad nr 1 Treriksröset/Naimakka. Scale 1:100 000. Meddelande från Naturgeografiska Institutionen, Stockholms universitet.
- Andersson, S., Rosqvist, G., Leng, M.J., Wastegård, S. & Blaauw, M., 2010: Late Holocene climate change in central Sweden inferred from lacustrine stable isotope data. Journal of Quaternary Science 25, 1305–1316. https://doi.org/10.1002/jqs.1415.
- Andersson, S. & Schoning, K., 2010: Surface wetness and mire development during the late Holocene in central Sweden. Boreas 39, 749–760. https://doi.org/10.1111/j.1502-3885.2010.00157.x.
- Andrén, T., Björck, J. & Johnsen, S., 1999: Correlation of Swedish glacial varves with the Greenland (GRIP) oxygen isotope record. Journal of Quaternary Science 14, 361–371. https://doi.org/10.1002/(SICI)1099-1417(199907)14:4<361::AID-JQS446>3.0.CO;2-R.
- Andrén, T., Björck, S., Andrén, E., Conley, D., Zillén, L. & Anjar, J., 2011: The development of the Baltic Sea Basin during the last 130 ka. In Harff J., Björck S. & Hoth P. (eds.): The Baltic Sea Basin. Central and Eastern European Development Studies (CEEDES), 75–97. Springer, Berlin, Heidelberg.
- Andrén, T., Lindeberg, G. & Andrén, E., 2002: Evidence of the final drainage of the Baltic Ice Lake and the brackish phase of the Yoldia Sea in glacial varves from the Baltic Sea. Boreas 31, 226–238. https://doi.org/10.1111/j.1502-3885.2002.tb01069.x.
- Antonsson, K., 2006: Holocene climate in Central and Southern Sweden: quantitative reconstructions from Fossil Data. PhD Thesis. Digital Comprehensive Summaries of Uppsala Dissertations from the Faculty of Science and Technology 168. 37 pp.
- Antonsson, K., Brooks, S.J., Seppä, H., Telford, R.J. & Birks, H.J.B., 2006: Quantitative palaeotemperature records inferred from fossil pollen and chironomid assemblages from Lake Gilltjärnen, northern central Sweden. Journal of Quaternary Science 21, 831–841. https://doi.org/10.1002/jqs.1004.
- Antonsson, K., Chen, D. & Seppä, H., 2008: Anticyclonic atmospheric circulation as an analogue for the warm and dry mid-Holocene summer climate in central Scandinavia. Climate of the Past Discussions 4, 585–610. https://doi.org/10.5194/cpd-4-585-2008.
- Antonsson, K. & Seppä, H., 2007: Holocene temperatures in Bohuslän, southwest Sweden: a quantitative reconstruction from fossil pollen data. Boreas 36, 400–410. https://doi.org/10.1080/03009480701317421.
- Baillie, M.G.L. & Munro, M.A.R., 1988: Irish tree rings, Santorini and volcanic dust veils. Nature 332, 344–346. https://doi.org/10.1038/332344a0.
- Bakke, J., Dahl, S.O., Paasche, Ø, Riis Simonsen, J., Kvisvik, B., Bakke, K. & Nesje, A., 2010: A complete record of Holocene glacier variability at Austre Okstindbreen, northern Norway: an integrated approach. Quaternary Science Reviews 29, 1246–1262. https://doi.org/10.1016/j.quascirev.2010.02.012.
- Barber, K.E. & Charman, D.J., 2003: Holocene palaeoclimate records from peatlands. In Mackay A.W., Battarbee R.W., Birks H.J.B. & Oldfield F (eds.): Global Change in the Holocene, 210–226. Edward Arnold, London.
- Barnekow, L., 2000: Holocene regional and local vegetation history and lake-level changes in the Torneträsk area, northern Sweden. Journal of Paleolimnology 23, 399–420. https://doi.org/10.1023/A:1008171418429.
- Berger, A. & Loutre, M.F., 1991: Insolation values for the climate of the last 10 million years. Quaternary Science Reviews 10, 297–317. https://doi.org/10.1016/0277-3791(91)90033-Q.
- Bergeron, T., Fries, M., Moberg, C.-A. & Ström, F., 1956: Fimbulvinter. Fornvännen 51, 1–18.
- Berglund, B.E., 2003: Human impact and climate changes – synchronous events and a causal link? Quaternary International 105, 7–12. https://doi.org/10.1016/s1040-6182(02)00144-1.
- Berglund, B.E., 1979: The deglaciation of southern Sweden 13,500-10,000 B.P. Boreas 8, 89–117. https://doi.org/10.1111/j.1502-3885.1979.tb00789.x.
- Berglund, B.E., Tesch, S. & Olausson, D., 1991: The Late Bronze Age Landscape. Ecological Bulletins 41, 73–77.
- Bergman, J., 2005: Tree-limit ecotonal response to Holocene climate change in the Scandes Mountains. PhD Thesis. Lund University, Department of Geology, Quaternary Sciences, LUNDQUA Thesis 53, 35 pp.
- Bergman, J., Hammarlund, D., Hannon, G., Barnekow, L. & Wohlfarth, B., 2005: Deglacial vegetation succession and Holocene tree-limit dynamics in the Scandes Mountains, west-central Sweden: stratigraphic data compared to megafossil evidence. Review of Palaeobotany and Palynology 134, 129–151. https://doi.org/10.1016/j.revpalbo.2004.12.005.
- Bergström, R., 1959: Postglacial skogsutveckling i Närke. Geologiska Föreningens i Stockholm Förhandlingar 81, 588–602. https://doi.org/10.1080/11035895909449174.
- Bernhardson, M. & Alexanderson, H., 2017: Early Holocene dune field development in Dalarna, central Sweden: a geomorphological and geophysical case study. Earth Surface Processes and Landforms 42, 1847–1859. https://doi.org/10.1002/esp.4141.
- Bernhardson, M., Alexanderson, H., Björck, S. & Adolphi, F., 2019: Sand drift events and surface winds in south-central Sweden: from the deglaciation to the present. Quaternary Science Reviews 209, 13–22. https://doi.org/10.1016/j.quascirev.2019.01.017.
- Berntsson, A., Jansson, K.N., Kylander, M.E., De Vleeschouwer, F. & Bertrand, S., 2015: Late Holocene high precipitation events recorded in lake sediments and catchment geomorphology, Lake Vuoksjávrátje, NW Sweden. Boreas 44, 676–692. https://doi.org/10.1111/bor.12127.
- Berntsson, A., Rosqvist, G.C. & Velle, G., 2014: Late-Holocene temperature and precipitation changes in Vindelfjällen, mid-western Swedish Lapland, inferred from chironomid and geochemical data. The Holocene 24, 78–92. https://doi.org/10.1177/0959683613512167.
- Bigler, C., Larocque, I., Peglar, S.M., Birks, H.J.B. & Hall, R.I., 2002: Quantitative multiproxy assessment of long-term patterns of Holocene environmental change from a small lake near Abisko, northern Sweden. The Holocene 12, 481–496. https://doi.org/10.1191/0959683602hl559rp.
- Birks, H.J.B. & Seppä, H., 2010: Late-Quaternary palaeoclimatic research in Fennoscandia – A historical review. Boreas 39, 655–673. https://doi.org/10.1111/j.1502-3885.2010.00160.x.
- Björck, J., Andrén, T., Wastegård, S., Possnert, G. & Schoning, K., 2002: An event stratigraphy for the last glacial-Holocene transition in eastern middle Sweden: results from investigations of varved clay and terrestrial sequences. Quaternary Science Reviews 21, 1489–1501. https://doi.org/10.1016/S0277-3791(01)00115-9.
- Björck, J., Possnert, G. & Schoning, K., 2001: Early Holocene deglaciation chronology in Västergötland and Närke, southern Sweden – biostratigraphy, clay varve, 14C and calendar year chronology. Quaternary Science Reviews 20, 1309–1326. https://doi.org/10.1016/S0277-3791(00)00168-2.
- Björck, J. & Wastegård, S., 1999: Climate oscillations and tephrochronology in eastern middle Sweden during the last glacial-interglacial transition. Journal of Quaternary Science 14, 399–410. https://doi.org/10.1002/(SICI)1099-1417(199908)14:5<399::AID-JQS448>3.0.CO;2-R.
- Björck, S., 1995: A review of the history of the Baltic Sea, 13.0-8.0 ka BP. Quaternary International 27, 19–40. https://doi.org/10.1016/1040-6182(94)00057-C.
- Björck, S. & Clemmensen, L.B., 2004: Aeolian sediment in raised bog deposits, Halland, SW Sweden: a new proxy record of Holocene winter storminess variation in southern Scandinavia? The Holocene 14, 677–688. https://doi.org/10.1191/0959683604hl746rp.
- Björck, S., Kromer, B., Johnsen, S., Bennike, O., Hammarlund, D., Lemdahl, G., Possnert, G., Rasmussen, T.L., Wohlfarth, B., Hammer, C.U. & Spurk, M., 1996: Synchronized terrestrial-atmospheric deglacial records around the North Atlantic. Science 274, 1155–1160. https://doi.org/10.1126/science.274.5290.1155.
- Björck, S., Muscheler, R., Kromer, B., Andresen, C.S., Heinemeier, J., Johnsen, S.J., Conley, D., Koç, N., Spurk, M. & Veski, S., 2001: High-resolution analyses of an early Holocene climate event may imply decreased solar forcing as an important climate trigger. Geology 29, 1107–1110. https://doi.org/10.1130/0091-7613(2001)029<1107:HRAOAE>2.0.CO;2.
- Björck, S., Rundgren, M., Ingólfsson, Ó & Funder, S., 1997: The Preboreal oscillation around the Nordic seas: terrestrial and lacustrine responses. Journal of Quaternary Science 12, 455–465. https://doi.org/10.1002/(SICI)1099-1417(199711/12)12:6<455::AID-JQS316>3.0.CO;2-S.
- Bjune, A.E., Seppä, H. & Birks, H.J.B., 2009: Quantitative summer-temperature reconstructions for the last 2000 years based on pollen-stratigraphical data from northern Fennoscandia. Journal of Paleolimnology 41, 43–56. https://doi.org/10.1007/s10933-008-9254-y.
- Blaauw, M., van Geel, B. & van der Plicht, J., 2004: Solar forcing of climatic change during the mid-Holocene: indications from raised bogs in the Netherlands. The Holocene 14, 35–44. https://doi.org/10.1191/0959683604hl687rp.
- Blackford, J.J. & Chambers, F.M., 1991: Proxy records of climate from blanket mires: evidence for a Dark Age (1400 BP) climatic deterioration in the British Isles. The Holocene 1, 63–67. https://doi.org/10.1177/095968369100100108.
- Blytt, A., 1876: Essay on the immigration of the Norwegian Flora during alternating rainy and dry periods. Alb. Cammermeyer, Christiania.
- Bond, G., Showers, W., Cheseby, M., Lotti, R., Almasi, P., DeMenocal, P., Priore, P., Cullen, H., Hajdas, I. & Bonani, G., 1997: A pervasive millennial-scale cycle in North Atlantic Holocene and glacial climates. Science 278, 1257–1266. https://doi.org/10.1126/science.278.5341.1257.
- Borgmark, A. & Wastegård, S., 2008: Regional and local patterns of peat humification in three raised peat bogs in Värmland, south-central Sweden. GFF 130, 161–176. https://doi.org/10.1080/11035890809453231.
- Bradley, R.S. & Bakke, J., 2019: Is there evidence for a 4.2 ka BP event in the northern North Atlantic region? Climate of the Past 15, 1665–1676. https://doi.org/10.5194/cp-15-1665-2019.
- Briner, J.P., McKay, N.P., Axford, Y., Bennike, O., Bradley, R.S., de Vernal, A., Fisher, D., Francus, P., Fréchette, B., Gajewski, K., Jennings, A., Kaufman, D.S., Miller, G., Rouston, C. & Wagner, B., 2016: Holocene climate change in Arctic Canada and Greenland. Quaternary Science Reviews 147, 340–364. https://doi.org/10.1016/j.quascirev.2016.02.010.
- Bronk Ramsey, C., 2013: Recent and planned developments of the program OxCal. Radiocarbon 55, 720–730. https://doi.org/10.2458/azu_js_rc.55.16215.
- Brunnberg, L., 1995: Clay-varve chronology and deglaciation during the Younger Dryas and Preboreal in the easternmost part of the Middle Swedish End Marginal Zone. PhD thesis, Stockholm University, Department of Quaternary Research, Quaternaria A:2.
- Büntgen, U., Myglan, V.S., Ljungqvist, F.C., McCormick, M., Di Cosmo, N., Sigl, M., Jungclaus, J., Wagner, S., Krusic, P.J., Esper, J., Kaplan, J.O., De Vaan, M.A.C., Luterbacher, J., Wacker, L., Tegel, W. & Kirdyanov, A.V., 2016: Cooling and societal change during the late antique little ice age from 536 to around 660 AD. Nature Geoscience 9, 231–236. https://doi.org/10.1038/ngeo2652.
- Charman, D.J., Blundell, A., Chiverrell, R.C., Hendon, D. & Langdon, P.G., 2006: Compilation of non-annually resolved Holocene proxy climate records: stacked Holocene peatland palaeo-water table reconstructions from northern Britain. Quaternary Science Reviews 25, 336–350. https://doi.org/10.1016/j.quascirev.2005.05.005.
- Dahl, S.O., Nesje, A., Lie, Ø, Fjordheim, K. & Matthews, J.A., 2002: Timing, equilibrium-line altitudes and climatic implications of two early-Holocene glacier readvances during the Erdalen Event at Jostedalsbreen, western Norway. The Holocene 12, 17–25. https://doi.org/10.1191/0959683602hl516rp.
- Davies, S.M., Abbott, P.M., Pearce, N.J.G., Wastegård, S. & Blockley, S.P.E., 2012: Integrating the INTIMATE records using tephrochronology: rising to the challenge. Quaternary Science Reviews 36, 11–27. https://doi.org/10.1016/j.quascirev.2011.04.005.
- Davies, S.M., Wastegård, S. & Wohlfarth, B., 2003: Extending the limits of the Borrobol Tephra to Scandinavia and detection of new early Holocene tephras. Quaternary Research 59, 345–352. https://doi.org/10.1016/S0033-5894(03)00035-8.
- Davis, B.A.S., Brewer, S., Stevenson, A.C. & Guiot, J., 2003: The temperature of Europe during the Holocene reconstructed from pollen data. Quaternary Science Reviews 22, 1701–1716. https://doi.org/10.1016/S0277-3791(03)00173-2.
- de Jong, R., Björck, S., Björkman, L. & Clemmensen, L.B., 2006: Storminess variation during the last 6500 years as reconstructed from an ombrotrophic peat bog in Halland, southwest Sweden. Journal of Quaternary Science 21, 905–919. https://doi.org/10.1002/jqs.1011.
- de Jong, R., Schoning, K. & Björck, S., 2007: Increased aeolian activity during climatic regime shifts as recorded in a raised bog in south-west Sweden during the past 1700 years. Climate of the Past Discussions 3, 383–408. https://doi.org/10.5194/cpd-3-383-2007.
- Denton, G.H. & Karlén, W., 1973: Holocene climatic variations – their pattern and possible cause. Quaternary Research 3, 155–205. https://doi.org/10.1016/0033-5894(73)90040-9.
- Digerfeldt, G., 1988: Reconstruction and regional correlation of Holocene lake-level fluctuations in Lake Bysjön, South Sweden. Boreas 17, 165–182. https://doi.org/10.1111/j.1502-3885.1988.tb00544.x.
- Edvardsson, J., Linderson, H., Rundgren, M. & Hammarlund, D., 2012: Holocene peatland development and hydrological variability inferred from bog-pine dendrochronology and peat stratigraphy – a case study from southern Sweden. Journal of Quaternary Science 27, 553–563. https://doi.org/10.1002/jqs.2543.
- Engels, S., 2021: The influence of Holocene forest dynamics on the chironomid fauna of a Boreal Lake (Flocktjärn, northeast Sweden). Boreas 50, 519–534. https://doi.org/10.1111/bor.12497.
- Fisher, T.G., Smith, D.G. & Andrews, J.T., 2002: Preboreal oscillation caused by a glacial Lake Agassiz flood. Quaternary Science Reviews 21, 873–878. https://doi.org/10.1016/S0277-3791(01)00148-2.
- Franzén, L., 2002: Tillväxtdynamik hos några myrar i södra Sverige – samt några noteringar om torvmarker som arkiv över atmosfärshändelser under holocen, Stiftelsen Svensk Torvforskning Projektrapport 47.
- Fredén, C., 1988: Marine life and deglaciation chronology of the Vänern basin, southwestern Sweden, Sveriges Geologiska Undersökning Ca 71, 80 pp.
- Frödin, J., 1921: De senglaciala isdämda sjöarna i örorsta delen av Stora Lule älvs flodområde och deras dräneringsvägar. Geologiska Föreningens i Stockholm Förhandlingar 43, 53–69. https://doi.org/10.1080/11035892109443888.
- Gedda, B., 2006: Terrestrial mollusc succession and stratigraphy of a Holocene calcareous tufa deposit from the Fyledalen valley, southern Sweden. The Holocene 16, 137–147. https://doi.org/10.1191/0959683606hl914rr.
- Göransson, H., 1977: The Flandrian Vegetational History of Southern Östergötland, University of Lund, Department of Quaternary Geology. LUNDQUA Thesis 3.
- Granlund, E., 1932: De svenska högmossarnas geologi. Sveriges Geologiska Undersökning C 373, 1–193.
- Gräslund, B., 2007: Fimbulvintern, Ragnarök och klimatkrisen år 536-537 e. Kr. Saga och sed: Kungl. Gustav Adolfs Akademiens årsbok 2007, 93–123.
- Gräslund, B. & Price, N., 2012: Twilight of the gods? The “dust veil event” of AD 536 in critical perspective. Antiquity 86, 428–443. https://doi.org/10.1017/S0003598X00062852.
- Grudd, H., 2008: Torneträsk tree-ring width and density AD 500-2004: a test of climatic sensitivity and a new 1500-year reconstruction of north Fennoscandian summers. Climate Dynamics 31, 843–857. https://doi.org/10.1007/s00382-007-0358-2.
- Grudd, H., Briffa, K.R., Karlén, W., Bartholin, T.S., Jones, P.D. & Kromer, B., 2002: A 7400-year tree-ring chronology in northern Swedish Lapland: natural climatic variability expressed on annual to millennial timescales. The Holocene 12, 657–665. https://doi.org/10.1191/0959683602hl578rp.
- Gulliksen, S., Birks, H.H., Possnert, G. & Mangerud, J., 1998: A calendar age estimate of the younger Dryas-Holocene boundary at Krakenes, Western Norway. Holocene 8, 249–259. https://doi.org/10.1191/095968398672301347.
- Gunnarson, B.E., 2008: Temporal distribution pattern of subfossil pines in central Sweden: perspective on Holocene humidity fluctuations. The Holocene 18, 569–577. https://doi.org/10.1177/0959683608089211.
- Gunnarson, B.E., Borgmark, A. & Wastegård, S., 2003: Holocene humidity fluctuations in Sweden inferred from dendrochronology and peat stratigraphy. Boreas 32, 347–360. https://doi.org/10.1080/03009480310001641.
- Hammarlund, D., Björck, S., Buchardt, B., Israelson, C. & Thomsen, C.T., 2003: Rapid hydrological changes during the Holocene revealed by stable isotope records of lacustrine carbonates from Lake Igelsjön, southern Sweden. Quaternary Science Reviews 22, 353–370. https://doi.org/10.1016/S0277-3791(02)00091-4.
- Hammarlund, D. & Lemdahl, G., 1994: A Late Weichselian stable isotope stratigraphy compared with biostratigraphical data: a case study from southern Sweden. Journal of Quaternary Science 9, 13–31. https://doi.org/10.1002/jqs.3390090103.
- Hammarlund, D., Velle, G., Wolfe, B.B., Edwards, T.W.D., Barnekow, L., Bergman, J., Holmgren, S., Lamme, S., Snowball, I., Wohlfarth, B. & Possnert, G., 2004: Palaeolimnological and sedimentary responses to Holocene forest retreat in the Scandes Mountains, west-central Sweden. The Holocene 14, 862–876. https://doi.org/10.1191/0959683604hl756rp.
- Harrison, S.P. & Digerfeldt, G., 1993: European lakes as palaeohydrological and palaeoclimatic indicators. Quaternary Science Reviews 12, 233–248.
- Heikkilä, M. & Seppä, H., 2003: A 11,000 yr palaeotemperature reconstruction from the southern boreal zone in Finland. Quaternary Science Reviews 22, 541–554. https://doi.org/10.1016/S0277-3791(02)00189-0.
- Helama, S., Jones, P.D. & Briffa, K.R., 2017: Dark ages cold period: a literature review and directions for future research. The Holocene 27, 1600–1606. https://doi.org/10.1177/0959683617693898.
- Högbom, A.G., 1892: Om märken efter isdämda sjöar i Jemtlands fjelltrakter. Geologiska Föreningens i Stockholm Förhandlingar 14, 561–582. https://doi.org/10.1080/11035899209445449.
- Holmlund, P., Karlén, W., Grudd, H., 1996: Fifty years of mass balance and Glacier front observations at the Tarfala Research Station. Geografiska Annaler A78, 105–114. https://doi.org/10.1080/04353676.1996.11880456.
- Hoppe, G., 1959: Glacial morphology and inland ice recession in northern Sweden. Geografiska Annaler 41, 193–212. https://doi.org/10.1080/20014422.1959.11907951.
- Hormes, A., Karlén, W. & Possnert, G., 2004: Radiocarbon dating of palaeosol components in moraines in Lapland, northern Sweden. Quaternary Science Reviews 23, 2031–2043. https://doi.org/10.1016/j.quascirev.2004.02.004.
- Hughes, P.D.M., Mauquoy, D., Barber, K.E. & Langdon, P.G., 2000: Mire-development pathways and palaeoclimatic records from a full Holocene peat archive at Walton Moss, Cumbria, England. Holocene 10, 465–479. https://doi.org/10.1191/095968300675142023.
- Hulth, J.M., 1895: Om floran i några kalktuffer från Vestergötland. Botaniska Notiser 5, 199–203.
- Iversen, J., 1944: Viscum, Hedera and Ilex as Climate Indicators. Geologiska Föreningens i Stockholm Förhandlingar 66, 463–483.
- Iversen, J., 1954: The Late-Glacial flora of Denmark and its relation to climate and soil. Danmarks Geologiske Undersøgelse, Række II. pp. 87–119.
- Jessen, C.A., Rundgren, M., Björck, S. & Hammarlund, D., 2005: Abrupt climatic changes and an unstable transition into a late Holocene thermal decline: a multiproxy lacustrine record from southern Sweden. Journal of Quaternary Science 20, 349–362. https://doi.org/10.1002/jqs.921.
- Karlén, W., 1988: Scandinavian glacial and climatic fluctuations during the Holocene. Quaternary Science Reviews 7, 199–209. https://doi.org/10.1016/0277-3791(88)90006-6.
- Karlén, W. & Kuylenstierna, J., 1996: On solar forcing of Holocene climate: evidence from scandinavia. The Holocene 6, 359–365. https://doi.org/10.1177/095968369600600311.
- Kaufman, D.S., Ager, T.A., Anderson, N.J., Anderson, P.M., Andrews, J.T., Bartlein, P.J., Brubaker, L.B., Coats, L.L., Cwynar, L.C., Duvall, M.L., Dyke, A.S., Edwards, M.E., Eisner, W.R., Gajewski, K., Geirsdóttir, A., Hu, F.S., Jennings, A.E., Kaplan, M.R., Kerwin, M.W., Lozhkin, A.V., MacDonald, G.M., Miller, G.H., Mock, C.J., Oswald, W.W., Otto-Bliesner, B.L., Porinchu, D.F., Rühland, K., Smol, J.P., Steig, E.J. & Wolfe, B.B., 2004: Holocene thermal maximum in the western Arctic (0-180°W). Quaternary Science Reviews 23, 529–560. https://doi.org/10.1016/j.quascirev.2003.09.007.
- Kullman, L., 1995: Holocene tree-limit and climate history from the Scandes Mountains, Sweden. Ecology 76, 2490–2502. https://doi-org.ezp.sub.su.se/10. 2307/2265823.
- Kullman, L., 1999: Early Holocene tree growth at a high elevation site in the northernmost Scandes of Sweden (Lapland): a palaeobiogeographical case study based on Megafossil evidence. Geografiska Annaler: Series A, Physical Geography 81, 63–74. https://doi.org/10.1111/j.0435-3676.1999.00049.x.
- Kullman, L. & Öberg, L., 2009: Post-little ice age tree line rise and climate warming in the Swedish Scandes: a landscape ecological perspective. Journal of Ecology 97, 415–429. https://doi.org/10.1111/j.1365-2745.2009.01488.x.
- Kullman, L., 2002: Rapid recent range-margin rise of tree and shrub species in the Swedish Scandes. Journal of Ecology 90, 68–77. https://doi.org/10.1046/j.0022-0477.2001.00630.x.
- Kullman, L., 2004: Tree-limit landscape evolution at the southern fringe of the Swedish Scandes (Dalarna province) – Holocene and 20th century perspectives. Fennia 182, 73–94.
- Kullman, L., 2013: Ecological tree line history and palaeoclimate – review of megafossil evidence from the Swedish Scandes. Boreas 42, 555–567. https://doi.org/10.1111/bor.12003.
- Kylander, M.E., Bindler, R., Cortizas, A.M., Gallagher, K., Mörth, C.M. & Rauch, S., 2013: A novel geochemical approach to paleorecords of dust deposition and effective humidity: 8500 years of peat accumulation at Store Mosse (the “Great Bog”), Sweden. Quaternary Science Reviews 69, 69–82. https://doi.org/10.1016/j.quascirev.2013.02.010.
- Lamb, H., 1977: Climate: Present, Past and Future (Vol. 2), 2nd ed. Methuen Publishing, London.
- Lamb, H., 1982: Climate, History and the Modern World. Routledge, Boca Raton, FL.
- Lane, C.S., Brauer, A., Blockley, S.P.E. & Dulski, P., 2013: Volcanic ash reveals time-transgressive abrupt climate change during the Younger Dryas. Geology 41, 1251–1254. https://doi.org/10.1130/G34867.1.
- Langdon, P.G. & Barber, K.E., 2005: The climate of Scotland over the last 5000 years inferred from multiproxy peatland records: inter-site correlations and regional variability. Journal of Quaternary Science 20, 549–566. https://doi.org/10.1002/jqs.934.
- Larsson, T.B., Rosqvist, G., Ericsson, G. & Heinerud, J., 2012: Climate change, Mooses and Humans in Northern Sweden 4000 cal. yr. BP. Journal of Northern Studies 6, 9–30.
- Leijonhufvud, L., Wilson, R. & Moberg, A., 2008: Documentary data provide evidence of Stockholm average winter to spring temperatures in the eighteenth and nineteenth centuries. The Holocene 18, 333–343. https://doi.org/10.1177/0959683607086770.
- Leijonhufvud, L., Wilson, R., Moberg, A., Söderberg, J., Retsö, D. & Söderlind, U., 2010: Five centuries of Stockholm winter/spring temperatures reconstructed from documentary evidence and instrumental observations. Climatic Change 101, 109–141. https://doi.org/10.1007/s10584-009-9650-y.
- Lemdahl, G., 1991: A rapid climatic change at the end of the Younger Dryas in south Sweden – palaeoclimatic and palaeoenvironmental reconstructions based on fossil insect assemblages. Palaeogeography, Palaeoclimatology, Palaeoecology 83, 313–331. https://doi.org/10.1016/0031-0182(91)90058-Y.
- Lind, E.M., Wastegård, S. & Larsen, J.J., 2013: A late Younger Dryas-Early Holocene tephrostratigraphy for Fosen, Central Norway. Journal of Quaternary Science 28, 803–811. https://doi.org/10.1002/jqs.2676.
- Lindén, M., Möller, P., Björck, S. & Sandgren, P., 2006: Holocene shore displacement and deglaciation chronology in Norrbotten, Sweden. Boreas 35, 1–22. https://doi.org/10.1111/j.1502-3885.2006.tb01109.x.
- Linderholm, H.W. & Gunnarson, B.E., 2005: Summer temperature variability in central Scandinavian during the last 3600 years. Geografiska Annaler, Series A: Physical Geography 87, 231–241. https://doi.org/10.1111/j.0435-3676.2005.00255.x.
- Linderholm, H.W., Nicolle, M., Francus, P., Gajewski, K., Helama, S., Korhola, A., Solomina, O., Yu, Z., Zhang, P., D’Andrea, W.J., Debret, M., Divine, D.V., Gunnarson, B.E., Loader, N.J., Massei, N., Seftigen, K., Thomas, E.K., Werner, J., Andersson, S., Berntsson, A., Luoto, T.P., Nevalainen, L., Saarni, S. & Väliranta, M., 2018: Arctic hydroclimate variability during the last 2000 years: current understanding and research challenges. Climate of the Past 14, 473–514. https://doi.org/10.5194/cp-14-473-2018.
- Ljungqvist, F.C., 2009: Temperature proxy records covering the last two millennia: a tabular and visual overview. Geografiska Annaler, Series A: Physical Geography 91, 11–29. https://doi.org/10.1111/j.1468-0459.2009.00350.x.
- Lundqvist, G., 1924: Sedimentationstyper i insjöarna. Geologiska Föreningens i Stockholm Förhandlingar 46, 56–75. https://doi.org/10.1080/11035892409444878.
- Lundqvist, G., 1940: Sjösediment från Gotland. Sveriges Geologiska Undersökning C 434, 143.
- Lundqvist, G., 1958: Kvartärgeologisk forskning i Sverige under ett sekel. Sveriges Geologiska Undersökning C 561, 62.
- Lundqvist, J. & Wohlfarth, B., 2000: Timing and east-west correlation of south Swedish ice marginal lines during the Late Weichselian. Quaternary Science Reviews 20, 1127–1148. https://doi.org/10.1016/S0277-3791(00)00142-6.
- Lundqvist, J., 1986: Late Weichselian glaciation and deglaciation in Scandinavia. Quaternary Science Reviews 5, 269–292. https://doi.org/10.1016/0277-3791(86)90192-7.
- Lundqvist, J., 1988: Younger Dryas-Preboreal moraines and deglaciation in southwestern Värmland, Sweden. Boreas 17, 301–316. https://doi.org/10.1111/j.1502-3885.1988.tb00961.x.
- Lundqvist, J., 2003: Deglaciation pattern in subaquatic – supra-aquatic transitional environment illustrated by the Klarälven valley system, Värmland, western Sweden. Geografiska Annaler, Series A: Physical Geography 85, 73–89. https://doi.org/10.1111/1468-0459.00190.
- Luoto, T.P., 2013: How cold was the Little Ice Age? A proxy-based reconstruction from Finland applying modern analogues of fossil midge assemblages. Environmental Earth Sciences 68, 1321–1329. https://doi.org/10.1007/s12665-012-1830-9.
- MacDonald, G.M., Beilman, D.W., Kremenetski, K.V., Sheng, Y., Smith, L.C. & Velichko, A.A., 2006: Rapid early development of circumarctic Peatlands and atmospheric CH4 and CO2 variations. Science 314, 285–288. https://doi.org/10.1126/science.1131722.
- MacLeod, A., Brunnberg, L., Wastegård, S., Hang, T. & Matthews, I.P., 2014: Lateglacial cryptotephra detected within clay varves in Östergötland, south-east Sweden. Journal of Quaternary Science 29, 605–609. https://doi.org/10.1002/jqs.2738.
- Magny, M. & Haas, J.N., 2004: A major widespread climatic change around 5300 cal. yr BP at the time of the Alpine Iceman. Journal of Quaternary Science 19, 423–430. https://doi.org/10.1002/jqs.850.
- Mallon, G., 2012: Patterns of mid-Holocene climate change – evidence from the peat archive. Doctoral Thesis. University of Southampton, Geography.
- Mangerud, J., Andersen, S.T., Berglund, B.E. & Donner, J.J., 1974: Quaternary stratigraphy of Norden, a proposal for terminology and classification. Boreas 3, 109–126. https://doi.org/10.1111/j.1502-3885.1974.tb00669.x.
- Marcott, S.A., Shakun, J.D., Clark, P.U. & Mix, A.C., 2013: A reconstruction of regional and global temperature for the past 11,300 years. Science 339, 1198–1201. https://doi.org/10.1126/science.1228026.
- Mauquoy, D. & Barber, K., 2002: Testing the sensitivity of the palaeoclimatic signal from ombrotrophic peat bogs in northern England and the Scottish Borders. Review of Palaeobotany and Palynology 119, 219–240. https://doi.org/10.1016/S0034-6667(01)00099-9.
- Mayewski, P.A., Rohling, E.E., Stager, J.C., Karlén, W., Maasch, K.A., Meeker, L.D., Meyerson, E.A., Gasse, F., van Kreveld, S., Holmgren, K., Lee-Thorp, J., Rosqvist, G., Rack, F., Staubwasser, M., Schneider, R.R. & Steig, E.J., 2004: Holocene climate variability. Quaternary Research 62, 243–255. https://doi.org/10.1016/j.yqres.2004.07.001.
- Mellström, A., van der Putten, N., Muscheler, R., De Jong, R. & Björck, S., 2015: A shift towards wetter and windier conditions in southern Sweden around the prominent solar minimum 2750 cal a BP. Journal of Quaternary Science 30, 235–244. https://doi.org/10.1002/jqs.2776.
- Miller, G.H., Brigham-Grette, J., Alley, R.B., Anderson, L., Bauch, H.A., Douglas, M.S.V., Edwards, M.E., Elias, S.A., Finney, B.P., Fitzpatrick, J.J., Funder, S.V., Herbert, T.D., Hinzman, L.D., Kaufman, D.S., MacDonald, G.M., Polyak, L., Robock, A., Serreze, M.C., Smol, J.P., Spielhagen, R., White, J.W.C., Wolfe, A.P. & Wolff, E.W., 2010: Temperature and precipitation history of the Arctic. Quaternary Science Reviews 29, 1679–1715. https://doi.org/10.1016/j.quascirev.2010.03.001.
- Moberg, A., Sonechkin, D.M., Holmgren, K., Datsenko, M.H. & Karlén, W., 2005: Highly variable Northern Hemisphere temperatures reconstructed from low- and high-resolution proxy data. Nature 433, 613–617. https://doi.org/10.1038/nature03265.
- Möller, P., Östlund, O., Barnekow, L., Sandgren, P., Palmbo, F. & Willerslev, E., 2013: Living at the margin of the retreating Fennoscandian Ice Sheet: The early Mesolithic sites at Aareavaara, northernmost Sweden. The Holocene 23, 104–116. https://doi.org/10.1177/0959683612455546.
- Muschitiello, F., Lea, J.M., Greenwood, S.L., Nick, F.M., Brunnberg, L., MacLeod, A. & Wohlfarth, B., 2016: Timing of the first drainage of the Baltic Ice Lake synchronous with the onset of Greenland Stadial 1. Boreas 45, 322–334. https://doi.org/10.1111/bor.12155.
- Muschitiello, F. & Wohlfarth, B., 2015: Time-transgressive environmental shifts across Northern Europe at the onset of the Younger Dryas. Quaternary Science Reviews 109, 49–56. https://doi.org/10.1016/j.quascirev.2014.11.015.
- Nesje, A. & Dahl, S.O., 2001: The Greenland 8200 cal. yr BP event detected in loss-on-ignition profiles in Norwegian lacustrine sediment sequences. Journal of Quaternary Science 16, 155–166. https://doi.org/10.1002/jqs.567.
- Nesje, A., Matthews, J.A., Dahl, S.O., Berrisford, M.S. & Andersson, C., 2001: Holocene glacier fluctuations of Flatebreen and winter-precipitation changes in the Jostedalsbreen region, western Norvay, based on glaciolacustrine sediment records. The Holocene 11, 267–280. https://doi.org/10.1191/095968301669980885.
- Nesje, A., Dahl, S.O., Thun, T. & Nordli, Ø, 2008: The “Little Ice Age” glacial expansion in western Scandinavia: summer temperature or winter precipitation? Climate Dynamics 30, 789–801. https://doi.org/10.1007/s00382-007-0324-z.
- Nesje, A., 2009: Latest Pleistocene and Holocene alpine glacier fluctuations in Scandinavia. Quaternary Science Reviews 28, 2119–2136. https://doi.org/10.1016/j.quascirev.2008.12.016.
- Nilsson, T., 1959: Aktuella utvecklingslinjer inom svensk allmän kvartärgeologi. Geologiska Föreningens i Stockholm Förhandlingar 81, 127–138. https://doi.org/10.1080/11035895909449136.
- Öberg, L. & Kullman, L., 2011: Recent glacier recession – a new source of postglacial treeline and climate history in the Swedish Scandes. Landscape Online 26, 1–38. https://doi.org/10.3097/LO.201126.
- O’Brien, S.R., Mayewski, P.A., Meeker, L.D., Meese, D.A., Twickler, M.S. & Whitlow, S.I., 1995: Complexity of Holocene climate as reconstructed from a Greenland Ice core. Science 270, 1962–1964. https://doi.org/10.1126/science.270.5244.1962.
- Ojala, A.E.K., Heinsalu, A., Kauppila, T., Alenius, T. & Saarnisto, M., 2008: Characterizing changes in the sedimentary environment of a varved lake sediment record in southern central Finland around 8000 cal. yr BP. Journal of Quaternary Science 23, 765–775. https://doi.org/10.1002/jqs.1157.
- Paus, A., 2013: Human impact, soil erosion, and vegetation response lags to climate change: challenges for the mid-Scandinavian pollen-based transfer-function temperature reconstructions. Vegetation History and Archaeobotany 22, 269–284. https://doi.org/10.1007/s00334-012-0360-4.
- Paus, A. & Haugland, V., 2017: Early- to mid-Holocene forest-line and climate dynamics in southern Scandes Mountains inferred from contrasting megafossil and pollen data. The Holocene 27, 361–383. https://doi.org/10.1177/0959683616660172.
- Pearce, C., Seidenkrantz, M.S., Kuijpers, A., Massé, G., Reynisson, N.F. & Kristiansen, S.M., 2013: Ocean Lead at the termination of the Younger Dryas cold spell. Nature Communications 4, 8–13. https://doi.org/10.1038/ncomms2686.
- Price, T.D., 2015: Ancient Scandinavia: An Archaeological History from the First Humans to the Vikings. Oxford University Press, New York, USA.
- Rainio, H., Saarnisto, M. & Ekman, I., 1995: Younger Dryas end moraines in Finland and NW Russia. Quaternary International 28, 179–192. https://doi.org/10.1016/1040-6182(95)00051-J.
- Rasmussen, S.O., Andersen, K.K., Svensson, A.M., Steffensen, J.P., Vinther, B.M., Clausen, H.B., Siggaard-Andersen, M.L., Johnsen, S.J., Larsen, L.B., Dahl-Jensen, D., Bigler, M., Röthlisberger, R., Fischer, H., Goto-Azuma, K., Hansson, M.E. & Ruth, U., 2006: A new Greenland ice core chronology for the last glacial termination. Journal of Geophysical Research Atmospheres 111, 1–16. https://doi.org/10.1029/2005JD006079.
- Rasmussen, S.O., Vinther, B.M., Clausen, H.B. & Andersen, K.K., 2007: Early Holocene climate oscillations recorded in three Greenland ice cores. Quaternary Science Reviews 26, 1907–1914. https://doi.org/10.1016/j.quascirev.2007.06.015.
- Regnéll, C., Mangerud, J. & Svendsen, J.I., 2019: Tracing the last remnants of the Scandinavian Ice sheet: ice-dammed lakes and a catastrophic outburst flood in northern Sweden. Quaternary Science Reviews 221, 105862. https://doi.org/10.1016/j.quascirev.2019.105862.
- Reimer, P.J., Austin, W.E.N., Bard, E., Bayliss, A., Blackwell, P.G., Bronk Ramsey, C., Butzin, M., Cheng, H., Edwards, R.L., Friedrich, M., Grootes, P.M., Guilderson, T.P., Hajdas, I., Heaton, T.J., Hogg, A.G., Hughen, K.A., Kromer, B., Manning, S.W., Muscheler, R., Palmer, J.G., Pearson, C., van der Plicht, J., Reimer, R.W., Richards, D.A., Scott, E.M., Southon, J.R., Turney, C.S.M., Wacker, L., Adolphi, F., Büntgen, U., Capano, M., Fahrni, S.M., Fogtmann-Schulz, A., Friedrich, R., Köhler, P., Kudsk, S., Miyake, F., Olsen, J., Reinig, F., Sakamoto, M., Sookdeo, A. & Talamo, S., 2020: The IntCal20 Northern Hemisphere Radiocarbon Age Calibration Curve (0–55 cal kBP). Radiocarbon 62, 725–757.https://doi.org/10.1017/RDC.2020.41.
- Renssen, H., Seppä, H., Heiri, O., Roche, D.M., Goosse, H. & Fichefet, T., 2009: The spatial and temporal complexity of the Holocene thermal maximum. Nature Geoscience 2, 411–414. https://doi.org/10.1038/ngeo513.
- Retsö, D., 2002: A contribution to the history of European winters: some climatological proxy data from early-sixteenth century Swedish documentary sources. Climatic Change 52, 137–173. https://doi.org/10.1023/A:1013093410975.
- Retsö, D., 2015: Documentary evidence of historical floods and extreme rainfall events in Sweden 1400-1800. Hydrology and Earth System Sciences 19, 1307–1323. https://doi.org/10.5194/hess-19-1307-2015.
- Retsö, D. & Söderberg, J., 2019: Winter severity in medieval Sweden. In Kiss A. & Pribyl K (eds.): The Dance of Death in Late Medieval and Renaissance Europe: environmental stress, mortality and social response (1st Ed.), 24–45. Routledge, London.
- Retsö, D. & Leijonhufvud, L., 2020: Documentary evidence of droughts in Sweden between the Middle Ages and c1800 (preprint). Climate of the Past. Discussion. https://doi.org/10.5194/cp-2020-25.
- Rohling, E.J. & Pälike, H., 2005: Centennial-scale climate cooling with a sudden cold event around 8,200 years ago. Nature 434, 975–979. https://doi.org/10.1038/nature03421.
- Roland, T.P., Caseldine, C.J., Charman, D.J., Turney, C.S.M. & Amesbury, M.J., 2014: Was there a “4.2 ka event” in Great Britain and Ireland? Evidence from the peatland record. Quaternary Science Reviews 83, 11–27. https://doi.org/10.1016/j.quascirev.2013.10.024.
- Rosén, P., Segerström, U., Eriksson, L., Renberg, I. & Birks, H.J.B., 2001: Holocene climatic change reconstructed from diatoms, chironomids, pollen and near-infrared spectroscopy at an alpine lake (Sjuodjijaure) in northern Sweden. The Holocene 11, 551–562. https://doi.org/10.1191/095968301680223503.
- Rosqvist, G., Jonsson, C., Yam, R., Karlén, W. & Shemesh, A., 2004: Diatom oxygen isotopes in pro-glacial lake sediments from northern Sweden: a 5000 year record of atmospheric circulation. Quaternary Science Reviews 23, 851–859. https://doi.org/10.1016/j.quascirev.2003.06.009.
- Rosqvist, G.C., Leng, M.J., Goslar, T., Sloane, H.J., Bigler, C., Cunningham, L., Dadal, A., Bergman, J., Berntsson, A., Jonsson, C., Wastegård, S., 2013: Shifts in precipitation during the last millennium in northern Scandinavia from lacustrine isotope records. Quaternary Science Reviews 66, 22–34. https://doi.org/10.1016/j.quascirev.2012.10.030.
- Rubensdotter, L. & Rosqvist, G., 2003: The effect of geomorphological setting on Holocene lake sediment variability, northern Swedish Lapland. Journal of Quaternary Science 18, 757–767. https://doi.org/10.1002/jqs.800.
- Rundgren, M., 2008: Stratigraphy of peatlands in central and northern Sweden: evidence of Holocene climatic change and peat accumulation. GFF 130, 95–107. https://doi.org/10.1080/11035890801302095.
- Rundgren, M. & Björck, S., 2003: Late-glacial and early Holocene variations in atmospheric CO2 concentration indicated by high-resolution stomatal index data. Earth and Planetary Science Letters 213, 191–204. https://doi.org/10.1016/S0012-821X(03)00324-8.
- Rundqvist, S., Hedenås, H., Sandström, A., Emanuelsson, U., Eriksson, H., Jonasson, C. & Callaghan, T.V., 2011: Tree and shrub expansion over the past 34 years at the tree-line near Abisko, Sweden. Ambio 40, 683–692. https://doi.org/10.1007/s13280-011-0174-0.
- Sandegren, R., 1937: Jordarterna (kvartära bildningar) in “Beskrivning till kartbladet Forshaga”. Sveriges Geologiska Undersökning Aa 179, 48–111.
- Sannel, A.B.K., Hempel, L., Kessler, A. & Prėskienis, V., 2018: Holocene development and permafrost history in sub-Arctic peatlands in Tavvavuoma, northern Sweden. Boreas 47, 454–468. https://doi.org/10.1111/bor.12276.
- Schenk, F., Bennike, O., Väliranta, M., Avery, R., Björck, S. & Wohlfarth, B., 2020: Floral evidence for high summer temperatures in southern Scandinavia during 15–11 cal ka BP. Quaternary Science Reviews 233, 106243. https://doi.org/10.1016/j.quascirev.2020.106243.
- Schoning, K., 2001: The brackish Baltic Sea Yoldia stage – palaeoenvironmental implications from marine benthic fauna and stable oxygen isotopes. Boreas 30, 290–298. https://doi.org/10.1111/j.1502-3885.2001.tb01048.x.
- Seppä, H., Bjune, A.E., Telford, R.J., Birks, H.J.B. & Veski, S., 2009: Last nine-thousand years of temperature variability in Northern Europe. Climate of the Past 5, 523–535. https://doi.org/10.5194/cp-5-523-2009.
- Seppä, H., Hammarlund, D. & Antonsson, K., 2005: Low-frequency and high-frequency changes in temperature and effective humidity during the Holocene in south-central Sweden: implications for atmospheric and oceanic forcings of climate. Climate Dynamics 25, 285–297. https://doi.org/10.1007/s00382-005-0024-5.
- Sernander, R., 1915: Svenska kalktuffer. Geologiska Föreningens i Stockholm Förhandlingar 37, 521–554. https://doi.org/10.1080/11035891509444833.
- Sernander, R., 1908: On the evidences of postglacial changes of climate furnished by the peat-mosses of Northern Europe. Geologiska Föreningens i Stockholm Förhandlingar 30, 465–473. https://doi.org/10.1080/11035890809445601.
- Shakesby, R.A., Matthews, J.A., Winkler, S., Fabel, D. & Dresser, P.Q., 2020: Early-Holocene moraine chronology, Sognefjell area, southern Norway: evidence for multiple glacial and climatic fluctuations within the Erdalen Event (∼10.2-9.7 ka). Norwegian Journal of Geology / Norsk Geologisk Forening 100, 1–28.
- Shala, S., Helmens, K.F., Luoto, T.P., Salonen, J.S., Väliranta, M. & Weckström, J., 2017: Comparison of quantitative Holocene temperature reconstructions using multiple proxies from a northern boreal lake. The Holocene 27, 1745–1755. https://doi.org/10.1177/0959683617708442.
- Shemesh, A., Rosqvist, G., Rietti-Shati, M., Rubensdotter, L., Bigler, C., Yam, R. & Karlén, W., 2001: Holocene climatic change in Swedish Lapland inferred from an oxygen-isotope record of lacustrine biogenic silica. The Holocene 11, 447–454. https://doi.org/10.1191/095968301678302887.
- Snowball, I. & Sandgren, P., 1996: Lake sediment studies of Holocene glacial activity in the Kårsa valley, northern Sweden: contrasts in interpretation. The Holocene 6, 367–372. https://doi.org/10.1177/095968369600600312.
- Snowball, I., Sandgren, P. & Petterson, G., 1999: The mineral magnetic properties of an annually laminated Holocene lake-sediment sequence in northern Sweden. The Holocene 9, 353–362. https://doi.org/10.1191/095968399670520633.
- Snowball, I., Korhola, A., Briffa, K.R. & Koç, N., 2004: Holocene climate dynamics in Fennoscandia and the north atlantic. In Battarbee R.W (ed.): Past Climate Variability Through Europe and Africa, 465–494. Kluwer Academic Publishers, Dordrecht, the Netherlands.
- Snowball, I., Zillén, L. & Gaillard, M.J., 2002: Rapid early-Holocene environmental changes in northern Sweden based on studies of two varved lake-sediment sequences. The Holocene 12, 7–16. https://doi.org/10.1191/0959683602hl515rp.
- Snowball, I., Muscheler, R., Zillén, L., Sandgren, P., Stanton, T. & Ljung, K., 2010: Radiocarbon wiggle matching of Swedish lake varves reveals asynchronous climate changes around the 8.2 kyr cold event. Boreas 39, 720–733. https://doi.org/10.1111/j.1502-3885.2010.00167.x.
- Sommer, R.S., Lindqvist, C., Persson, A., Bringsøe, H., Rhodin, A.G.J., Schneeweiss, N., Široký, P., Bachmann, L. & Fritz, U., 2009: Unexpected early extinction of the European pond turtle (Emys orbicularis) in Sweden and climatic impact on its Holocene range. Molecular Ecology 18, 1252–1262. https://doi.org/10.1111/j.1365-294X.2009.04096.x.
- Sørensen, R., 1979: Late Weichselian deglaciation in the Oslofjord area, south Norway. Boreas 8, 241–246. https://doi.org/10.1111/j.1502-3885.1979.tb00806.x.
- Sorrel, P., Debret, M., Billeaud, I., Jaccard, S.L., McManus, J.F. & Tessier, B., 2012: Persistent non-solar forcing of Holocene storm dynamics in coastal sedimentary archives. Nature Geoscience 5, 892–896. https://doi.org/10.1038/ngeo1619.
- St. Amour, N.A., Hammarlund, D., Edwards, T.W.D. & Wolfe, B.B., 2010: New insights into Holocene atmospheric circulation dynamics in central Scandinavia inferred from oxygen-isotope records of lake-sediment cellulose. Boreas 39, 770–782. https://doi.org/10.1111/j.1502-3885.2010.00169.x.
- Stroeven, A.P., Hättestrand, C., Kleman, J., Heyman, J., Fabel, D., Fredin, O., Goodfellow, B.W., Harbor, J.M., Jansen, J.D., Olsen, L., Caffee, M.W., Fink, D., Lundqvist, J., Rosqvist, G.C., Strömberg, B. & Jansson, K.N., 2016: Deglaciation of Fennoscandia. Quaternary Science Reviews 147, 91–121. https://doi.org/10.1016/j.quascirev.2015.09.016.
- Strömberg, B., 1994: Younger Dryas deglaciation at Mt. Billingen, and clay varve dating of the Younger Dryas/Preboreal transition. Boreas 23, 177–193. https://doi.org/10.1111/j.1502-3885.1994.tb00598.x.
- Subetto, D.A., Wohlfarth, B., Davydova, N.N., Sapelko, T.V., Björkman, L., Solovieva, N., Wastegård, S., Possnert, G. & Khomutova, V.I., 2002: Climate and environment on the Karelian Isthmus, northwestern Russia, 13000-9000 cal. yrs BP. Boreas 31, 1–19. https://doi.org/10.1111/j.1502-3885.2002.tb01051.x.
- Sundell, T., Kammonen, J., Halinen, P., Pesonen, P. & Onkamo, P., 2014: Archaeology, genetics and a population bottleneck in prehistoric Finland. Antiquity 88, 1132–1147. https://doi.org/10.1017/S0003598X00115364.
- Sundqvist, H.S., Holmgren, K. & Lauritzen, S.-E., 2007: Stable isotope variations in stalagmites from northwestern Sweden document climate and environmental changes during the early Holocene. The Holocene 17, 259–267. https://doi.org/10.1177/0959683607073292.
- Sundqvist, H.S., Holmgren, K., Moberg, A., Spötl, C. & Mangini, A., 2010: Stable isotopes in a stalagmite from NW Sweden document environmental changes over the past 4000 years. Boreas 39, 77–86. https://doi.org/10.1111/j.1502-3885.2009.00099.x.
- Svensson, G., 1988: Bog development and environmental conditions as shown by the stratigraphy of store mosse mire in southern Sweden. Boreas 17, 89–111. https://doi.org/10.1111/j.1502-3885.1988.tb00126.x.
- Swindles, G.T., Morris, P.J., Mullan, D.J., Payne, R.J., Roland, T.P., Amesbury, M.J., Lamentowicz, M., Turner, T.E., Gallego-Sala, A., Sim, T., Barr, I.D., Blaauw, M., Blundell, A., Chambers, F.M., Charman, D.J., Feurdean, A., Galloway, J.M., Gałka, M., Green, S.M., Kajukało, K., Karofeld, E., Korhola, A., Lamentowicz, Ł, Langdon, P., Marcisz, K., Mauquoy, D., Mazei, Y.A., McKeown, M.M., Mitchell, E.A.D., Novenko, E., Plunkett, G., Roe, H.M., Schoning, K., Sillasoo, Ü, Tsyganov, A.N., van der Linden, M., Väliranta, M. & Warner, B., 2019: Widespread drying of European peatlands in recent centuries. Nature Geoscience 12, 922–928. https://doi.org/10.1038/s41561-019-0462-z.
- Tallavaara, M. & Seppä, H., 2012: Did the mid-Holocene environmental changes cause the boom and bust of hunter-gatherer population size in eastern Fennoscandia? The Holocene 22, 215–225. https://doi.org/10.1177/0959683611414937.
- Teller, J.T., Leverington, D.W. & Mann, J.D., 2002: Freshwater outbursts to the oceans from glacial Lake Agassiz and their role in climate change during the last deglaciation. Quaternary Science Reviews 21, 879–887. https://doi.org/10.1016/S0277-3791(01)00145-7.
- Timms, R.G.O., Abrook, A.M., Matthews, I.P., Francis, C.P., Mroczkowska, A., Candy, I., Brooks, S.J., Milner, A.M. & Palmer, A.P., 2021: Evidence for centennial-scale Lateglacial and early Holocene climatic complexity from Quoyloo Meadow, Orkney, Scotland. Journal of Quaternary Science 36, 339–359. https://doi.org/10.1002/jqs.3282.
- Toohey, M., Krüger, K., Sigl, M., Stordal, F. & Svensen, H., 2016: Climatic and societal impacts of a volcanic double event at the dawn of the Middle Ages. Climatic Change 136, 401–412.
- Väliranta, M., Salonen, J.S., Heikkilä, M., Amon, L., Helmens, K., Klimaschewski, A., Kuhry, P., Kultti, S., Poska, A., Shala, S., Veski, S. & Birks, H.H., 2015: Plant macrofossil evidence for an early onset of the Holocene summer thermal maximum in northernmost Europe. Nature Communications 6, 1–8. https://doi.org/10.1038/ncomms7809.
- van der Linden, M., van Geel, B., 2006: Late Holocene climate change and human impact recorded in a south Swedish ombrotrophic peat bog. Palaeogeography, Palaeoclimatology, Palaeoecology 240, 649–667. https://doi.org/10.1016/j.palaeo.2006.03.039
- van Geel, B., Brinkkemper, O., van Reenen, G.B.A., van der Putten, N.N.L., Sybenga, J.E., Soonius, C., Kooijman, A.M., Hakbijl, T. & Gosling, W.D., 2020: Multicore study of upper Holocene Mire development in West-Frisia, Northern Netherlands: ecological and Archaeological aspects. Quaternary 3, 12. https://doi.org/10.3390/quat3020012.
- van Geel, B., Buurman, J. & Waterbolk, H.T., 1996: Archaeological and palaeoecological indications of an abrupt climate change in The Netherlands, and evidence for climatological teleconnections around 2650 BP. Journal of Quaternary Science 11, 451–460. https://doi.org/10.1002/(SICI)1099-1417(199611/12)11:6<451::AID-JQS275>3.0.CO;2-9.
- van Geel, B., Hallewas, D.P. & Pals, J.P., 1983: A late Holocene deposit under the Westfriese Zeedijk near Enkhuizen (Prov. of Noord-Holland, The Netherlands): palaeoecological and archaeological aspects. Review of Palaeobotany and Palynology 38, 269–335. https://doi.org/10.1016/0034-6667(83)90026-X.
- van Geel, B., Heusser, C., Renssen, H. & Schuurmans, C., 2000: Climatic change in Chile at around 2700 BP and global evidence for solar forcing: a hypothesis. The Holocene 10, 659–664. https://doi.org/10.1191/09596830094908.
- Vestøl, O., Ågren, J., Steffen, H., Kierulf, H. & Tarasov, L., 2019: NKG2016LU: a new land uplift model for Fennoscandia and the Baltic Region. Journal of Geodesy 93, 1759–1779. https://doi.org/10.1007/s00190-019-01280-8.
- von Post, L., 1916: Om skogsträdspollen i sydsvenska torvmosselagerföljder. Geologiska Föreningens i Stockholm Förhandlingar 38, 384–394.
- von Post, L., 1924: Ur de sydsvenska skogarnas regionala historia under post-arktisk tid. Geologiska Föreningens i Stockholm Förhandlingar 46, 83–128. https://doi.org/10.1080/11035892409444880.
- von Post, L., 1930: Die postarktische geschichte der Europäischen wälder nach den vorliegenden pollendiagrammen. Meddelanden Från Stockholms Högskolas Geologiska Institut 16, 1–27.
- von Post, L., 1934: Bonäslinjen. Geologiska Föreningens i Stockholm Förhandlingar 56, 19–59. https://doi.org/10.1080/11035893409448197.
- Vorren, K.-D., Blaauw, M., Wastegård, S., van der Plicht, J. & Jensen, C., 2007: High-resolution stratigraphy of the northernmost concentric raised bog in Europe: Sellevollmyra, Andøya, northern Norway. Boreas 36, 253–277. https://doi.org/10.1080/03009480601061152.
- Walker, M., Head, M.J., Berkelhammer, M., Björck, S., Cheng, H., Cwynar, L., Fisher, D., Gkinis, V., Long, A., Lowe, J., Newnham, R., Rasmussen, S.O. & Weiss, H., 2018: Formal ratification of the subdivision of the Holocene series/Epoch (Quaternary system/period): two new Global Boundary Stratotype Sections and Points (GSSPs) and three new stages/subseries. Episodes Journal of International Geoscience 41, 213–223. https://doi.org/10.18814/epiiugs/2018/018016.
- Walker, M., Head, M.J., Lowe, J., Berkelhammer, M., Björck, S., Cheng, H., Cwynar, L.C., Fisher, D., Gkinis, V., Long, A., Newnham, R., Rasmussen, S.O. & Weiss, H., 2019: Subdividing the Holocene series/epoch: formalization of stages/ages and subseries/subepochs, and designation of GSSPs and auxiliary stratotypes. Journal of Quaternary Science 34, 173–186. https://doi.org/10.1002/jqs.3097.
- Walker, M.J.C., Berkelhammer, M., Björck, S., Cwynar, L.C., Fisher, D.A., Long, A.J., Lowe, J.J., Newnham, R.M., Rasmussen, S.O. & Weiss, H., 2012: Formal subdivision of the Holocene series/epoch: a discussion paper by a working group of INTIMATE (integration of ice-core, marine and terrestrial records) and the subcommission on quaternary stratigraphy (international commission on Stratigraphy). Journal of Quaternary Science 27, 649–659. https://doi.org/10.1002/jqs.2565.
- Wanner, H., Beer, J., Bütikofer, J., Crowley, T.J., Cubasch, U., Flückiger, J., Goosse, H., Grosjean, M., Joos, F., Kaplan, J.O., Küttel, M., Müller, S.A., Prentice, I.C., Solomina, O., Stocker, T.F., Tarasov, P., Wagner, M. & Widmann, M., 2008: Mid- to Late Holocene climate change: an overview. Quaternary Science Reviews 27, 1791–1828. https://doi.org/10.1016/j.quascirev.2008.06.013.
- Wastegård, S., 1998: Deglaciation chronology and marine environments in southwestern Sweden. Boreas 27, 178–194. https://doi.org/10.1111/j.1502-3885.1998.tb00877.x.
- Weiss, H., 2019: Weiss review of Bradley and Bakke CP-2018-162 northern North Atlantic at 4.2 ka BP. Climate of the Past. Discussion. https://doi.org/10.5194/cp-2018-162-RC2.
- Widgren, M., 2012: Climate and causation in the Swedish Iron Age: learning from the present to understand the past. Geografisk -Danish Journal of Geography 112, 126–134. https://doi.org/10.1080/00167223.2012.741886.
- Wohlfarth, B., Björck, S., Possnert, G. & Holmquist, B., 1998: An 800-year long, radiocarbon-dated varve chronology from south-eastern Sweden. Boreas 27, 243–257. https://doi.org/10.1111/j.1502-3885.1998.tb01419.x.
- Wohlfarth, B., Luoto, T.P., Muschitiello, F., Väliranta, M., Björck, S., Davies, S.M., Kylander, M., Ljung, K., Reimer, P.J. & Smittenberg, R.H., 2018: Climate and environment in southwest Sweden 15.5–11.3 cal. ka BP. Boreas 47, 687–710. https://doi.org/10.1111/bor.12310.
- Wohlfarth, B., Muschitiello, F., Greenwood, S.L., Andersson, A., Kylander, M., Smittenberg, R.H., Steinthorsdóttir, M., Watson, J. & Whitehouse, N.J., 2017: Hässeldala – a key site for last termination climate events in Northern Europe. Boreas 46, 143–161. https://doi.org/10.1111/bor.12207.
- Zoller, H., 1960: Pollenanalytische Untersuchungen zur Vegetationsgeschichte der insubrischen Schweiz. Denkschrift der Schweizerischen Naturforschenden Gesellschaft 83, 45–156.
- Zuidhoff, F.S., 2003: Palsa growth and decay in northern Sweden. climatic and environmental controls. Doctoral Thesis. Acta Universitatis Upsaliensis. Digital comprehensive Summaries of Uppsala Dissertations from the Faculty of Science and Technology, 813.