Abstract
Urinary tract infections (UTIs) are among the most frequent infectious diseases affecting humans, and represent an important public health problem with a substantial economic burden. Due to the high empiric use of antibiotics for the treatment of UTI, antibacterial resistance of Enterobacteriaceae, specifically the main uropathogens Escherichia coli and Klebsiella pneumoniae, has significantly increased worldwide. In this article the worldwide epidemiology of resistant Gram-negative bacteria causing UTIs, with a special focus on extended spectrum beta lactamase (ESBL) positive pathogens, as well as new threats such as multi-drug-resistant (MDR) clones (e.g. E. coli 131 (ST131) and K. pneumoniae ST258), are reviewed. The increased prevalence of MDR Enterobacteriaceae, limiting available treatment options for infections caused by these organisms, and the lack of new antibiotics provide good rationale for using older antibiotics, such as fosfomycin, that have been shown to retain some activity against MDR bacteria.
Introduction
Urinary tract infections (UTIs) are among the most frequent infectious diseases affecting humans, and represent an important public health problem with a substantial economic burden. In the US, UTIs are responsible for >7 million physician visits annually and 15% of all community-prescribed antibiotics, with similar figures in some European countries.Citation1
UTIs are primarily caused by Gram-negative bacteria. The main pathogen responsible for uncomplicated cystitis and pyelonephritis is Escherichia coli followed by other species of Enterobacteriaceae, such as Proteus mirabilis and mostly Klebsiella pneumoniae, and by Gram-positive pathogens, such as Enterococcus faecalis and Staphylococcus saprophyticus.Citation2–3 International guidelines for the treatment of uncomplicated UTIs and pyelonephritis recommend various agents, such as nitrofurantoin monohydrate, trimethoprim-sulfamethoxazole, fosfomycin trometamol, pivmecillinam, fluoroquinolones and beta-lactams.Citation1,3 However, there is an alarming level of antimicrobial resistance developing in UTI pathogens as a result of indiscriminate and widespread use of antibiotics. Bacteria producing extended spectrum beta-lactamases (ESBLs), showing resistance to most antibiotics except for the carbapenem group, are steadily increasing in the population.Citation1,4
Clinicians treating these infections caused by drug-resistant pathogens have few effective antimicrobials to choose from and many are associated with significant adverse effects. There are now multi-, and often pan-resistant organisms that could be encountered in clinical practice. Therefore, it is crucial that the spread of resistance be accurately monitored and controlled.Citation5
Extended spectrum beta-lactamases (ESBLs)
ESBLs are enzymes able to hydrolyze third-and fourth generation cephalosporins and monobactams, but not cephamycins or carbapenems. They are inhibited by clavulanic acid (sulbactam or tazobactam), the genetic determinants are generally associated with plasmids but often cause co-resistance (aminoglyclosides, quinolones). ESBLs represent a public health concern, due to the high prevalence in E. coli in the community, and have a variable prevalence in K. pneumoniae (higher in hospitals). Table includes a classification of ‘classical’ ESBLs and Figure shows the trend of variants of different beta-lactamase families.
Table 1 Classification of ‘classical’ extended spectrum beta-lactamases (ESBLs)
In past years, CTX-M enzymes have become the most prevalent ESBLs in both nosocomial and community settings.Citation6 These are molecular class-A ESBLs, inhibited by clavulanic acid, with higher hydrolytic activity against cefotaxime than ceftazidime (but new variants also hydrolyze ceftazidime) and genetically related to chromosomal β-lactamases from bacteria of the Kluyvera genus. At least five different phylogenetic groups (≤90%) are known. The first CTX-M was identified in 1989 in E. coli isolated from the ear exudate of a newborn, and was highly resistant to cefotaxime and the majority of beta-lactam antibiotics.Citation7 Simultaneously, Salmonella typhimurium strains resistant to most beta-lactams, co-trimoxazole, tobramycin and gentamicin were isolated in Argentina, carrying a cefotaximase different from that isolated in E. coli, and named CTX-M-2.Citation8
The epidemiology of ESBLs is changing (Table ). It is noteworthy that in the two last decades of the past century, ESBL-positive strains were confined to the hospital setting, where they were responsible for nosocomial outbreaks, but these strains have become prevalent in infected outpatients since 2000. The prevalence of ESBLs, especially CTX-M, is increasing worldwide, with significant differences between geographic areas, again due to extensive and often indiscriminate use of antibiotics.
Table 2 Changing epidemiology of ESBLs
Epidemiology of resistant Gram-negative bacteria causing UTIs
There is no general overview of ESBL in UTIs, but results from bloodstream and abdominal infections give an idea of the different prevalence of these enzymes throughout Europe.
According to the European Antimicrobial Resistance Surveillance Network (EARS-Net), the main European Union (EU) surveillance system for multi-drug-resistant pathogens isolated in bloodstream infections and collected in 28 EU member states plus two European Economic Area (EEA) countries (Iceland and Norway), the resistance of E. coli and K. pneumonia to most common antibacterial classes used in clinical practice is high particularly in some countries, where these drugs can no more be empirically used for the treatments of UTIs. For example, in Italy in 2014, rates of antibiotic resistance to aminopenicillins, aminoglycosides and fluoroquinolones in E. coli were 65, 19 and 44%, and rates of antibiotic resistance to aminoglycosides and fluoroquinolones in K. pneumonia were 49% and 56%.Citation9
As far as ESBL-positive E. coli strains are concerned, national rates of resistant isolates in 2014 ranged from 3.3% (Iceland) to 40.4% (Bulgaria) (Figure , Table ). It is noteworthy that most countries with resistance percentages of ≥25% were located in South and East Europe, with a clear gradient from North to South. In fact, rates of resistant isolates in Northern European countries was lower than 10%, but ranged from 12 to 30% in Mediterranean countries (with even higher rates in Eastern Europe). Between 2011 and 2014, increasing percentages of ESBL-positive E. coli were observed in 12 countries (Belgium, Bulgaria, the Czech Republic, France, Germany, Greece, Ireland, Italy, Norway, Portugal, Slovenia and Sweden). The same increasing trend was seen for the overall EU/EEA population rate (from 9.6% in 2011 to 12.0% in 2014).Citation9
Figure 2 Escherichia coli. Percentage of invasive isolates with resistance to third-generation cephalosporins, by country, EU/EEA countries, 2014Citation9.
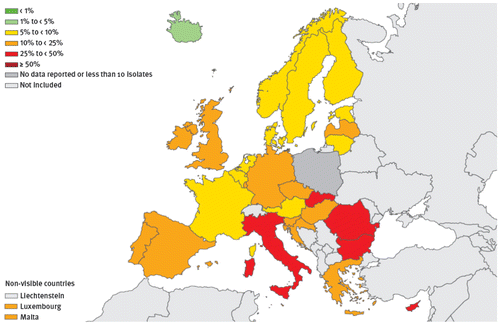
Table 3 Percentage of ESBL positive E. coli and K. pneumoniae isolates in the EU/EEA Countries, 2014Citation9
Findings are similar for ESBL-positive K. pneumoniae strains. The percentage of resistant isolates in 2014 varied widely (from zero in Iceland to 74.8% in Bulgaria), with a significant increasing trend between 2011 and 2014 seen in 11 countries (the Czech Republic, France, Ireland, Italy, Malta, Norway, Portugal, Romania, Spain, Sweden and the United Kingdom) and overall (from 23.6% in 2011 to 28.0% in 2014) (Table , Figure ). A North–South gradient was also present, because most Northern European countries had a rate <10% and most Mediterranean countries had a higher rate, ranging from 18% in Spain to 73% in Greece). Similarly to E. coli, the increase of combined resistance to more antimicrobial classes and the high frequency of ESBL-producing isolates may lead to increased use of carbapenems, thus, favouring the further dissemination of carbapenemase-producing bacteria.Citation9
Figure 3 Klebsiella pneumoniae. Percentage of invasive isolates with resistance to third-generation cephalosporins, by country, EU/EEA countries, 2014Citation9.
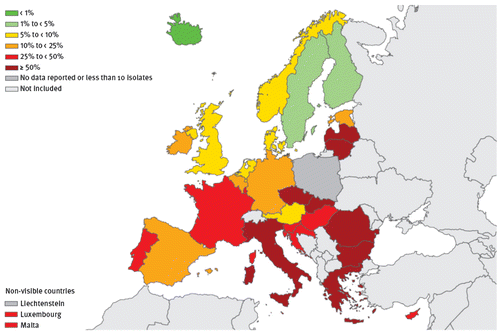
A study evaluated the ESBL rate in 3160 clinical strains of E. coli isolated from intra-abdominal infections from 13 European countries between 2008 and 2009. The average frequency of ESBL-producing strains was 11%, but this varied widely (from 1.4% in Estonia to 35% in Turkey) (Figure ).Citation10
Figure 4 Frequencies of ESBL-positive clinical isolates of E. coli in different countriesCitation10.
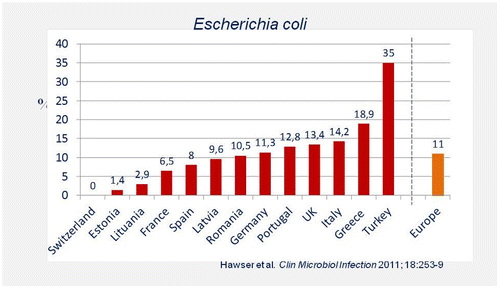
Outside Europe, ESBL diffusion is even higher, and prevalence is increasing everywhere, particularly in Asia, Latin America and the Middle East. Hawser et al. evaluated 2841 clinical isolates of K. pneumoniae from intra-abdominal infections worldwide collected in the Study for Monitoring Antimicrobial Resistance Trends (SMART) during 2008 and 2009. The overall rate of ESBL-positive strains was 22.4%, with the highest resistance rates observed in Latin America and Africa (Table ).Citation11 SMART data confirmed the same global rate of ESBL positivity in E. coli (23%), with the highest values in Asia and Latin America.
Table 4 Percentages of ESBL-positive K. pneumoniae isolates worldwide, 2011Citation11
The SMART study also evaluated the prevalence of ESBLs in Enterobacteriaceae (E. coli, K. pneumoniae, K. oxytoca and P. mirabilis) isolated from patients with UTIs between 2009 and 2011 (Figure ). In this case, Asia and the Middle East showed not only the highest ESBL prevalence, but also a significant increase from 2009 to 2011 (Middle East p < 0.0001, Asia p = 0.0002). In Europe, the average prevalence of ESBL-positive UTI pathogens was calculated to be 20%.Citation12 In all regions, with the partial exception of North America, molecular data show the absolute dominance of CTX-M, together with the emergence of carbapenemases.Citation11,13–15
Figure 5 Prevalence of ESBLs in E. coli, K. pneumonia, K. oxytoca and P. mirabilis from urinary tract infections by region from SMART 2009 to 2011Citation12.
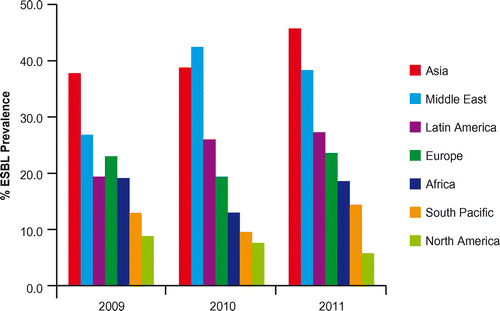
Most ESBL-positive Enterobacteriaceae are resistant to several antibiotics, such as fluoroquinolones, trimethoprim-sulfamethoxazole and beta-lactams, which are no longer suitable for the empiric treatment of some infections, including UTIs. One of the most active antibiotics against ESBL-positive UTI pathogens is fosfomycin trometamol. Data from a systematic review of ESBL-producing E. coli susceptibility to fosfomycin between 2010 and 2015 showed rates of 81% to 100% (Table ).Citation16, Citation17
Table 5 Susceptibility to fosfomycin of ESBL-producing E. coli strains (2010–2017) Adapted from Citation16,Citation17
A new threat: multi-drug-resistant (MDR) clones
ESBL diffusion is typically driven by international MDR high-risk clones. E. coli sequence type 131 (ST131) and K. pneumoniae ST258 are the quintessential examples of these clones. They emerged in the 2000s as important human pathogens, have spread extensively throughout the world, and are the main clones responsible for the rapid increase in antimicrobial resistance among E. coli and K. pneumoniae strains, respectively. E. coli ST131 is often fluoroquinolone-resistant and associated with ESBL production, especially CTX-M-15. K. pneumoniae ST258 is associated with carbapenemases, most often KPC-2 and KPC-3. Epidemic plasmids with blaCTX-M and blaKPC have contributed significantly to the success of these clones.Citation44
Virulence determinants also appear to play a pivotal role in ST131 success among bacteria responsible for UTIs, as shown in animal modelsCitation45,46 and recently demonstrated in 16 CTX-M-15 producing uropathogenic ST131 E. coli isolates recovered in India. Several adhesins, toxins, siderophores and protectins are produced by these isolates.Citation47
ESBL-bearing strains seem particularly prone to harbouring other resistance genes, leading to multi-drug resistance. This is particularly the case for ST131,Citation48 for which an increase in fosfomycin resistance in urinary isolates has been reported in Spain to parallel fosfomycin consumption.Citation49 Another issue with ST131, and a further problem in UTI therapy, seems to be production of all types of carbapenemases by these strains.
Klebsiella pneumoniae from hospitals to outpatients
K. pneumoniae is a leading cause of nosocomial infections worldwide, causing pneumonia, bloodstream infections, UTIs, surgical site or wound infections and meningitis. It is among the most common multi-resistant bacteria causing healthcare-associated infectionsCitation50 and has significant epidemic potential, often being responsible for serious infections. In recent years, K. pneumoniae has shown its ability to acquire plasmids encoding for ESBLs, secondary to the abuse of wide-spectrum cephalosporins in hospitals, but infections caused by this resistant phenotype also spread in the community.Citation51 The first plasmidic ESBL in K. pneumoniae was identified in Germany at beginning of 1980 and derived from SHV-1.Citation52 Until the end of 1990s, K. pneumonia-producing ESBLs were found in hospital settings only and derived from TEM to SHV. From 2000 onwards, the epidemiology changed with the diffusion of CTX-M ESBLs in K. pneumoniae.Citation51
Sporadic cases of K. pneumoniae with CTX-M-15 were initially reported from various European countries such as Great Britain, Italy and Spain,Citation53–55 but increasing percentages were soon reported worldwide, as shown by data presented in various multicentre studies.Citation56–58 The mobility of genetic elements, in particular those conferring antibiotic resistance, together with clonal expansion contribute to the persistence of these strains in hospitals and in the community.Citation56,58 There are a few reports of K. pneumoniae strains with chromosomally integrated CTX-M-15 at an undetermined locus, which were typed as ST1 in a Spanish study.Citation59 Recently, a site of integration of a complete CTX-M-15/ISEcp1 element into the chromosome of a K. pneumoniae ST101 strainCitation60 was identified in Germany.
Susceptibilities to fosfomycin between 2010 and 2015 were reported in a recent systematic review – ESBL-producing K. pneumoniae susceptibility to fosfomycin ranged from 40 to 97.5% (Table ).
Table 6 Susceptibility to fosfomycin of ESBL-producing K. pneumoniae strains (2010–2015)Citation16
It was concluded that, on the grounds of local susceptibility data, fosfomycin trometamol could be considered for the treatment of patients with infections due to problematic MDR bacteria.Citation16
Conclusions
The increasing prevalence of MDR Enterobacteriaceae limits available treatment options for infections caused by these organisms. Given the lack of new antibiotics, there is a valid rationale for testing older antibiotics that retain some activity against MDR bacteria.
Of these, fosfomycin trometamol appears to be a possible option because of its broad spectrum of activity against both Gram-positive and Gram-negative bacteria. Microbiological data appear to support this possibility, and susceptibility percentages are very high in K. pneumoniae and, mostly, in E. coli.
Conflict of interest
The authors state that they have no conflicts of interest to declare.
Funding
No external funding was obtained for this article; all authors take full responsibility for the content.
Notes on contributors
Annarita Mazzariol earned her PhD in Basic Microbiology at the University of Verona. She is currently an associate professor in the same University and is responsible for the MDR screening unit at the joint Clinical Microbiology Laboratories of Verona. Her main areas of interest is bacterial resistance mechanisms, with special focus on beta-lactamases.
Alda Bazaj has a masters in Bioinformatics and Medical Biotechnologies from the University of Verona, Italy. She attended the Department of Microbiology of the Peking University Medical Hospital, (with a scholarship from the European Society of Clinical Microbiology and Infectious Diseases) and the Unité des Rickettsies, Faculté de médecine, Marseille (with a grant from the Université de la Meditérranée), She has now started her PhD course in Applied Life and Health Sciences at the University of Verona.
Giuseppe Cornaglia obtained a degree in Medicine and Surgery from the University of Sassari, Italy. He is a full professor of Microbiology in the University of Verona and leads the joint Clinical Microbiology laboratories of Verona. He has been President of the European Society of Clinical Microbiology and Infectious Diseases, ESCMID (2007–2009 and 2010–2012) and is currently the ESCMID Director for International Affairs and the Chairman of the ESCMID Study Group for Antimicrobial Resistance Surveillance (ESGARS). A specialist in antimicrobial resistance epidemiology and related research, he received an Honorary Degree in Public Health from the St. Elizabeth University of Bratislava (Slovakia) for his ‘outstanding contribution to Public Health in the field of antimicrobial resistance research in Eastern Europe’.
References
- Bonkat G, Pickard R, Bartoletti R, Bruyère F, Cai T, Geerlings SE, et al. Guidelines on urological infections. EAU. 2017.
- Flores-Mireles AL, Walker JN, Caparon M, Hultgren SJ. Urinary tract infections: epidemiology, mechanisms of infection and treatment options. Nat Rev Microbiol. 2015;13(5):269–84.10.1038/nrmicro3432
- Gupta K, Hooton TM, Naber KG, Wullt B, Colgan R, Miller LG, et al. Infectious Diseases Society of America; European Society for Microbiology and Infectious Diseases. International clinical practice guidelines for the treatment of acute uncomplicated cystitis and pyelonephritis in women: a 2010 update by the Infectious Diseases Society of America and the European Society for Microbiology and Infectious Diseases. Clin Infect Dis. 2011;52(5):e103–2.10.1093/cid/ciq257
- Oteo J, Pérez-Vázquez M, Campos J. Extended-spectrum [beta]-lactamase producing Escherichia coli: changing epidemiology and clinical impact. Curr Opin Infect Dis. 2010;23(4):320–6.10.1097/QCO.0b013e3283398dc1
- Boyle DP, Zembower TR. Epidemiology and management of emerging drug-resistant Gram-negative bacteria: extended-spectrum β-lactamases and beyond. Urol Clin North Am. 2015;42(4):493–505.10.1016/j.ucl.2015.05.005
- Cantón R, Coque TM. The CTX-M beta-lactamase pandemic. Curr Opin Microbiol. 2006;9(5):466–75.10.1016/j.mib.2006.08.011
- Bauernfeind A, Grimm H, Schweighart S. A new plasmidic cefotaximase in a clinical isolate of Escherichia coli. Infection. 1990;18(5):294–8.10.1007/BF01647010
- Bauernfeind A, Casellas JM, Goldberg M, Holley M, Jungwirth R, Mangold P, et al. A new plasmidic cefotaximase from patients infected with Salmonella typhimurium. Infection. 1992;20(3):158–63.10.1007/BF01704610
- European Centre for Disease Prevention and Control. Antimicrobial resistance surveillance in Europe 2014. Annual Report of the European Antimicrobial Resistance Surveillance Network (EARS-Net). Stockholm: ECDC; 2014. p. 2015.
- Hawser SP, Bouchillon SK, Lascols C, Hackel M, Hoban DJ, Badal RE, et al. Susceptibility of European Escherichia coli clinical isolates from intra-abdominal infections, extended-spectrum β-lactamase occurrence, resistance distribution, and molecular characterization of ertapenem-resistant isolates (SMART 2008–2009). Clin Microbiol Infect. 2012;18(3):253–9.10.1111/j.1469-0691.2011.03550.x
- Hawser SP, Bouchillon SK, Lascols C, Hackel M, Hoban DJ, Badal RE, et al. Susceptibility of Klebsiella pneumoniae Isolates from Intra-Abdominal Infections and Molecular Characterization of Ertapenem-Resistant Isolates. Antimicrob Agents Chemother. 2011;55(8):3917–21.10.1128/AAC.00070-11
- Morrissey I, Hackel M, Badal R, Bouchillon S, Hawser S, Biedenbach D. A review of ten years of the study for monitoring antimicrobial resistance trends (SMART) from 2002 to 2011. Pharmaceuticals (Basel). 2013;6(11):1335–46.10.3390/ph6111335
- Lascols C, Hackel M, Marshall SH, Hujer AM, Bouchillon S, Badal R, et al. Increasing prevalence and dissemination of NDM-1 metallo-β-lactamase in India: data from the SMART study (2009). J Antimicrob Chemother. 2011;66(9):1992–7.10.1093/jac/dkr240
- Lascols C, Hackel M, Hujer AM, Marshall SH, Bouchillon SK, Hoban DJ, et al. Using nucleic acid microarrays to perform molecular epidemiology and detect novel β-lactamases: a snapshot of extended-spectrum β-lactamases throughout the world. J Clin Microbiol. 2012;50(5):1632–9.10.1128/JCM.06115-11
- Sheng WH, Badal RE, Hsueh PR, SMART ProGram. Distribution of extended-spectrum β-lactamases, AmpC β-lactamases, and carbapenemases among Enterobacteriaceae isolates causing intra-abdominal infections in the Asia-Pacific region: results of the study for Monitoring Antimicrobial Resistance Trends (SMART). Antimicrob Agents Chemother. 2013;57(7):2981–8.10.1128/AAC.00971-12
- Vardakas KZ, Legakis NJ, Triarides N, Falagas ME. Susceptibility of contemporary isolates to fosfomycin: a systematic review of the literature. Int J Antimicrob Agents. 2016;47(4):269–85.10.1016/j.ijantimicag.2016.02.001
- Mezzatesta ML, La Rosa G, Maugeri G, Zingali T, Caio C, Novelli A, et al. In vitro activity of fosfomycin trometamol and other oral antibiotics against multidrug-resistant uropathogens. Int J Antimicrob Agents. 2017;49(6):763–6.10.1016/j.ijantimicag.2017.01.020
- Cho YH, Jung SI, Chung HS, Yu HS, Hwang EC, Kim SO, et al. Antimicrobial susceptibilities of extended-spectrum beta-lactamase-producing Escherichia coli and Klebsiella pneumoniae in health care-associated urinary tract infection: focus on susceptibility to fosfomycin. Int Urol Nephrol. 2015;47(7):1059–66.10.1007/s11255-015-1018-9
- Sultan A, Rizvi M, Khan F, Sami H, Shukla I, Khan HM. Increasing antimicrobial resistance among uropathogens: Is fosfomycin the answer? Urol Ann. 2015;7(1):26–30.10.4103/0974-7796.148585
- Khan IU, Mirza IA, Ikram A, Ali S, Hussain A, Ghafoor T. In vitro activity of fosfomycin tromethamine against extended spectrum beta-lactamase producing urinary tract bacteria. J Coll Physicians Surg Pak. 2014;24(12):914–917.
- Cagan Aktas S, Gencer S, Batırel A, Hacıseyitoglu D, Ozer S. Fosfomycin susceptibility of urinary Escherichia coli isolates producing extended-spectrum beta-lactamase according to CLSI and EUCAST recommendations. Mikrobiyol Bul. 2014;48(4):545–555. [ in Turkish].
- Sorlozano A, Jimenez-Pacheco A, de Dios Luna Del Castillo J, Sampedro A, Martinez-Brocal A, Miranda-Casas C, et al. Evolution of the resistance to antibiotics of bacteria involved in urinary tract infections: a 7-year surveillance study. Am J Infect Control. 2014;42(10):1033–8.10.1016/j.ajic.2014.06.013
- Kresken M, Pfeifer Y, Hafner D, Wresch R, Korber-Irrgang B. Occurrence of multidrug resistance to oral antibiotics among Escherichia coli urine isolates from outpatient departments in Germany: extended-spectrum beta-lactamases and the role of fosfomycin. Int J Antimicrob Agents. 2014;44(4):295–300.10.1016/j.ijantimicag.2014.05.020
- Seo MR, Kim SJ, Kim Y, Kim J, Choi TY, Kang JO, et al. Susceptibility of Escherichia coli from community-acquired urinary tract infection to fosfomycin, nitrofurantoin, and temocillin in Korea. J Korean Med Sci. 2014;29(8):1178–1181.10.3346/jkms.2014.29.8.1178
- Villar HE, Jugo MB, Macan A, Visser M, Hidalgo M, Maccallini GC. Frequency and antibiotic susceptibility patterns of urinary pathogens in male outpatients in Argentina. J Infect Dev Ctries. 2014;8(6):699–704.
- Gupta V, Rani H, Singla N, Kaistha N, Chander J. Determination of extended-spectrum beta-lactamases and AmpC production in uropathogenic isolates of Escherichia coli and susceptibility to fosfomycin. J Lab Physicians. 2013;5(2):90–93.10.4103/0974-2727.119849
- Lai B, Zheng B, Li Y, Zhu S, Tong Z. In vitro susceptibility of Escherichia coli strains isolated from urine samples obtained in mainland China to fosfomycin trometamol and other antibiotics: a 9-year surveillance study (2004–2012). BMC Infect Dis. 2014;14:66.10.1186/1471-2334-14-66
- Qiao LD, Chen S, Yang Y, Zhang K, Zheng B, Guo HF, et al. Characteristics of urinary tract infection pathogens and their in vitro susceptibility to antimicrobial agents in China: data from a multicenter study. BMJ Open. 2013;3(12):e004152.10.1136/bmjopen-2013-004152
- Karlowsky JA, Denisuik AJ, Lagace-Wiens PR, Adam HJ, Baxter MR, Hoban DJ, et al. In vitro activity of fosfomycin against Escherichia coli isolated from patients with urinary tract infections in Canada as part of the CANWARD surveillance study. Antimicrob Agents Chemother. 2014;58(2):1252–6.10.1128/AAC.02399-13
- Demir T, Buyukguclu T. Evaluation of the in vitro activity of fosfomycin tromethamine against Gram-negative bacterial strains recovered from community- and hospital-acquired urinary tract infections in Turkey. Int J Infect Dis. 2013;17(11):e966–70.10.1016/j.ijid.2013.04.005
- Sahni RD, Balaji V, Varghese R, John J, Tansarli GS, Falagas ME. Evaluation of fosfomycin activity against uropathogens in a fosfomycin-naive population in South India: a prospective study. Future Microbiol. 2013;8(5):675–80.10.2217/fmb.13.31
- Rodríguez-Avial C, Rodríguez-Avial I, Hernández E, Picazo JJ. Increasing prevalence of fosfomycin resistance in extended-spectrum-beta-lactamase-producing Escherichia coli urinary isolates (2005–2009–2011). Rev Esp Quimioter. 2013;26(1):43–46. [in Spanish].
- Fournier D, Chirouze C, Leroy J, Cholley P, Talon D, Plésiat P, et al. Alternatives to carbapenems in ESBL-producing Escherichia coli infections. Med Mal Infect. 2013;43(2):62–6.10.1016/j.medmal.2013.01.006
- Bonkat G, Muller G, Braissant O, Frei R, Tschudin-Suter S, Rieken M, et al. Increasing prevalence of ciprofloxacin resistance in extended-spectrum-beta-lactamase-producing Escherichia coli urinary isolates. World J Urol. 2013;31:1427–32.10.1007/s00345-013-1031-5
- Araj GF, Jaber FA. In vitro activity of fosfomycin and other antimicrobials against uropathogenic Escherichia coli and Klebsiella pneumoniae at a tertiary care center in Lebanon. J Med Liban. 2012;60(3):142–147.
- Briongos-Figuero LS, Gómez-Traveso T, Bachiller-Luque P, Domínguez-Gil González M, Gómez-Nieto A, Palacios-Martín T, et al. Epidemiology, risk factors and comorbidity for urinary tract infections caused by extended-spectrum beta-lactamase (ESBL)-producing enterobacteria. Int J Clin Pract. 2012;66(9):891–6.10.1111/ijcp.2012.66.issue-9
- Meier S, Weber R, Zbinden R, Ruef C, Hasse B. Extended-spectrum beta-lactamase-producing Gram-negative pathogens in community-acquired urinary tract infections: an increasing challenge for antimicrobial therapy. Infection. 2011;39(4):333–40.10.1007/s15010-011-0132-6
- Liu HY, Lin HC, Lin YC, Yu SH, Wu WH, Lee YJ. Antimicrobial susceptibilities of urinary extended-spectrum beta-lactamase-producing Escherichia coli and Klebsiella pneumoniae to fosfomycin and nitrofurantoin in a teaching hospital in Taiwan. J Microbiol Immunol Infect. 2011;44(5):364–8.10.1016/j.jmii.2010.08.012
- den Heijer CD, Donker GA, Maes J, Stobberingh EE. Antibiotic susceptibility of unselected uropathogenic Escherichia coli from female Dutch general practice patients: a comparison of two surveys with a 5 year interval. J Antimicrob Chemother. 2010;65(10):2128–33.10.1093/jac/dkq286
- Auer S, Wojna A, Hell M. Oral treatment options for ambulatory patients with urinary tract infections caused by extended-spectrum-beta-lactamase-producing Escherichia coli. Antimicrob Agents Chemother. 2010;54(9):4006–8.10.1128/AAC.01760-09
- Wachino J, Yamane K, Suzuki S, Kimura K, Arakawa Y. Prevalence of Fosfomycin Resistance among CTX-M-Producing Escherichia coli Clinical Isolates in Japan and Identification of Novel Plasmid-Mediated Fosfomycin-Modifying Enzymes. Antimicrob Agents Chemother. 2010;54(7):3061–4.10.1128/AAC.01834-09
- Tena D, González-Praetorius A, González JC, Heredero E, Illescas S, de Baranda CS, et al. Changes in the antimicrobial susceptibility of Escherichia coli isolates from community diagnosed urinary tract infections during the period 2003–2007. Multicentre study in Castilla la Mancha (Spain). Rev Esp Quimioter. 2010;23(1):36–42 . [in Spanish].
- Chislett RJ, White G, Hills T, Turner DP. Fosfomycin susceptibility among extended-spectrum-beta-lactamase-producing Escherichia coli in Nottingham UK. J Antimicrob Chemother. 2010;65(5):1076–7.10.1093/jac/dkq051
- Mathers AJ, Peirano G, Pitout JD. The Role of epidemic resistance plasmids and international high-risk clones in the spread of multidrug-resistant Enterobacteriaceae. Clin Microbiol Rev. 2015;28(3):565–91.10.1128/CMR.00116-14
- Clermont O, Lavollay M, Vimont S, Deschamps C, Forestier C, Branger C, et al. The CTX-M-15-producing Escherichia coli diffusing clone belongs to a highly virulent B2 phylogenetic subgroup. J Antimicrob Chemother. 2008;61(5):1024–8.10.1093/jac/dkn084
- Lavigne JP, Vergunst AC, Goret L, Sotto A, Combescure C, Blanco J, et al. Virulence potential and genomic mapping of the worldwide clone Escherichia coli ST131. PLoS ONE. 2012;7(3):e34294.10.1371/journal.pone.0034294
- Hussain A, Ewers C, Nandanwar N, Guenther S, Jadhav S, Wieler LH, et al. Multiresistant uropathogenic Escherichia coli from a region in India where urinary tract infections are endemic: genotypic and phenotypic characteristics of sequence type 131 isolates of the CTX-M-15 extended-spectrum-β-lactamase-producing lineage. Antimicrob Agents Chemother. 2012;56(12):6358–65.10.1128/AAC.01099-12
- López-Cerero L, Navarro MD, Bellido M, Martín-Peña A, Viñas L, Cisneros JM, et al. Escherichia coli belonging to the worldwide emerging epidemic clonal group O25b/ST131: risk factors and clinical implications. J Antimicrob Chemother. 2014;69(3):809–14.10.1093/jac/dkt405
- Oteo J, Orden B, Bautista V, Cuevas O, Arroyo M, Martínez-Ruiz R, et al. CTX-M-15-producing urinary Escherichia coli O25b-ST131-phylogroup B2 has acquired resistance to fosfomycin. J Antimicrob Chemother. 2009;64(4):712–7.10.1093/jac/dkp288
- Peleg AY, Hooper DC. Hospital-acquired infections due to Gram-negative bacteria. N Engl J Med. 2010;362(19):1804–13.10.1056/NEJMra0904124
- Carrër A, Nordmann P. CTX-M-15-producing Klebsiella pneumoniae: a change in the epidemiology of ESBL. Pathol Biol (Paris). 2011;59(6):e133–5.
- Knothe H, Shah P, Krcmery V, Antal M, Mitsuhashi S. Transferable resistance to cefotaxime, cefoxitin, cefamandole and cefuroxime in clinical isolates of Klebsiella pneumoniae and Serratia marcescens. Infection. 1983;11(6):315–7.10.1007/BF01641355
- Livermore DM, Hawkey PM. CTX-M changing the face of ESBLs in the UK. J Antimicrob Chemother. 2005;56(3):451–4.10.1093/jac/dki239
- Mugnaioli C, Luzzaro F, De Luca F, Brigante G, Perilli M, Amicosante G, et al. CTX-M type ESBL in Italy: molecular epidemiology of an emerging countryside problem. Antimicrob Agents Chemother. 2006;50(8):2700–6.10.1128/AAC.00068-06
- Hernández JR, Martínez-Martínez L, Cantón R, Coque TM, Pascual A, Spanish Group for Nosocomial Infections (GEIH). Nationwide study of E. coli and K. pneumoniae producing ESBL in Spain. Antimicrob Agents Chemother. 2005:49(5):2122–2215.
- Poirel L, Bonnin RA, Nordmann P. Genetic support and diversity of acquired extended-spectrum β-lactamases in Gram-negative rods. Infect Genet Evol. 2012;12(5):883–93.10.1016/j.meegid.2012.02.008
- Younes A, Hamouda A, Dave J, Amyes S. Prevalence of transferable blaCTX-M-15 from hospital-and community-acquired Klebsiella pneumoniae isolates in Scotland. J Antimicrob Chemother. 2011;66(2):313–8.10.1093/jac/dkq453
- Coelho A, Mirelis B, Alonso-Tarrés C, Larrosa MN, Miró E, Abad RC, et al. Detection of three stable genetic clones of CTX-M-15-producing Klebsiella pneumoniae in the Barcelona metropolitan area Spain. J Antimicrob Chemother. 2009;64(4):862–4.10.1093/jac/dkp264
- Coelho A, González-López JJ, Miró E, Alonso-Tarrés C, Mirelis B, Larrosa MN, et al. Characterisation of the CTX-M-15-encoding gene in Klebsiella pneumoniae strains from the Barcelona metropolitan area: plasmid diversity and chromosomal integration. Int J Antimicrob Agents. 2010;36(1):73–8.10.1016/j.ijantimicag.2010.03.005
- Mshana SE, Fritzenwanker M, Falgenhauer L, Domann E, Hain T, Chakraborty T, et al. Molecular epidemiology and characterization of an outbreak causing Klebsiella pneumoniae clone carrying chromosomally located bla(CTX-M-15) at a German University-Hospital. BMC Microbiol. 2015;15:122.10.1186/s12866-015-0460-2
- Alibi S, Ferjani A, Boukadida J. Molecular characterization of extended spectrum beta-lactamases produced by Klebsiella pneumoniae clinical strains from a Tunisian Hospital. Med Mal Infect. 2015;45(4):139–43.10.1016/j.medmal.2015.01.010
- Kopacz J, Mariano N, Colon-Urban R, Sychangco P, Wehbeh W, Segal-Maurer S, et al. Identification of extended-spectrum-beta-lactamase-positive Klebsiella pneumoniae urinary tract isolates harboring KPC and CTX-M beta-lactamases in non hospitalized patients. Antimicrob Agents Chemother. 2013;57(10):5166–9.10.1128/AAC.00043-13
- Morfín-Otero R, Mendoza-Olazarán S, Silva-Sánchez J, Rodríguez-Noriega E, Laca-Díaz J, Tinoco-Carrillo P, et al. Characterization of Enterobacteriaceae isolates obtained from a tertiary care hospital in Mexico, which produces extended-spectrum beta-lactamase. Microb Drug Resist. 2013;19(5):378–83.10.1089/mdr.2012.0263