Abstract
The study of hybridogenesis is expected to shed light on the role played by the so‐called ‘asexual reproduction’ in vertebrate evolution, since hybridogenesis, gynogenesis and parthenogenesis are restricted to lower vertebrates. Hence, it seemed interesting to define the stage and time of germline differentiation in reproductively unstable organisms which, to accomplish functional gamete maturation, can change the normal approach to meiosis by means of unusual cytological steps, such as genome elimination and endoreduplication. The cytogenetic aspects of ‘asexual’ meiosis have already been thoroughly studied. At present, molecular investigations are in progress to characterise the single steps of germline differentiation in a hybridogenetic green frog system, and the results obtained so far can be considered promising, as shown in this work. Vasa, PL10 and Y‐box homolog genes, first isolated in Rana (Pelophylax), are expressed during early phases of gametogenesis in R. (P.) ridibunda and R. (P.) lessonae (parental species), and R. (P.) esculenta (their natural hybrid), suggesting that gametogenesis, during the adult life, follows a unique genetic sequence in the two parental species as well as in hybridogenetic hybrids. Similar molecular investigations need to be carried out on larval stages prior to metamorphosis, when the genetic plasticity of gemline cells allows their commitment versus either the standard and/or hybridogenetic reproduction mode.
This paper is dedicated to Professors Giorgio Mancino and Stefania Bucci on the occasion of their retirement.
There must be a molecular basis for the differences in anatomy and way of life among organisms. (Wilson et al. Citation1974)
Rana esculenta, a fertile hybrid
Western Palearctic water frogs, also known as the Rana esculenta complex, represent an interesting biological mystery both at the taxonomic and reproductive level. Interspecific hybridisation takes place spontaneously in them, and certain hybrids can give rise to F1 (Berger Citation1968, Citation1988) or a peculiar form of R1 offspring (Mancino 2007). These hybrids possess a unique reproductive mode, referred as to hybridogenesis, built on peculiar cytological processes occurring in germ line cells (Schultz Citation1969; Tunner Citation1974). Such processes imply a pre‐meiotic exclusion of one of the two parental chromosome sets, followed by pre‐meiotic and/or meiotic endoreduplication of the retained set (Tunner & Heppich‐Tunner Citation1991). Somatic hybridity is restored with fertilisation by a gamete containing the excluded parental genome. The most widespread population system is the L–E one, in which the Rana esculenta (E) hybrid, arising from the natural cross between Rana ridibunda (R) and Rana lessonae (L), coexists with Rana lessonae.
The hybridogenetic constitution of R. esculenta has been confirmed both by genetic studies based on somatic and germ line gene dosage, and by cytogenetic analyses carried out on oocyte lampbrush chromosomes as well as spermatocyte bivalents (Uzzell Citation1982; Hotz & Uzzell Citation1982; Hotz Citation1983; Bucci et al. Citation1990; Mancino Citation1994; Beerli et al. Citation1996). However, the molecular mechanisms regulating the exclusion and endoreduplication, two distinct cytological processes that can occur autonomously in a temporally separated fashion, are still unknown (Mancino et al. Citation1987; Figure ). Some esculenta hybrids do not accomplish genome exclusion and show meiotic abnormalities. Accordingly, the reproductive capacity of these non‐hybridogenetic hybrids proves to be seriously compromised (Mancino et al. Citation1984; Hotz et al. Citation1985; Bucci et al. Citation1990; Guerrini et al. Citation1997). Haploid spermatogonia have been detected in R. esculenta hybrids of the L–E system (Heppich et al. Citation1982). More recently we observed that the co‐presence of haploid and diploid spermatogonial cells in R. esculenta adult hybrids derived from the crosses between L–E and R–E system frogs (Ragghianti et al. Citation2007; Figure ).
Figure 1 a, The haploid chromosome set of an oocyte I from a hybridogenetic R. esculenta specimen of the L–E system: the 13 univalents are identifiable as belonging to R. ridibunda lampbrush genome on the basis of specific cytogenetic markers: centromeres are always evident as loopless granules or bars and a few giant lateral structures are inserted along the chromosomes. The haploid set of 13 ridibunda univalents can be explained by assuming that this oocyte has excluded the lessonae genome without accomplishing the subsequent endoreduplication. b,c, Chromosome preparations of germinal tissues hybridised in situ with DIG‐labelled RrS1 as a probe. Spermatogonial metaphases (male specimen, cross 02.41) containing either 13 ridibunda chromosomes, 6 of which show RrS1‐marked centromeres (b) or 26 ridibunda chromosomes, 12 of which have RrS1‐marked centromeres (c). These findings reflect germ cells after the elimination of lessonae genome, but prior to the endoreduplication of the remaining ridibunda genome (b), and both the exclusion and subsequent endoreduplication of the ridibunda genome, respectively (c). Phase contrast (a); c = centromeres; S = sphere. Scale bar: 20 µm (a), 5 µm (b, c).
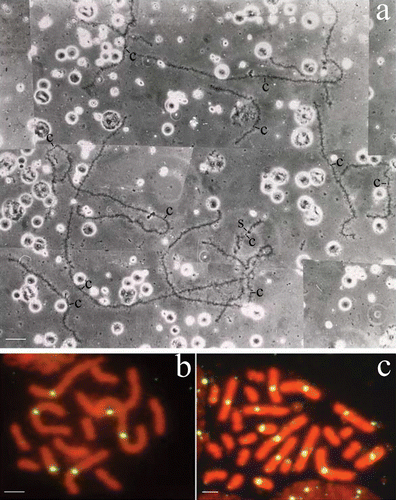
The evolutionary role of hybridogenesis is still a debated question. The hybridogenetic reproductive mode could be considered a device to overcome the interspecific isolation barriers usually affecting the meiotic process (Mancino et al. Citation1987). In line with this hypothesis, hybridogenesis can represent a tool that allows hybrids to reproduce hemiclonally.
Genome organisation of green frogs
In order to clarify the phylogenetic relationships within western Palearctic water frogs, we performed molecular investigations on the genome organisation of this amphibian group. The main goal of these studies was to assess the taxonomic rank reached by R. ridibunda during its speciation with respect to partially or totally sympatric species, such as R. lessonae living in central and central‐southern Europe, and Balkan species sharing the south‐eastern regions of the ridibunda habitat. Molecular analyses concerning genome organisation of the centromeric satellite RrS1 (Ragghianti et al. Citation1995, Citation1999, Citation2004) emphasised that: (1) the RrS1 family is specifically present in the genome of green frogs; (2) elements belonging to RrS1 DNA family are also present in Balkan species, and in the North‐African species R. saharica; (3) R. ridibunda and R. epeirotica are the species in which the molecular organisation of RrS1 sequences is mainly conservative.
Moreover, we characterised two dispersed families of repetitive DNA, named Rana/PolIII and RlBamH1. Rana/PolIII elements are tandemly arrayed SINE‐like retrotransposons scattered throughout the genome of western Palearctic water frogs (Bucci et al. Citation1999). RlBamH1 sequences, showing similarity to members of the hAT DNA transposon family, are highly conserved within the Rana genus, being present not only in green frogs but also in the brown frog R. dalmatina (Casola et al. Citation2004). Since transposition represents a significant evolutionary mechanism that may contribute to genome turnover as well as genetic variability (Lerat et al. Citation2003 and references therein), the evolutionary dynamics of the two repetitive DNA families could be related to differentiation events of the Rana genus.
Characterisation of Rana germ cell‐specific genes
Bearing in mind the complex reproductive mode adopted by interspecific hybrids inside the green frog system, we supposed that a molecular analysis of gametogenetic processes, both of the parental species and hybrids, might contribute to decoding the cellular and molecular events regulating hybridogenesis. We therefore focused our attention on highly conserved genes coding for post‐transcriptional regulators that play a key role in germ cell specification and differentiation, both in vertebrates and invertebrates.
By using a RT‐PCR strategy in Rana lessonae, we isolated RlVlg, RlPL10 and RlYB2 genes, the respective homologs of the Vasa, PL10 and Y‐box families. Vasa and PL10 encode two putative DEAD‐box ATP‐dependent RNA helicases involved in RNA binding and unwinding (Linder & Lasko Citation2006). Y‐box proteins are involved in masking the mRNAs stored in oocytes, by preventing them being translated (Sommerville Citation1999). RlVlg and RlYB2 were germline specifically expressed (Marracci et al. 2007a, Citation2008). During oogenesis, RlVlg, RlPL10 and RlYB2 transcripts were distributed throughout the cytoplasm of early oocytes (Figure ). RlVlg and RlPL10 were differentially expressed in different phases of mitotic and meiotic spermatogenetic divisions, as shown by immunostaining experiments with anti‐H3 phosphohistone antibody used as a mitotic and meiotic marker (Marracci et al. Citation2007; Figure ). The three genes showed similar expression patterns in the parental species and hybrid (Marracci et al. Citation2007, Citation2008).
Figure 2 Expression profile ofRlVlg (a), RlPL10 (b) and RlYB2 (c) during oogenesis of R. ridibunda (a), R. lessonae (b), and R. esculenta (c), by using whole‐mount (a, c) and on section (b) in situ hybridisations. Oocyte stages are indicated. Scale bars: 100 µm.
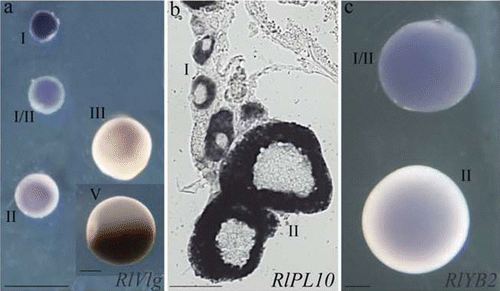
Figure 3 Expression pattern ofRlVlg (a, b) and RlPL10 (c, d) during spermatogenesis. Hybridised sections of adult testes of R. ridibunda (a, c) and R. esculenta (b, d) were immunostained with anti‐phosphohistone H3 antibody. RlVlg (blue) and phosphohistone H3 (red fluorescence) expression signals were not overlapping. The RlPL10 expression pattern (blue‐marked) included phosphohistone H3‐positive cells. SGI = primary spermatogonia, SGII = secondary spermatogonia, SCI = primary spermatocites, SCII = secondary spermatocytes, SD = spermatids, SZ = spermatozoa. Scale bar: 40 µm.
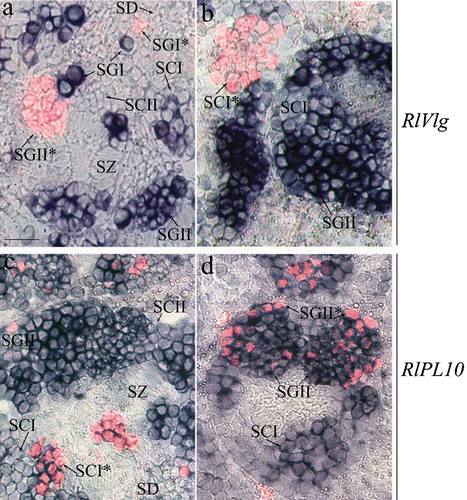
As in other organisms, RlVlg, RlPL10 and RlYB2 can be involved in regulating mRNAs translation during gametogenesis of R. ridibunda, R. lessonae, and R. esculenta, allowing a correct pathway for germ cell development and differentiation.
Conclusions and perspectives
During their life history inside the genus Rana, the real species R. ridibunda and R. lessonae have become highly differentiated with respect to morphological features and to ethological and ecological preferences. Despite the entity of the general morpho‐anatomical and functional differentiation, R. ridibunda and R. lessonae succeeded in overcoming the last reproductive isolation barrier at gamete level and producing gametes which maintained a considerable rate of cytological similarity during evolution. Then the two parental species carried out cross fertilisation, giving rise to the hybrid, R. esculenta, that, in turn, produced genetically balanced and functional gametes by following a modified gametogenetic process denoted as hybridogenesis. The occurrence of similar expression profiles of RlVlg, RlPL10 and RlYB2 in the parental species and their hybrid argues for the occurrence of similar basic mechanisms, that control gametogenesis and lead to the production of compatible gametes in R. ridibunda, R. lessonae and R. esculenta. These results support the hypothesis that green frog species appear closely related when the gametic and gametogenetic aspects are taken into consideration, while their specific morpho‐anatomical and physiological characters make them seem very differentiated.
Finally, RlVlg, RlPL10 and RlYB2 represent distinctive markers of male and female green frog germ cells. Since previous cytological studies have highlighted an altered development of germ cells of hybrid embryos, compared to those of the parental species (Ogielska & Wagner Citation1993), we consider these genes to be useful candidates to shed new light on the molecular basis of hybridogenetic events, namely exclusion and endoreduplication, which occur during pre‐metamorphic and metamorphic larval developmental stages.
Acknowledgements
This work was supported by Pisa University grants.
Notes
This paper is dedicated to Professors Giorgio Mancino and Stefania Bucci on the occasion of their retirement.
References
- Beerli , P. , Hotz , H. and Uzzell , T. 1996 . Geologically dated sea barriers calibrate a protein clock for Aegean water frogs. . Evolution , 50 : 1676 – 1687 .
- Berger , L. 1968 . Morphology of F1‐generation of various crosses within Rana esculenta‐complex. . Acta Zoologica Cracoviensia , 13 : 301 – 324 .
- Berger , L. 1988 . Principles of studies of European water frogs. . Acta Zoologica Cracoviensia , 31 : 563 – 580 .
- Bucci , S. , Ragghianti , M. , Mancino , G. , Berger , L. , Hotz , H. and Uzzell , T. 1990 . Lampbrush and mitotic chromosomes of the hemiclonally reproducing hybrid Rana esculenta and its parental species. . The Journal of Experimental Zoology , 255 : 37 – 56 .
- Bucci , S. , Ragghianti , M. , Mancino , G. , Petroni , G. , Guerrini , F. and Giampaoli , S. 1999 . Rana/Pol III: A family of SINE‐like sequences in the genomes of western Palearctic water frogs. . Genome , 42 : 504 – 511 .
- Casola , C. , Marracci , S. , Bucci , S. , Ragghianti , M. , Mancino , G. , Hotz , H. , Uzzell , T. and Guex , G. D. 2004 . A hAT‐related family of interspersed repetitive elements in genomes of western Palaearctic water frogs. . Journal of Zoological Systematics and Evolutionary Research , 42 : 234 – 244 .
- Guerrini , F. , Bucci , S. , Ragghianti , M. , Mancino , G. , Hotz , H. , Uzzell , T. and Berger , L. 1997 . Genomes of two water frog species resist germ line exclusion in interspecies hybrids. . The Journal of Experimental Zoology , 279 : 163 – 176 .
- Heppich , S. , Tunner , H. G. and Greilhuber , J. 1982 . Premeiotic chromosome doubling after genome elimination during spermatogenesis of the species hybrid Rana esculenta. . Theoretical and Applied Genetics , 61 : 101 – 104 .
- Hotz , H. 1983 . Genetic diversity among water frog genomes inherited with and without recombination PhD dissertation, Universität Zürich
- Hotz , H. and Uzzell , T. 1982 . Biochemical detected sympatry of two water frog species: Two different cases in the Adriatic Balkans (Amphibia: Ranidae). Proceedings. . Academy of Natural Sciences of Philadelphia , 134 : 50 – 79 .
- Hotz , H. , Mancino , G. , Bucci Innocenti , S. , Ragghianti , M. , Berger , L. and Uzzell , T. 1985 . Rana ridibunda varies geographically in inducing clonal gametogenesis in interspecies hybrids. . The Journal of Experimental Zoology , 236 : 199 – 210 .
- Lerat , E. , Rizzon , C. and Biémont , C. 2003 . Sequence divergence within transposable element families in the Drosophila melanogaster genome. . Genome Research , 13 : 1889 – 1896 .
- Linder , P. and Lasko , P. 2006 . Bent out of shape: RNA unwinding by the DEAD‐box helicase Vasa. . Cell , 125 : 219 – 221 .
- Mancino , G. 1994 . From conventional to molecular amphibian cytogenetics. . Animal Biology , 3 : 95 – 104 .
- Mancino , G. 2007 . Citogenetica degli Anfibi. 4 Citogenetica e Biologia riproduttiva , 67 – 78 . Bologna : Calderoni .
- Mancino , G. , Bucci , S. , Ragghianti , M. , Hotz , H. , Berger , L. and Uzzell , T. 1984 . Chromosomes, hybridization and hybridogenesis. . Acta Embryologiae et Morphologiae Experimentalis (‘Halocynthia Association’) , 5 : 209
- Marracci , S. , Casola , C. , Bucci , S. , Mancino , G. and Ragghianti , M. 2008 . Isolation and expression of RlYB2, a germ cell‐specific Y‐box gene in Rana. . The Italian Journal of Zoology , 75 : 1 – 9 .
- Marracci , S. , Casola , C. , Bucci , S. , Ragghianti , M. , Ogielska , M. and Mancino , G. 2007 . Differential expression of two vasa/PL10‐related genes during gametogenesis in the special model system Rana. . Development Genes Evolution , 217 : 395 – 402 .
- Mancino , G. , Ragghianti , M. , Bucci , S. , Hotz , H. , Berger , L. and Uzzell , T. 1987 . Cytogenetics of hibridogenesis in western Palearctic water frogs. . Acta Embryologiae et Morphologiae Experimentalis (‘Halocynthia Association’) , 8 : 131 – 135 .
- Ogielska , M. and Wagner , E. 1993 . Oogenesis and ovary development in the natural hybridogenetic water frog, Rana esculenta L. I Tadpole stages until metamorphosis. . Zoologische Jahrbücher (Physiologie) , 97 : 349 – 368 .
- Ragghianti , M. , Bucci , S. , Casola , C. , Marracci , S. and Mancino , G. 2004 . Molecular investigations in western Palearctic water frogs. . The Italian Journal of Zoology , 2 (Suppl.) : 17 – 23 .
- Ragghianti , M. , Bucci , S. , Guerrini , F. and Mancino , G. 1999 . Characterization of two repetitive DNA families (RrS1 and Rana Pol/III) in the genomes of Palearctic green water frogs. . The Italian Journal of Zoology , 66 : 255 – 263 .
- Ragghianti , M. , Bucci , S. , Marracci , S. , Casola , C. , Mancino , G. , Hotz , H. , Guex , G‐D. , Plötner , J. and Uzzell , T. 2007 . Gametogenesis of hybrids between two population systems of hemiclonally reproducing water frogs. . Genetical Research , 89 : 39 – 45 .
- Ragghianti , M. , Guerrini , F. , Bucci , S. , Mancino , G. , Hotz , H. , Uzzell , T. and Guex , G. D. 1995 . Molecular characterization of a centromeric satellite DNA in the hemiclonal hybrid frog Rana esculenta and its parental species. . Chromosome Research , 3 : 497 – 506 .
- Schultz , R. J. 1969 . Hybridization, unisexuality, and polyploidy in the teleost Poeciliopsis (Poeciliidae) and other vertebrates. . The American Naturalist , 103 : 605 – 619 .
- Sommerville , J. 1999 . Activities of cold‐shock domain proteins in translation control. . Bioessays , 21 : 319 – 325 .
- Tunner , H. G. 1974 . Die klonale Struktur einer Wasserfroschpopulation. Zeitschrift für Zoologische. . Systematik und Evolutionsforschung , 12 : 309 – 314 .
- Tunner , H. G. and Heppich‐Tunner , S. 1991 . Genome exclusion and two strategies of chromosome duplication in oogenesis of a hybrid frog. . Naturwissenschaften , 78 : 32 – 34 .
- Uzzell , T. 1982 . Immunological relationship of western Palearctic water frogs (Salientia: Ranidae). . Amphibia–Reptilia , 3 : 135 – 143 .
- Wilson , A. C. , Sarich , V. M. and Maxon , L. R. 1974 . The importance of gene rearrangement in evolution: Evidence from studies on rates of chromosomal, protein and anatomical evolution. . Proceedings of the National Academy of Sciences of the United States of America , 71 : 3028 – 3030 .