Abstract
Cork oak (Quercus suber L., 1753) is a Mediterranean evergreen tree species with a thick and characteristic bark covering the trunk and branches. Despite the fact that cork oak is distributed over about 1.7 million hectares in the western Mediterranean Basin, information about soil biota in this particular habitat at present is scanty. This study was carried out in a cork tree wood in Bergeggi (Liguria, NW Italy), characterized by a Pluvioseasonal Oceanic Mediterranean bioclimate. The profile, texture, pH, total carbonate content, total organic carbon (C) and nitrogen (N) contents, soluble phosphorus (P) and cation exchange capacity were measured in order to characterize the soil. Soil biological quality was evaluated by studying microarthropod communities. Each month, from February 2007 to January 2008, 10 soil samples measuring 10 × 10 × 10 cm were collected from the wood being studied and arthropods were extracted using Berlese-Tullgren funnels. The biological quality of soil based on arthropods (QBS-ar) index, taxa abundance, Shannon diversity index (H’) and Pielou’s evenness index (J) were applied to assess soil biological quality in the cork oak being studied.
A total of 29 taxonomic groups were identified. Some important groups, such as Pseudoscorpionida, Palpigradi, Miriapoda and Protura were detected every month, but only a few groups showed a clear abundance distribution trend throughout the year and globally it was impossible to define general trends. The results suggested that the condition of the soil was good, as generally observed in natural environments not subjected to significant impacts. It was also possible to demonstrate that the Mediterranean climate causes strong seasonal fluctuations in soil biota, resulting in corresponding differences in the QBS-ar index values. Therefore, the QBS-ar index could be considered to be a valid candidate for the biomonitoring of soil biodiversity in natural and anthropic soils, but in Mediterranean climates comparisons of different areas must be performed analyzing samples collected during the same period.
Introduction
Soil is one of the most species-rich habitats of terrestrial ecosystems (Wolters Citation2001) and the Mediterranean Region in particular is one of the biodiversity hotspots on the planet, reaching the highest peaks of diversity of soil-borne organisms (e.g. Blondel et al. Citation2010). Soil reflects ecosystem metabolism since all the bio-geo-chemical processes of the different ecosystem components are combined within it; therefore soil quality fluctuations are considered to be a suitable criterion for evaluating the long-term sustainability of ecosystems. Soil biota are key players in several supporting and regulating ecosystem services (Jeffery et al. Citation2010). Furthermore, soil fauna is often used to provide soil quality indicators (Cortet et al. Citation1999; Van Straalen Citation2004; Parisi et al. Citation2005; Tabaglio et al. Citation2009; Menta et al. Citation2011; Cluzeau et al. Citation2012; Testi et al. Citation2012; Visioli et al. Citation2013; Arredondo et al. in press). Soil organisms may be useful in monitoring environmental changes because they provide objective metrics that integrate physical, chemical and biological parameters (Blakely et al. Citation2002). Therefore, a number of authors have proposed new methods for soil quality assessment based on soil fauna, some of them based on a general evaluation of microarthropods (Parisi et al. Citation2005), others on the evaluation of a single taxon (Bernini et al. Citation1995; Paoletti & Hassal Citation1999; Paoletti & Hassal Citation1999; Parisi & Menta Citation2008). Most of the studies on the use of microarthropod communities as a tool to asses soil quality have focused on agroecosystems or polluted or degraded sites (Frampton Citation1997; Ruf Citation1998; Villenave et al. Citation2001; Blakely et al. Citation2002; Gardi et al. Citation2002; Huhta Citation2002; Tabaglio et al. Citation2008, Citation2009; Aspetti et al. Citation2010; Hartley et al. Citation2012; Santorufo et al. Citation2012), but recently Blasi et al. (Citation2013) analyzed 11 Mediterranean forest areas in Central Italy using the biological quality of soil based on arthropods (QBS-ar) index (Parisi et al. Citation2005) to assess the impact of different disturbances on soil quality. Despite the notable amount of literature on the use of this index published in recent decades (Parisi et al. Citation2005; Gardi et al. Citation2008; Tabaglio et al. Citation2009; Menta et al. Citation2011), no study has focused on the impact of the climatic factors on the QBS-ar in Mediterranean area. In our opinion, further research is necessary on such ecosystems, affected by dry summers, to evaluate the influence of seasonality on the soil microarthropod community and to assess the utility and the best sampling periods of some indices such as the QBS-ar and others. In particular, the QBS-ar index is based on soil microarthropods that, considering the soil fauna, are among the most abundant and diversified groups (Hishi et al. Citation2008). Environmental factors, such as water content and organic matter, affect soil microarthropod abundance and diversity (Takeda Citation1987; Klironomos & Kendrick Citation1995). Summer drought has been shown to alter the distribution and the abundance of both plants and their above-ground invertebrate herbivores (Buckland et al. Citation1997; Masters et al. Citation1998; Stenberg Citation2000), whereas it has been suggested that soil-dwelling invertebrates may be less responsive to the direct impacts of climate changes than their above-ground counterparts (Bale et al. Citation2002; Richardson et al. Citation2002) and their response to an increased incidence of summer droughts is therefore likely to vary, depending on feeding strategies and life history (Staley et al. Citation2007). However, since reduced soil moisture can have a strong impact on soil fauna’s feeding and ovoposition behaviour, survival and abundance (Anderson Citation1987; Brown & Gange Citation1990; Setälä et al. Citation1995), the indirect impact of changes in rainfall on soil fauna populations is likely to be severe (Staley et al. Citation2007).
This study was carried out in a Mediterranean cork oak (Quercus suber L., 1753) forest in northwestern Italy during an annual cycle. Cork oak is a Mediterranean evergreen tree species with a thick and characteristic bark covering the trunk and branches – the cork. Despite the fact that cork oak woodlands occupy about 1.7 million hectares in the western Mediterranean Basin (Mendes & Graca Citation2009), information on soil biodiversity in this important habitat is currently scanty.
In this study we aimed to answer two methodological questions about the application of some soil biological quality indices:
Can seasonal variation and changes of weather, typical of the Mediterranean areas, influence biological indices based on soil microarthropods?
Are synthetic indices, such as QBS-ar, better tools in Mediterranean ecosystems compared to other indices e.g. Shannon Diversity index, Acari and Collembola ratio, etc.?
Two more questions were specifically aimed to characterize soil microarthropod community in a cork oak forest, a peculiar Mediterranean habitat:
What is the level of soil microarthropod biodiversity in a cork oak forest?
Are soil microarthropods sensitive to seasonal variation in this type of wood?
Material and methods
Study area
The study area is located in the cork tree wood of Bergeggi (Province of Savona, Liguria, NW Italy: 44°15′27′′ N, 08°26′35′′E). The station extends over a steep (35°), straight, south-facing slope, at about 150–200 m above sea level. The geological substratum consists of quartz/mica schists (Scisti di Gorra Formation) and the soil parent material appears to be of weathering material in situ, overlaying the hard rock. The cork trees (Q. suber) are organized in more or less thick nuclei, together with downy oak (Quercus pubescens Willd, 1805) or, towards the edges of the area, in isolated specimens. The station may have had a greater extension in the past, since other cork trees are widespread in the adjacent urbanized area near S. Sebastiano. Specimens of holm oak (Quercus ilex L., 1753) are present too, and the undergrowth is dominated by cistus (Cistus salvifolius L., 1753, Cistus monspeliensis L., 1753), myrtle (Mirtus communis L., 1753), Butcher’s broom (Ruscus aculeatus L., 1753) and mastic tree (Pistacia lentiscus L., 1753). The climate of this area has been carefully monitored at a nearby meteorological station (Capo di Vado): based on monthly data collected in the 5-year period 2006–2010, shows a marked Mediterranean climate characterised by summer drought between June and August. According to the Worldwide Bioclimatic Classification System (Citation1996–2009), its bioclimate is Pluvioseasonal Oceanic Mediterranean.
Figure 1. Ombrothermic diagram from monthly mean temperatures and rainfalls data collected by the Capo di Vado meteorological station (2006–2010).
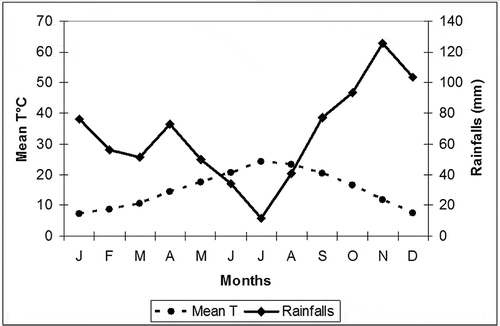
The considerable interest of such an area in terms of soil fauna biodiversity is evidenced by the collection of 11 species of Protura (Galli et al. Citation2012) and many interesting Pseudoscorpionida and Coleoptera species (G. Gardini and R. Poggi unpub.) as well as by the co-occurrence of two Palpigradi species (Christian et al. Citation2010), the description of one being based on material collected here (Eukoenenia gallii Christian, 2009).
Soil characterization
A characterization of the soil was performed by the description of a soil profile consisting of small excavated pits large enough to enable the different horizons to be examined and described: it was excavated and described until a depth was reached which was little affected by pedogenetic processes (BC horizon, 40 cm) and biological activity (rhizosphere). Field descriptions were carried out in accordance with Food and Agriculture Organization methods and terminology (FAO Citation2006).
The profile consists of thick undecomposed and partially decomposed litter (Oi–Oe–Oa; 0–10 cm) at the surface of a very dark brown mineral horizon characterized by accumulation of humified organic matter mixed with the mineral fraction (A; 10–14 cm). A transitional BC horizon has been distinguished under the A horizon. It is characterized by a moderately coarse blocky structure and common coarse angular rock fragments ().
In order to characterize the physical and chemical properties of this soil, we sampled each solum horizon (approximately 1 kg). Routine laboratory analyses were performed in compliance with officially prescribed Italian methods (MiPAF Citation2000): soil samples were air-dried, particle size distribution analysis was carried out by wet-sieving for the fraction > 50 μm and the composition of the fine fraction (<50 μm) was determined by a pipette procedure after dispersion of the sample with sodium hexametaphosphate, (NaPO3)6. The pH was measured with the potentiometric method in a 1:2.5 soil:water suspension, and electrical conductivity was measured in a 1:5 soil:water suspension. The total carbonate content was determined using the Dietrich Früling calcimeter. The total organic carbon (C) and nitrogen (N) contents were determined using an elemental analyzer based on Dumas’ (Citation1831) methods; the soluble phosphorus (P) was determined with sodium bicarbonate (NaHCO3) (Olsen et al. Citation1954). The cation exchange capacity (C.E.C.) and exchangeable bases were determined with barium chloride (BaCl2)-triethanolamine at pH 8.2; the concentrations of chemical elements extracted were determined by atomic absorption and flame emission spectrophotometry (FAAS).
The main physical and chemical characteristics of the soil are summarized in . The particle-size analysis is in agreement with the field description. In most organic layers, the determination of the texture class was not possible due to the low content of mineral grains. Stones (Ø > 2 mm) are common in the upper mineral horizon (A) of the profile while there is an increase in the percentage of sand and stones as the depth increases (BC), reflecting the presence of the weathered rock substratum. The significant values of the clay and silt fraction in the BC horizon indicate a moderately pedogenic weathering.
Table I. The main physical and chemical features.
The pH (H2O) values are rather acid, increasing from 5.3 at the surface to 5.7 at the bottom of the profile. All horizons revealed complete leaching of carbonates (decarbonated), while organic matter was concentrated only in the surface horizon where mostly leaves and twigs fall to the ground and are subsequently mixed with the soil. The pH of the soil developed from quartzitic rocks was often acid because of the low carbonate content. C.E.C. was variable, ranging between 14 and 37.4 meq/100g. The high values of the C.E.C. in the superficial horizons are certainly related to the level of organic matter content. Calcium (Ca) was the dominant exchangeable cation in all samples, whereas magnesium (Mg) and potassium (K) were much lower and sodium (Na) negligible. The base saturation (BS = exchangeable bases/cation exchange capacity) percentage ranged from 41.5 to 32%, these low values possibly explaining the low pH. The amount of P found in the horizons was very small while they displayed a moderate content of N.
The C/N ratio was 15–20 and the acid pH of the organic horizons was typical of the Moder humus form. These horizons consisted of moderately-humified and decomposed organic matter with moderately nutrient-poor conditions. In fact the soil acidification implies a decrease in litter decomposition rates and is reinforced by slow humification of organic matter (Stevenson Citation1994). Acidophilic fungi and arthropods participate in the transformation of plant residues. Mineral-organic compounds produced were labile and weakly bound with mineral portions of soil; in fact the low thickness litter (Oi–Oe), gradually, without bounds, pass on to humus-accumulative horizons (Oa–A).
Sampling and microarthropod extraction
In the study site, a representative area for soil sampling, homogeneous in terms of slope and vegetation, was delimited in the central part of the cork tree wood, to avoid edge effects. Samples were taken monthly from February 2007 to January 2008: on each sampling occasion 10 soil samples measuring 10 × 10 × 10 cm were randomly collected after removing above ground plant cover and superficial litter. Microarthropods were extracted into 70% Ethanol using Berlese-Tullgren funnels (2.5 mm mesh size) for 7 days. The number of specimens of each taxon was then recorded.
Soil biological quality and biodiversity indices
Soil quality was estimated using the QBS-ar index (Parisi et al. Citation2005; Gardi et al. Citation2008; Tabaglio et al. Citation2009; Menta et al. Citation2011). The QBS-ar index is based on the following concept: the higher the soil quality, the higher will be the number of microarthropod groups which are well adapted to soil habitats. QBS-ar is applied to soil microarthropods, separated in accordance with the biological form approach (sensu Sacchi & Testard Citation1971), with the intention of (1) evaluating the microarthropods’ level of adaptation to life in the soil environment and (2) overcoming the well-known difficulties of taxonomic analysis to species level for soil mesofauna. Edaphic microarthropods show morphological characteristics that give evidence of adaptation to soil environments, such as reduction or loss of pigmentation and visual apparatus; streamlined body form, with reduced and more compact appendages (hairs, antennae, legs); reduction or loss of flying, jumping or running adaptations, and a reduced water-retention capacity – e.g. thinner cuticle and a lack of hydrophobic compounds on the outer surface. By focusing on the presence of these characteristics, and not requiring the complex taxonomic identification to the species level, QBS-ar analysis can also be performed by non-specialists. The main phases for obtaining the QBS-ar values are: (1) sampling; (2) microarthropod extraction; (3) preservation of the collected specimens; (4) determination of biological forms; (5) calculation of the QBS-ar index (Parisi et al. Citation2005).
The biodiversity of the soil communities was evaluated using the number of observed taxa (NT), the Shannon-Weiner diversity index (H’) and Pielou’s evenness index (J). These diversity measures were calculated using the number of specimens observed in each sample identified at the taxonomical level as mentioned above.
Statistical analysis
In accordance with Blasi et al. (Citation2013), before carrying out the statistical analysis, we removed abundance data relating to Acari and Collembola, since they were present (as dominant or sub-dominant) in all samples and represented by the maximum EMI value (20).
One-way analysis of variance (ANOVA) was performed to test for differences between monthly collections; prior to running the analyses, the homogeneity of variances was tested using Levene’s test; whenever necessary, data were re-transformed and re-tested (Underwood Citation1997). When transformation did not produce homogeneous variances, we set α = 0.01, in order to make up for the increased likelihood of type 1 error. Post-hoc multiple comparisons after ANOVA were made by Tukey’s honestly significant differences test (Sokal & Rohlf Citation1995). The same test was performed to assess differences between monthly H’, J and QBS-ar index values. The correlation between biodiversity and calculated quality indices was performed using Spearman’s rho test. Finally, the above mentioned index values were regressed with the inclusion of rainfall data.
To highlight any compositional differences between the sampling months that could help us to understand the observed trend in H’, J and QBS-ar index values, we created a dataset from which we deleted rare taxa (i.e. taxa we found in less of 0.5% of the analyzed communities, nevertheless maintaining 98.6% of the total specimens): these could represent the so-called “background noise” which often makes it necessary to screen the original data. In accordance with this procedure, we deleted taxa such as Opiliones (0.04%), Isopoda (0.3%), Chilopoda Lithobiomorpha (0.08%), Diplopoda Glomeridae (0.07%), Diplopoda Julidae (0.1%), Blattaria, Embioptera, Psocoptera and Emiptera (0.03%), Orthoptera and Trichoptera (0.01%) and Lepidoptera larvae (0.1%).
On the dataset thus obtained, a centred Principal Components Analysis (PCA) was performed on log (x + 1) transformed data, to visualize any relationships or differences between the sampling months based on the detected taxa. Then Analysis of Similarity (One-way ANOSIM with Bonferroni correction) was performed using the Bray-Curtis distance measure to test similarity (homogeneity) within the sampling months.
The above mentioned statistical analyses were performed using PAST Software (PAleontological STatistics ver. 2.16) (Hammer et al. Citation2001).
Results
Microarthropod communities
A total of 29 taxonomic groups, distributed between Chelicerata, crustaceans, miriapods and Hexapoda, were identified from the 120 soil samples collected. Excluding mites and springtails, for which we considered the abundance range (for more details see data analysis section), a total of 12,388 individuals were extracted. shows the mean relative frequency (%) of each of the individual abundances for each taxon extracted from the 10 soil cores sampled during each monthly sampling session. Acari and Collembola were the most abundant groups sampled in all months (the abundances ranged between 100 and 1000 specimens every month). Polyxenida, Symphyla, Protura, Diplura, Coleoptera larvae and Diptera larvae represented each at least 5% of the total specimens in at least 8 months. Chilopoda Lithobiomorpha and Scolopendromorpha, Diplopoda Glomerida, Blattaria, Embioptera, Psocoptera and Hemiptera failed to reach 1% abundance in all months. For the other groups, the relative abundance ranged between 1 and 5%, exceeding 5% for only a few months of the year.
Table II. Mean and Standard Deviation of the relative frequencies (%) of the individual abundances for each taxon extracted from the 10 soil cores sampled during each monthly sampling session, Acari and Collembola abundance range, biological quality of soil based on arthropods (QBS-ar) index, Shannon-Weiner diversity index (H’) and Pielou’s evenness index (J) values.
Statistical analysis failed to highlight a clear density distribution of the taxa during the months. This failure could be due to the excessively high intra-taxon variability of the densities, which could have masked the differences between months. In spite of this, it is possible to identify a distribution trend for some groups. In detail, the Palpigrada presence was higher from June to October; Pauropoda showed a similar trend, with the highest density from April to January while it was possible to identify a peak in autumn. Pseudoscorpions and Polyxenida miriapods showed the highest densities from July to January and from August to January respectively. In contrast, Geophilomorpha centipedes reached their highest density during the period from late autumn to early spring. Similar trends were detected for Polydesmida miriapods and for Protura, which revealed their highest density in winter and spring. Lastly, adult Coleoptera displayed their highest density from April to October. The remaining groups displayed no evident trends.
Indices
The QBS-ar index reached the lowest (147) and the highest (230) values in August and June respectively, the H’ index in November (1.54) and June (2.04) and the J index in February (0.71) and January (0.83). The statistical analysis on the QBS-ar values calculated for each month detected significant differences (F = 5.344, p < 0.001): post-hoc multiple comparisons (Tukey’s test) indicated that the QBS-ar mean value for June was significantly higher than the mean values calculated for February (p < 0.01), August (p < 0.001) and November (p < 0.001); furthermore, the QBS-ar mean value estimated for August was significantly lower than those calculated for June (p < 0.001), July (p < 0.01) and January (p < 0.01). Moreover, for the H’ index, the ANOVA revealed significant differences between the monthly calculated values (F = 2.78, p < 0.01), though Tukey’s test did not highlight significant differences. The ANOVA performed on the J index did not reveal differences between the months (F = 1.604, p > 0.05).
The H’ index was highly correlated with QBS-ar (correlation coefficient value (r): 0.86), while J was not correlated with the other two indices. None of the indices considered was correlated with either temperature or rainfall.
The PCA scatter plot () appeared to show a differentiation between four periods: July–September, October–January, February–May and June. However, ANOSIM revealed that each month differed significantly from the others (r = 0.37, p < 0.01), thus not confirming the seasonal trend perceivable in the PCA analysis.
Discussion
The results obtained in this study showed the importance of cork woods as a reservoir of biodiversity, in particular for microarthropod communities: in this environment we found most adapted to natural and stable soils microarthropod taxa such as Palpigradi, Pauropoda, Symphyla and Protura. In particular, the constant presence and high abundance of Palpigradi throughout the year (Christian et al. Citation2010) suggests stable and undisturbed soil conditions. Furthermore, the presence of Pseudoscorpionida, many Myriapoda taxa and Protura confirmed this conclusion. The cork wood studied showed a microarthropod richness which was close to that observed in beech or in other forests and higher than in agricultural environments (Tabaglio et al. Citation2008; Sapkota et al. Citation2012), degraded soils (Menta et al. Citation2008) or urban ecosystems (Santorufo et al. Citation2012). The results obtained from the biodiversity and soil biological quality index calculations led to the same conclusions: H’ and QBS-ar indices showed values close to those recorded in turkey oak and beech woods (Blasi et al. Citation2013) and higher than those obtained in grasslands (Menta et al. Citation2011), in pastures (Zucca et al. Citation2010), in agricultural ecosystems (Tabaglio et al. Citation2008; Mazzoncini et al. Citation2010; Sapkota et al. Citation2012), in post-mining soils (Madej et al. Citation2011), in urban soils (Hartley et al. Citation2008; Santorufo et al. Citation2012), in soils treated with sewage sludge (Andrés et al. Citation2011) and in plant-remediated sediment-derived soil (Hartley et al. Citation2011).
Our results on soil characteristics are consistent with the observations of other authors studying the relationships between soil fauna communities, humus forms and soil properties. The diversity of zoological groups (Shannon index) can be linearly correlated to soil pH, humus forms and levels of organic C (Hasegawa Citation2002; Salmon et al. Citation2008). However, soil pH had a significant direct positive effect on the richness of fauna as lignin content in the litter (Moco et al. Citation2010). For instance the densities and local diversity of Collembola were observed to increase with soil acidity (Loranger et al. Citation2001), expressed by (a) lower pH and (b) lower content in total bases. Regarding the influence of humus forms, Takeda (Citation1987) found that Collembola species increased their density in line with the amount of litter (Oi and Oe, the zone of most saprophagous activity). Sadaka and Ponge (Citation2003) showed that there was a decrease in the population size of most zoological groups from organic (Oi, Oe) to mineral horizons (A, B), but Oi and Oe horizons varied as the most populated horizon depending on the years and animal groups. Finally, a higher density of invertebrates and abundance of Collembola was observed in the south-facing site, due to higher solar radiation (Salmon et al. Citation2008).
One of the aims of this study was to evaluate the monthly or seasonal fluctuations in microarthropods. Statistical analysis failed to highlight a clear trend in the taxa abundances across the months. In our opinion, the variability in the 10 soil samples collected each month was so high that it increased the intra-month variability. We suppose that this is due to the aggregate distribution of many of the soil arthropod groups, so that they are abundant (or even dominant) in some samples and scanty or absent in others collected during the same day, a few metres away. These results confirm the importance of collecting more sub-samples in each study locality, to obtain a more complete and representative picture of the soil community composition in different micro-habitats.
Our results also seem to indicate that, contrary to what emerged from a study of broadleaf deciduous forests typical of a temperate climate (Blasi et al. Citation2013), in which QBS-ar data can be compared even when samples were collected in different months, in Mediterranean sclerophyllous woods and scrub, QBS-ar values vary monthly in each locality, making it impossible to compare two or more areas using results from samplings carried out in different seasons. Therefore, in the sampling design of projects and studies aimed at the evaluation of soil biological quality it is important to carefully take into account this last aspect. This study showed that in Mediterranean sclerophyllous woods the worst soil biological quality values are recorded during summer, and in particular in August. It is well known that during this period soil conditions are generally inhospitable for soil fauna and many species belonging to different taxa enter a quiescent condition, others move in depth and, finally, others perish. For this reason, it becomes very important to collect soil samples for microarthropod extraction and QBS-ar application during the spring (which for Italy corresponds to June and July) in the Mediterranean sclerophyllous woods, when the microarthropod community is more active and the QBS-ar values are highest. In this way, we will obtain information about the maximum level of biodiversity and the presence in a specific soil of groups well adapted to soil and therefore more vulnerable.
Finally, according to this study, the QBS-ar index seems to be a good tool to evaluate soil biological quality compared to other indices such as the Shannon Diversity index, density of specimens, etc., in particular when sample collections are limited in time or space. In conclusion, QBS-ar seems to be a good candidate for the evaluation of soil biological quality in natural and degraded soils in Mediterranean areas, provided it is applied following a sampling scheme that takes into account the ecological peculiarities of each habitat.
References
- Anderson DC. 1987. Below-ground herbivory in natural communities: A review emphasising fossorial animals. The Quarterly Review of Biology 62:261–286.
- Andrés P, Mateos E, Tarrasóna D, Cabrera C, Figuerola B. 2011. Effects of digested, composted, and thermally dried sewage sludge on soil microbiota and mesofauna. Applied Soil Ecology 48:236–242.
- Arredondo F, García-Montero LG, Menta C, Valverde-Asenjo I. in press. Soil quality indicators for forest management. In: Martínez-Falero E, Martín-Fernández S, García-Abril A, editors. Quantitative techniques in participatory forest management, Boca-Ratón, FL: Taylor & Francis Group.
- Aspetti GP, Boccelli R, Ampollini D, Del Re AAM, Capri E. 2010. Assessment of soil-quality index based on microarthropods in corn cultivation in Northern Italy. Ecological Indicators 10:129–135.
- Bale JS, Masters GJ, Hodkinson ID, Awmack C, Bezemer TM, Brown VK, Butterfield J, Buse A, Coulson JC, Farrar J, Good JEG, Harrington R, Hartley S, Jones TH, Lindroth RL, Press MC, Symrnioudis I, Watt AD, Whittaker JB. 2002. Herbivory in global climate change research: Direct effects of rising temperature on insect herbivores. Global Change Biology 8:1–16.
- Bernini F, Avanzati AM, Baratti M, Migliorini M. 1995. Oribatid mites (Acari Oribatida) of the Farma Valley (Southern Tuscany). Notulae Oribatologicae LXV. Redia LXXVIII. 45–129.
- Blakely JK, Neher DA, Spongberg AL. 2002. Soil invertebrate and microbial communities, and decomposition as indicators of polycyclic aromatic hydrocarbon contamination. Applied Soil Ecology 21:71–88.
- Blasi S, Menta C, Balducci L, Conti FD, Petrini E, Piovesan G. 2013. Soil microarthropod communities from Mediterranean forest ecosystems in Central Italy under different disturbances. Environmental Monitoring and Assessment 187:1637–1655.
- Blondel J, Aronson J, Bodiou JY, Boeuf G. 2010. The Mediterranean region. Biological diversity in space and time. 2nd ed. Oxford: Oxford University Press. 376 pp.
- Brown VK, Gange AC. 1990. Insect herbivory below ground. Advances in Ecological Research 20:1–58.
- Buckland SM, Grime JP, Hodgson JG, Thompson K. 1997. A comparison of plant responses to the extreme drought of 1995 in northern England. Journal of Ecology 85:875–882.
- Christian E, Capurro M, Galli L. 2010. Phenology of two syntopic Eukoenenia species in a northern Italian forest soil (Arachnida: Palpigradi). Revue Suisse de Zoologie 117:829–834.
- Cluzeau D, Guernion M, Chaussod R, Martin-Laurent F, Villenave C, Cortet J, Ruiz-Camacho N, Pernin C, Mateille T, Philippot L, Bellido A, Rougé L, Arrouays D, Bispo A, Pérès G. 2012. Integration of biodiversity in soil quality monitoring: baselines for microbial and soil fauna parameters for different land-use types. European Journal of Soil Biology 49:63–72.
- Cortet J, Gomot de Vauflery A, Poinsot-Balaguer N, Gomot L, Texier C, Cluzeau D. 1999. The use of invertebrate soil fauna in monitoring pollutant effects. European Journal of Soil Biology 35:115–134.
- Dumas JBA. 1831. Procédés de l‘analyse organique. Annales des Chimie et des Physique 247:198–213.
- FAO. 2006. World reference base for soil resources 2006. A framework for international classification, correlation and communication. 2nd edition. World Soil Resources Reports No. 103. Rome: FAO.
- Frampton GK. 1997. The potential of Collembola as indicators of pesticide usage evidence and methods from the UK arable ecosystem. Pedobiologia 41:179–184.
- Galli L, Capurro M, Shrubovych J, Torti C. 2012. Phenology of Protura in a northwestern Italian forest soil. (Hexapoda: Protura). Acta Zoologica Cracoviensia 55:33–43.
- Gardi C, Menta C, Leoni A. 2008. Evaluation of environmental impact of agricultural management practices using soil microarthropods. Fresenius Environmental Bulletin 17:1165–1169.
- Gardi C, Tomaselli M, Parisi V, Petraglia A, Santini C. 2002. Soil quality indicators and biodiversity in northern Italian permanent grasslands. European Journal of Soil Biology 38:103–110.
- Hammer Ø, Harper DAT, Ryan PD. 2001. PAST: Paleontological Statistics Software Package for Education and Data Analysis. Palaeontologia Electronica 4:9 pp. http://palaeo-electronica.org/2001_1/past/issue1_01.htm. Accessed May 2011 5.
- Hartley W, Dickinson NM, Riby P, Shutes B. 2012. Sustainable ecological restoration of brownfield sites through engineering or managed natural attenuation? A case study from Northwest England. Ecological Engineering 40:70–79.
- Hartley W, Riby P, Dickinson NM, Shutes B, Sparke S, Scholz M. 2011. Planting woody crops on dredged contaminated sediment provides both positive and negative effects in terms of remediation. Environmental Pollution 159:3416–3424.
- Hartley W, Uffindell L, Plumb A, Rawlinson HA, Putwain P, Dickinson NM. 2008. Assessing biological indicators for remediated anthropogenic urban soils. Science of the total Environment 405:358–369.
- Hasegawa M. 2002. The response of collembolan community to the amount and composition of organic matter of a forest floor. Pedobiologia 46:353–364.
- Hishi T, Fujimaki R, McGonigle TP, Takeda H. 2008. Relationships among fine roots, fungal hyphae and soil microarthropods among different soil microhabitats in a temperate coniferous forest of Chmaecyparis obtusa. European Journal of Soil Biology 44:473–477.
- Huhta V. 2002. Soil microarthropods communities in planted birch stands in comparison with natural forests in central Finland. Applied Soil Ecology 20:199–209.
- Jeffery S, Gardi C, Jones A, Montanarella L, Marmo L, Miko L, Ritz K, Peres G, Römbke J, van der Putten WH, editors. 2010. European atlas of soil biodiversity. Luxembourg: Publications Office of the European Union.
- Klironomos JN, Kendrick B. 1995. Relationships among microarthropods, fungi, and their environment. Plant and Soil 170:183–197.
- Loranger G, Bandyopadhyaya I, Razaka B, Ponge J-F. 2001. Does soil acidity explain altitudinal sequences in collembolan communities?. Soil Biology & Biochemistry 33:381–393.
- Madej G, Barczyk G, Gdawiec M. 2011. Evaluation of Soil Biological Quality Index (QBS-ar): Its sensitivity and usefulness in the post-mining chronosequence – Preliminary research. Polish Journal of Environmental Studies 20:1367–1372.
- Masters GJ, Brown VK, Clarke IP, Whittaker JB, Hollier JA. 1998. Direct and indirect effects of climate change on insect herbivores: Auchenorrhyncha (Homoptera). Ecological Entomology 23:45–52.
- Mazzoncini M, Canali S, Giovannetti M, Castagnoli M, Tittarelli F, Antichi D, Nannelli R, Cristiani C, Bàrberi P. 2010. Comparison of organic and conventional stockless arable systems: A multidisciplinary approach to soil quality evaluation. Applied Soil Ecology 44:124–132.
- Mendes AMSC, Graça JAR. 2009. Cork bottle stoppers and other cork products. In: Aronson J, Pereira JS, Pausas JG, editors. Cork oak woodlands on the edge, Washington, DC: Society for Ecological Restoration International/Island Press. pp. 59–69.
- Menta C, Leoni A, Bardini M, Gardi C, Gatti F. 2008. Nematode and microarthropod communities: Comparative use of soil quality bioindicators in covered dump and natural soils. Environmental Bioindicators 3:35–46.
- Menta C, Leoni A, Gardi C, Conti F. 2011. Are grasslands important habitats for soil microarthropod conservation?. Biodiversity and Conservation 20:1073–1087.
- MiPAF (Ministero delle Politiche Agricole e Forestali). 2000. Osservatorio nazionale pedologico e per la qualità del suolo. International Society of Soil Science, Società Italiana della Scienza del Suolo. In: P. Violante. Metodi di analisi chimica del suolo. Milano: F. Angeli Ed.
- Moco MKS, Gama-Rodriguea EF, Gama-Rodrigues AC, Machado RCR, Baligar VC. 2010. Relationships between invertebrate communities, litter quality and soil attributes under different cacao agroforestry systems in the south of Bahia, Brazil. Applied Soil Ecology 46:347–354.
- Olsen SR, Cole CV, Watanabe FS, Dean LA. 1954. Estimation of available phosphorus in soils by extraction with sodium bicarbonate. USDA circular 939. Washington D.C: U.S. Government Printer Office.
- Paoletti MG. 1999. The role of earthworms for assessment of sustainability and as bioindicators. Agriculture, Ecosystem and Environment 74:137–155.
- Paoletti MG, Hassal M. 1999. Woodlice (Isopoda: Oniscidea): Their potential for assessing sustainability and use as bioindicators. Agriculture, Ecosystem and Environment 74:157–165.
- Parisi V, Menta C. 2008. Microarthropods of the soil: Convergence phenomena and evaluation of soil quality using QBS-ar and QBS-c. Fresenius Environmental Bulletin 17:1170–1174.
- Parisi V, Menta C, Gardi C, Jacomini C, Mozzanica E. 2005. Microarthropod community as a tool to asses soil quality and biodiversity: a new approach in Italy. Agriculture Ecosystem and Environment 105:323–333.
- Richardson SJ, Press MC, Parson AN, Hartley SE. 2002. How do nutrients and warming impact on plant communities and their insect herbivores?. Journal of Ecology 90:544–556.
- Ruf A. 1998. A maturity index for predatory soil mites (Mesostigmata: Gamasina) as indicators of environmental impacts of pollution on forest soils. Applied Soil Ecology 9:447–452.
- Sacchi CF, Testard P. 1971. Ecologie Animale. Paris: Doin.
- Sadaka N, Ponge JF. 2003. Soil animal communities in holm oak forests: Influence of horizon, altitude and year. European Journal of Soil Biology 39:197–207.
- Salmon S, Artuso N, Frizzera L, Zampedri R. 2008. Relationships between soil fauna communities and humus forms: Response to forest dynamics and solar radiation. Soil Biology & Biochemistry 40:1707–1715.
- Santorufo L, Van Gestel CAM, Rocco A, Misto G. 2012. Soil invertebrates as bioindicators of urban soil quality. Environmental Pollution 161:57–63.
- Sapkota TB, Mazzoncini M, Bàrberi P, Antichi D, Silvestri N. 2012. Fifteen years of no till increase soil organic matter, microbial biomass and arthropod diversity in cover crop-based arable cropping systems. Agronomy for Sustainable Development 32:853–863.
- Setälä H, Marshall VG, Trofymow JA. 1995. Influence of micro- and macro-habitat factors on collembolan communities in Douglas-fir stumps during forest succession. Applied Soil Ecology 2:227–242.
- Sokal RR, Rohlf FJ. 1995. Biometry. The principle and practice of statistics in biological research. 3rd ed. New York: W. H. Freeman and Co. 853 pp.
- Staley JT, Hodgson CJ, Mortimer SR, Morecroft MD, Masters GJ, Brown VK, Taylor ME. 2007. Effects of summer rainfall manipulations on the abundance and vertical distribution of herbivorous soil macro-invertebrates. European Journal of Soil Biology 43:189–198.
- Stenberg M. 2000. Terrestrial gastropods and experimental climate change: A field study in a calcareous grassland. Ecological Research 15:73–81.
- Stevenson FJ. 1994. Humus chemistry. Genesis, composition, reactions. 2nd ed. New York: Wiley.
- Tabaglio V, Gavazzi C, Menta C. 2008. The influence of no-till, conventional tillage and nitrogen fertilization on physico-chemical and biological indicators after three years of monoculture barley. Italian Journal of Agronomy 3:233–240.
- Tabaglio V, Gavazzi C, Menta C. 2009. Physico-chemical indicators and microarthropod communities as influenced by no-till, conventional tillage and nitrogen fertilisation after four years of continuous maize. Soil & Tillage Research 105:135–142.
- Takeda H. 1987. Dynamics and maintenance of Collembolan community structure in a forest soil system. Research in Population Ecology 29:291–346.
- Testi A, Fanelli G, Crosti R, Castigliani V, D’Angeli D. 2012. Characterizing river habitat quality using plant and animal bioindicators: A case study of Tirino River (Abruzzo Region, Central Italy). Ecological Indicators 20:24–33.
- Underwood AJ. 1997. Experiments in ecology: their logical design and interpretation using analysis of variance. Cambridge: University Press. pp 504.
- Van Straalen NM. 2004. The use of soil invertebrates in ecological survey of contaminated soils. In: Doelman P, Eijsackers HJP, editors. Vital soil function, value and properties. Amsterdam: Elsevier. pp. 159–194.
- Villenave C, Bongers T, Ekschmitt K, Djigal D, Chotte JL. 2001. Changes in nematodes communities following cultivation of soil after fallow periods at different length. Applied Soil Ecology 17:43–52.
- Visioli G, Menta C, Gardi C, Conti FD. 2013. Metal toxicity and biodiversity in serpentine soils: Application of bioassay tests and microarthropod index. Chemosphere 90:1267–1273.
- Wolters V. 2001. Biodiversity of soils animals and its function. European Journal of Soil Biology 37:221–227.
- Worldwide Bioclimatic Classification System. 1996–2009. S. Rivas-Martinez & S. Rivas-Saenz, Phytosociological Research Center, Spain. Available: www.globalbioclimatics.org, Accessed May 2011 5.
- Zucca C, Canu A, Previtali F. 2010. Soil degradation by land use change in an agropastoral area in Sardinia (Italy). Catena 83:46–54.