Abstract
Predator control has historically shaped management towards minimising predator pressure on sensitive species in most hunting and protected areas. Despite widespread use, few studies of medium-sized carnivores have evaluated such control. To fill this void, we studied the effect of control on shaping a carnivore community consisting of European badgers Meles meles Linnaeus, 1758, stone martens Martes foina Erxleben, 1777 and red foxes Vulpes vulpes Linnaeus, 1758, in the Serra de Montsant area (NE Spain), and we report the recovery dynamics for different habitats (forest, scrubland and cultivated land) over time (3 years) when that control was halted. The results show that non-selective predator control is inadequate to reconcile the conservation of ecosystems with hunting. Specifically, it favours the frequency of occurrence of red foxes, which are the main consumer of small game species, and also reduces the frequency of occurrence of European badgers and stone martens, which are protected species. The recovery or regression sequence was observed over time in an area where there had previously been control, and was compared with the point of reference provided by an uncontrolled area. The results show the recovery of European badgers (135% over 3 years) and stone martens (285% over 3 years), and the regression of red foxes (–10% over 3 years) when control is halted. Monitoring the different habitats, European badgers recover first on cultivated land, then scrubland and more slowly in forest. Stone martens recover first in forest, and then scrubland and cultivated land. In contrast, red foxes maintain their numbers in scrubland, and decline slightly in forest and on cultivated land. The observed preferences have been associated with the capacity to meet basic needs of food, protection and balance with competitors.
Introduction
The reduction in the population of some species of Carnivora (Woodroffe Citation2001) and the effect on other farmed, game or endangered species (Sillero-Zubiri & Switzer Citation2004) has historically shaped management strategies towards minimising predation pressure on sensitive species in most hunting and protected areas (Whitfield et al. Citation2003). To achieve this objective, predator control methods are used (Reynolds & Tapper Citation1996), which usually imply a reduction in predator populations (Sillero-Zubiri & Switzer Citation2004) in order to increase game abundance and reproductive success (Graham et al. Citation2005; Beja et al. Citation2009). Although these aims are not always achieved (Heydon et al. Citation2000a, b; Virgós & Travaini Citation2005), game keepers see predator control as an essential tool in maintaining or enhancing game population sizes and increasing economic revenue from related activities (Reynolds & Tapper Citation1996; Delibes-Mateos et al. Citation2013). In general, the effect of predator control varies depending on the selectivity, intensity, scope and duration of the programme, and the biology of the species involved (Reynolds et al. Citation1993; Harding et al. Citation2001; Baker & Harris Citation2006; Duarte et al. Citation2012). When control is maintained over time, the interactions that occur can be explained by the mechanisms of the competitor release effect (Caut et al. Citation2007; Casanovas et al. Citation2012). When the control is not maintained over time, or only covers small areas, the results are usually very limited (Reynolds et al. Citation1993; Harding et al. Citation2001; McDonald & Harris Citation2002).
When control is halted or the natural causes which led to a population decline disappear, carnivores’ recovery depends on many factors and varies greatly from one species to another (Frank & Woodroffe Citation2001). Wolves (Canis lupus Linnaeus, 1758), coyotes (Canis latrans Say, 1823) and lions (Panthera leo Linnaeus, 1758) readily move into vacated areas (Beasom Citation1974; Smuts Citation1978; Fritts & Mech Citation1981), and so are able to recolonise rapidly, due to their reproductive flexibility and dispersal ability (Frank & Woodroffe Citation2001). On the other hand, with a similar reproductive capacity at group level, hyaena recovery is extremely slow because females are extraordinarily philopatric (Holekamp et al. Citation1993). Furthermore, higher recovery rates have been observed in plots close to any high-density or protected African wild dog (Lycaon pictus Temminck, 1820) populations (Fanshawe et al. Citation1997; Mills & Hofer Citation1998). Despite these clear differences, recovery rates are not solely dependent upon species biology. In addition to intrinsic growth rates and immigration, the composition and physiognomy of the various components of the landscape explain the differential response of carnivores to the use of vacated plots (Bender et al. Citation2003; Mortelliti & Boitani Citation2008) and recovery time (Fleishman et al. Citation2002). Most landscapes are complex mosaics of many kinds of habitat, some of which meet the ecological requirements to ensure the fitness of the species, whilst the most inhospitable take longer to be occupied (Hanski Citation2009).
Few prior studies on medium-sized carnivores have investigated the role of predator control in the shaping and composition of carnivore communities (Beja et al. Citation2009), and even fewer have described the habitat recovery dynamics when control is halted. To address this lack, we studied the effect of non-selective predator control on population trends in habitat recovery in a carnivore community, European badger Meles meles (Linnaeus, 1758), stone marten Martes foina (Erxleben, 1777) and red fox Vulpes vulpes (Linnaeus, 1758,) in the Serra de Montsant area (NE Iberian Peninsula). These three species live in sympatry throughout much of their distribution in the Iberian Peninsula, where they have been well studied (Gortázar Citation2008; Reig Citation2008; Revilla et al. Citation2008). They are basically omnivorous, nocturnal or crepuscular, and show a broad overlap in resource use (habitat and food) (Larivière & Jennings Citation2009; Sillero-Zubiri Citation2009). The intrinsic growth rate of the red fox (r = 1.1) is greater than that of the European badger (r = 0.46) or stone marten (r = 0.62) (Casanovas et al. Citation2012). Red fox dispersal and colonisation ability is superior to that of European badger and stone marten (Blanco Citation1986; Libois & Waechter Citation1991; Ferrari Citation1997).
To determine the effect of predator control, we compared the frequency of occurrence of the three species in areas with and without control measures. The recovery or regression sequence was observed over time in an area where there had previously been control, and was compared with the point of reference provided by the uncontrolled area. Analysing recovery in each habitat allowed us to observe different behaviour in different parts of the landscape mosaic. With natural indicators (droppings identified on trails), it is possible to infer an increase or decrease in population numbers (Kruuk et al. Citation1980; Conner & Labisky Citation1985), and varying occupation or use of space (Heinemeyer et al. Citation2008). With this information obtained through non-invasive methods, it is reasonable to expect that after a period of non-selective predator control, the size of the European badger and stone marten populations (with lower intrinsic growth rate and lower dispersal capacity) would show a trend towards a decrease in number. However, we expected that red fox (with a higher intrinsic growth rate and remarkable dispersal abilities) would show a trend towards an increase in numbers. With few references regarding the recovery of habitats, it is also reasonable to expect that the recovery of the different components of the landscape mosaic is related to each species’ habitat preferences.
Materials and methods
Study area: description
The study area was the Serra de Montsant, a mountain range in Catalonia (NE Spain, 41°12'–41°22'N, 0°41'–0°56'E), with an approximate area of 29,000 hectares. The rugged relief of this mountain range mainly consists of Oligocene conglomerate. The altitude ranges between 214 and 1163 m above sea level. Hydrological resources are centred almost entirely on the Montsant and Siurana rivers, with a torrential regime and a marked dry season. A Mediterranean climate prevails, with an average annual temperature of 11–14°C and an average annual rainfall of 450–600 L/m2. The vegetation is typically Mediterranean, but with sub-Mediterranean and Euro-Siberian influences in some sections. The native vegetation was modified a long time ago and nowadays the area is very heterogeneous, characterised by a mosaic pattern of patches of forest, where Aleppo pine (Pinus halepensis Mille) is interspersed with Mediterranean scrubland of tall shrubs and rocks, dominated by kermes oak (Quercus coccifera L.), tree heath (Erica arborea L.), mastic (Pistacia lentiscus L.) and Genista sp., and there are permanent crops rich in groves of hazel, almond, olive and fruit trees, but especially vineyards. In global terms, habitat measurements are available with great reliability (IDESCAT, Generalitat of Catalonia, http://www.idescat.cat). The area includes streams and reservoirs, riparian vegetation and an extensive network of paths and tracks, disused crop fields and residential areas (). Breakdown of measurements by individual plots is not possible, since the landscape of Serra de Montsant is very fragmented, with extremely irregular geological formations and intertwined habitats. The area also hosts an important faunal community, with a considerable diversity of high-interest species (Barrull & Mate Citation2007). Prior to the 2005–2006 hunting season, two types of hunting management were identified in the study area: Private Hunting Areas without predator control (PHAw) (18,018 ha) and Private Hunting Areas with predator control (PHAc) (10,822 ha), according to official statistics (Autonomous Government of Catalonia) (IDESCAT, Generalitat of Catalonia). Habitat was similar throughout both areas and no marked differences were observed in terms of percentage land use (). In PHAw, the management strategy consisted mainly of releasing farm-bred small game such as red-legged partridge Alectoris rufa (Linnaeus, 1758) and rabbit Oryctolagus cuniculus Linnaeus, 1758. Predation control was non-existent or anecdotal. In PHAc, common practices still included the release of farm-reared game animals but focused especially on predator control. However, the available information was fragmentary, heterogeneous and not computerised. Some documents and capture records, referring to the period 1991 to 1999, were deposited at the Territorial Services of the Autonomous Government of Catalonia, but official information previous to this period was not available (Barrull et al. Citation2011). According to information provided by owners of the hunting areas and their trappers, predators had been culled for decades, and control methods were mostly based on snares (approximately 85%) and cage traps (approximately 15%), together with an uncontrolled percentage of body-gripping traps used by poachers. Culled species included European badgers, stone martens, red foxes, small-spotted genets Genetta genetta (Linnaeus, 1758), feral cats Felis silvestris catus Schreber, 1775, dogs Canis lupus familiaris Linnaeus, 1758, black-billed magpies Pica pica Linnaeus, 1758 and some birds of prey. From the 2005–2006 hunting season onwards, no predator control of any kind was performed.
Table I. Differences in size and land use composition between private hunting areas with non-selective control (PHAc) and private hunting areas without control (PHAw) in Serra de Montsant, northeast of the Iberian Peninsula, in 2005–2006.
Field study: experimental design
To compare the frequency of occurrence of the three species, droppings in the field and from latrines were collected along trails 1 km in length with a regular width of approximately 4 m. The entire length of each trail is included in a plot defined in the Habitats Map of the Cartographic Institute of Catalonia (2006, pp. 417, 444, 445). To avoid or minimise potential problems of spatial and temporal dependence of the data (Hurlbert Citation1984), the minimum distance between trails was 1 km and trails were examined in a random order, the same for seasons and years ().
Figure 1. Location of the Serra de Montsant and its municipalities in the northeast of the Iberian Peninsula. The gray lines indicate the scat deposition paths of the Private Hunting Area without control. The black lines indicate scat deposition paths of the Private Hunting Area with control. The white squares indicate villages: MOR (La Morera de Montsant), LVA (La Vilella Alta), LVB (La Vilella Baixa), CBC (Cabacés), LBF (La Bisbal de Falset), MGF (Margalef), ULL (Ulldemolins) and COR (Cornudella de Montsant).
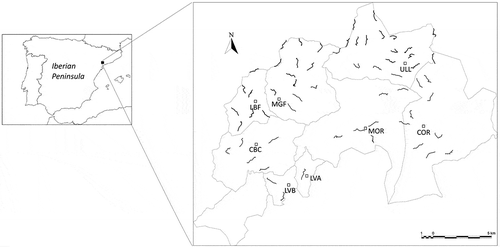
To contrast the frequency of occurrence, we evaluated 72 experimental conditions: 3 years (September 2008#x2013;August 2009, September 2009–August 2010 and September 2010–August 2011), four seasons (autumn, winter, spring and summer), three habitats (forest, scrubland and cultivated land) and two management regimes (PHAw and PHAc). To ensure equal representation of the experimental conditions and maximum power in the analysis, a fixed number of repetitions was assigned for each experimental condition (Norman & Streiner Citation2008). For each of these conditions, 10 trails were established (720 trails in total). Each trail was classified according to the predominant habitat of the polygon to which it belonged. Trail management regimes were determined on the basis of information provided by the Regional Services of the Environment and Housing Department of the Autonomous Government of Catalonia. For the present study, autumn was defined as September, October and November, winter as December, January and February, spring as March, April and May, and summer as June, July and August.
Frequency of occurrence has been shown to be a good indicator for comparing population trends. The use of radioactive isotopes has demonstrated that in comparable conditions (the same species, the same habitat and the same time periods), faecal signs can be used to infer increased or decreased population size and varying occupancy or use of the space (Kruuk et al. Citation1980; Conner & Labisky Citation1985; Heinemeyer et al. Citation2008). The collection of droppings along trails is a suitable method for detecting the presence of European badger (Virgós Citation2001b), stone marten (Virgós Citation2001a) and red fox (Webbon et al. Citation2004). To minimise problems associated with the use of scat deposition as a means of determining space occupation, droppings were cleared from all trails two weeks before data collection. To ensure repeatability of the measurements, the trails were monitored at the same speed by the same two researchers.
Scats from different species were identified by shape, size and location (Bang & Dahlstrøm Citation2007; Jędrzejewski & Sidorovich Citation2010). Cylindrical and pooled scats (10–15 cm long and 2–3 cm wide), sometimes fragmented or forming a loose amorphous mass, and left in oblong pits (latrines) were assigned to European badger; a single scat (though sometimes grouped) with a uniform tubular appearance (8–10 cm long and 1–1.2 cm wide), usually with a curved shape and tapered at one end, was assigned to stone marten; a single cylindrical scat (8–10 cm long and 1.5–2 cm wide), with a pointed tip and divided into 2–3 fragments, was assigned to red fox. This characterisation enabled us to discriminate faeces by species and avoid confusing them with those of other carnivores present in the study area. Exceptionally, when the identification of a particular scat was dubious, the scat was not included.
Statistical analysis
To quantitatively assess occurrence, each trail was divided into five 200-m sections, and the number of sections with observed occurrence of species was determined (Lozano et al. Citation2007). For each species, the frequency of occurrence thus defined on each trail was assigned values between 0 (no signs in any section) and 5 (presence of signs in all sections). To minimise problems associated with different intraspecific rates of faeces deposition, a record was considered positive when the presence of at least one sample of the study species was observed.
To contrast the effect of management conditions (control and non-control), time (sequence of recovery and regression), habitat (landscape features) and season on the frequency of occurrence, we used general linear models with third-order interaction of three- and four-level factors. The impact of predator control on each species was determined by contrasting management conditions with data corresponding to the first year of the study (design of three factors: the time was set at year 1). Regression or recovery of the species over time was determined by contrasting the effect of time on each of the management conditions (design of three factors: one corresponding to observations of PHAw, and the other corresponding to observations of PHAc). For each species, the habitat recovery sequence was obtained by comparing the evolution over time of the frequency of occurrence under the two management conditions (design of three factors: one for each habitat). The contrast of frequencies of occurrence for seasons was carried out with a complete four-factor design. The transformation proposed by Anscombe (Citation1948) was used to achieve homoscedasticity of variance:
where TA is the transformed frequency of occurrence, FO is the frequency of occurrence, and n is total number of observations per trail (in our case, n = 5). However, the balanced nature of the proposed designs (same number of replicates per experimental condition in all cases) made it possible to relax the normal condition of residuals where necessary, requiring only the unimodal condition (Peña Citation1994). To avoid a distorting effect that could lead to different detectability of droppings in habitats and different rates of interspecific and intraspecific defecation in time, the frequencies of occurrence of the same species, in the same habitat and for the same time period were contrasted in all cases. Findings were considered significant at α = 0.05 for contrasting the significance of factors and interactions, and 95% confidence intervals were obtained by the least significant difference (LSD) method.
Results
At baseline (first year of the study), the contrast of means in the frequency of occurrence between areas with and without predator control showed that the frequency of occurrence of European badger (F = 42.02, p = 0.0000) and stone marten (F = 137.52, p = 0.0000) was greater in the area without control. However, red fox (F = 5.14, p = 0.0244) showed a greater frequency of occurrence in the area with predator control. A separate analysis of areas (with and without predator control) revealed that there were no significant differences over the 3 years of study in the frequency of occurrence of European badger (F = 0.83, p = 0.4373) or red fox (F = 0:12, p = 0.8849) in PHAw. However, there were significant differences in the frequency of occurrence of European badger (F = 18.76, p = 0.0000) and red fox (F = 6.29, p = 0.0021) in PHAc. As regards frequency of occurrence in the third year with respect to the first year, European badger percentage increase was 135% and fox percentage reduction was 10%. Moreover, differences were observed in the frequency of occurrence of stone marten in PHAw (F = 21:32, p = 0.0000) and PHAc (F = 106.47, p = 0.0000), with a percentage increase of 45 and 330% respectively.
The reference point/horizon provided by the no-control area and the frequency of occurrence monitoring conducted in PHAc in different habitats () revealed firstly that European badger recovered frequency of occurrence in plots when cultivated land was dominant (92% second year); subsequently, it recovered plots where scrubland was dominant (72% second year and 93% third year), and, more slowly, it recovered plots where forest was dominant (27% first year, 47% second year and 68% third year). For stone marten, recovery occurred in the first instance in plots dominated by forest (98% third year), and then in plots dominated by scrubland (69% second year and 88% third year) and cultivated land (37% second year and 82% third year). In contrast, red fox maintained a presence in plots with a predominance of scrubland (106% third year) and slightly declined in plots with a predominance of forest (110% second year and 88% third year) and cultivated land (90% second year and 93% third year).
Figure 2. Evolution over time of the mean frequency of occurrence in different habitats, by two management conditions. Gray line = Private Hunting Areas with predator control. Black line = Private Hunting Areas without predator control. The bars represent 95% confidence intervals obtained by the method of least significant difference (LSD). 1st year = September 2008–August 2009, 2nd year = September 2009–August 2010, 3rd year = September 2010–August 2011.
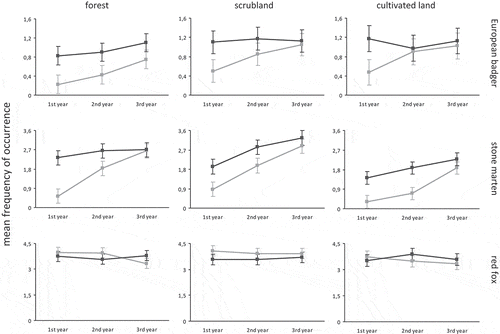
Season significantly affected the frequency of occurrence of stone marten (F = 12.52, p = 0.0000) and red fox (F = 4.57, p = 0.0035), but no significant differences in the frequency of occurrence of European badger (F = 1.75, p = 0.1555) according to season were observed. In a descriptive analysis, the seasonal gradient of frequency of occurrence decreased in the following order: summer > spring > winter ≈ autumn for European badgers; summer > spring ≈ winter > autumn for stone marten; winter > summer ≈ autumn > spring for red fox.
Discussion
In the first year of study, a comparison of frequency of occurrence revealed that non-selective predator control resulted in an increased frequency of occurrence of the most opportunistic species, the red fox. In contrast, the frequency of occurrence of European badger and stone marten decreased. The observed differences between PHAw and PHAc in frequency of occurrence are consistent with the species response to removal in the presence of competitors (Casanovas et al. Citation2012). The results of the simulation study with the competitor release effect model have shown that the factors that most affect population dynamics are removal intensity and strategy employed, intrinsic growth rate and immigration. According to this model, the species with the highest growth rate and greatest capacity for dispersal (red fox) maintains or increases its abundance (occupancy of space vacated by competitors), while the other species with a lower growth rate (European badger and stone marten) reduce their numbers. The moderate increase of red fox, in relation to the loss of other species, is explained by the fact that it is a game species: shooting foxes as a control measure is legal during hunting seasons.
The reference point/horizon provided by PHAw, and the percentage change in the frequency of occurrence in the PHAc obtained for the different species over time showed recovery by European badger and stone marten, and regression by red fox. The recovery of European badger and stone marten can be partly explained by abandonment of a predator control strategy, the greater availability of vacated space and the lower intraspecific competition in PHAc (Broekhuizen & Muskens Citation1984; Macdonald & Newman Citation2005). The red fox regression over time is consistent with the competitive hierarchy of the medium-sized carnivores studied. It has been observed that when interspecific interactions become aggressive, European badger displaces red fox by interference competition (Neal & Cheeseman Citation1996; Macdonald et al. Citation2004). Following the same argument, there is evidence of asymmetric cases of aggressive interactions and intraguild predation in which, under certain conditions, red fox displaces or preys on stone marten (Palomares & Caro Citation1999; Padial et al. Citation2002). This in turn may limit recovery of the stone marten population, as has already been proven for other small mustelids (Mulder Citation1990; Lindström et al. Citation1995). In the Serra de Montsant, this obstacle to the recovery of stone marten was not observed, because the increase in the frequency of occurrence of red fox in PHAc was very moderate.
Regarding the recovery of environmentally balanced habitats compared to the PHAw reference point, the European badger initially recovered the plots with a predominance of cultivated land, followed by plots with a predominance of scrubland and, more slowly, plots with a predominance of forest. The preference shown by the European badger for cultivated land can be explained in terms of the food resources that this habitat provides (Virgós et al. Citation2005). Scrubland, on the other hand, provides protection, refuge and some food (Molina-Vacas et al. Citation2009). The scarcity of food resources that forest provides may explain the lower preference shown for the use of these environments (Revilla et al. Citation2001). Stone marten initially recovered plots with a predominance of forest, followed by those with a predominance of scrubland and cultivated land. This faster recovery of the forest can be explained by the competitive advantage this environment offers this species. The climbing ability of stone marten differentiates it from the other two species studied, allowing it to access resources unavailable to the two other medium-sized carnivores (Larivière & Jennings Citation2009). The remarkable recovery of stone marten in all types of plots can be related to the near return to the PHAw reference environmental balance in the third year. The red fox remained slightly above the PHAw reference environmental balance in the plots where scrubland was predominant, whereas frequency of occurrence in plots dominated by cultivated land and forest was slightly reduced. In the Serra de Montsant, the preference shown by the three species for the use of scrubland is consistent with other studies, where scrubland or mosaic of scrub (tall shrubs, mixture of trees and abundant rocks) provide refuge and food for medium-sized carnivores (Mangas et al. Citation2008). The partial displacement of the red fox from cultivated land and forest is compatible with the recovery of the European badger (top competitor) and, to a lesser extent, of the stone marten (lower competitor) in these habitats (Barrull et al. Citation2013).
The effect of seasonality varies with species biology. Variations are explained mainly by the incorporation of the next generation of young individuals, the dispersion of family members, the seasonality of food, surplus faecal marking by males in mating periods and the weather conditions, all of which change their mobility habits (Henry et al. Citation1988; Artois Citation1989; Libois & Waechter Citation1991).
The results obtained in the Serra de Montsant show that non-selective predator control has been inadequate to reconcile the conservation of ecosystems with hunting. Non-selective control favours the red fox, which is the main consumer of small game species (Prigioni et al. Citation2008), and also reduces the population of European badger and stone marten, which are protected species. Thus, non-selective control has yielded results contrary to those desired. However, without predator control, European badger and stone marten recover over time and red fox declines. Consequently, to conserve biodiversity and exploit hunting resources effectively, it is more appropriate not to implement non-selective predator control measures. Alternatively, recent studies have demonstrated the effectiveness of selective trapping methods in some cases, when using specific models (Muñoz-Igualada et al. Citation2008, Citation2010) and when trappers receive proper training (Duarte et al. Citation2012). The use of trapping methods with adequate security devices can increase efficiency and selectivity, as well as help reduce mortality and injuries to target and non-target species (Frey et al. Citation2007; Duarte et al. Citation2012). The cost/benefit balance of this kind of selective control versus not performing control should improve management strategies. Regarding habitat recovery after non-selective control, the preferences observed in the Serra de Montsant are related to the ability to meet basic needs for food, shelter and competitor balance. The European badger occupies the highest level of the hierarchy as regards food and shelter. The stone marten occupies the lowest level of the hierarchy in the first instance, a characteristic that sets it apart from other competitors. The red fox occupies an intermediate level of the hierarchy, reducing numbers in all habitats as a result of the recovery of its competitors. The preferences observed in habitat use are consistent with the ability to satisfy basic needs (Virgós & Casanovas Citation1998; Virgós & Garcia Citation2002).
Acknowledgements
This research was supported in part by research project 2009 SGR 403, Departament d’Economia i Coneixement de la Generalitat de Catalunya.
References
- Anscombe FJ. 1948. The transformation of poisson, binomial and negative-binomial data. Biometrika 35:246–54. doi:10.1093/biomet/35.3-4.246.
- Artois M. 1989. Le renard roux (Vulpes vulpes Linnaeus, 1758). Encyclopédie des Carnivores de France. vol. 3. París: Société Française pour l’Étude et la Protection des Mammifères.
- Baker PJ, Harris S. 2006. Does culling reduce fox (Vulpes vulpes) density in commercial forests in Wales, UK? . European Journal of Wildlife Research 52:99–108. doi:10.1007/s10344-005-0018-y .
- Bang P, Dahlstrøm P. 2007. Animal tracks and signs. New York: Oxford University Press.
- Barrull J, Mate I. 2007. Fauna vertebrada del Parc Natural de la Serra de Montsant. Tarragona: Diputació de Tarragona.
- Barrull J, Mate I, Casanovas JG, Salicrú M, Gosàlbez J. 2011. Selectivity of mammalian predator control in managed hunting areas: An example in a Mediterranean environment. Mammalia 75:363–369. doi:10.1515/MAMM.2011.052.
- Barrull J, Mate I, Ruiz-Olmo J, Casanovas J, Gosàlbez JG, Salicrú M. 2013. Factors and mechanisms that explain coexistence in a Mediterranean carnivore assemblage: An integrated study based on camera trapping and diet. Mammalian Biology - Zeitschrift für Säugetierkunde doi:10.1016/j.mambio.2013.11.004.
- Beasom SL. 1974. Intensives short-term predator removal as a game management tool. Trasactions of the North American Wildlife and Natural Resources Conference 39:230–240.
- Beja P, Gordinho L, Reino L, Loureiro F, Santos-Reis M, Borralho R. 2009. Predator abundance in relation to small game management in southern Portugal: Conservation implications. European Journal of Wildlife Research 55:227–238. doi:10.1007/s10344-008-0236-1.
- Bender DJ, Tischendorf L, Fahrig L. 2003. Using patch isolation metrics to predict animal movement in binary landscape. Landscape Ecology 18:17–39. doi:10.1023/A:1022937226820.
- Blanco JC. 1986. On the diet, size and use of home range and activity patterns of a red fox in central Spain. Acta Theriologica 31:547–556.
- Broekhuizen S, Muskens G. 1984. Wat is er met de steenmarter, Martes foina (Erxleben, 1777) in Nederland aan de hand?. Lutra 27:261–273.
- Casanovas JG, Barrull J, Mate I, Zorrilla JM, Ruiz-Olmo J, Gosàlbez J, Salicrú M. 2012. Shaping carnivore communities by predator control: Competitor release revisited. Ecological Research 27:603–614. doi:10.1007/s11284-012-0931-y.
- Caut S, Casanovas JG, Virgós E, Lozano J, Witmer GW, Courchamp F. 2007. Rats dying for mice: Modelling the competitor release effect. Austral Ecology 32:858–868. doi:10.1111/j.1442-9993.2007.01770.x.
- Conner Mc, Labisky RF. 1985. Evaluation of radioisotope tagging for estimating abundance of raccoon populations. The Journal of Wildlife Management 49:326–332. doi:10.2307/3801525.
- Delibes-Mateos M, Díaz-Fernández S, Ferreras P, Viñuela J, Arroyo B. 2013. The role of economic and social factors driving predator control in small-game estates in central Spain. Ecology and Society 18:28. http://dx.doi.org/10.5751/ES-05367-18022810.5751/ES-05367-180228.
- Duarte J, Farfán MA, Fa JE, Vargas JM. 2012. How effective and selective is traditional Red Fox snaring?. Galemys 24:1–11. doi:10.7325/Galemys.2012.A01.
- Fanshawe JH, Ginsberg JR, Sillero-Zubiri C, Woodroffe R. 1997. The status and distribution of remaining wild dog populations. In: Woodroffe R, Ginsberg J, Macdonald D, editors. The African wild dog: Status survey and conservation action plan. Gland, Switzerland: IUCN. 11–57.
- Ferrari N. 1997. Eco-éthologie du blaireau européen (Meles meles L., 1758) dans le Jura suïsse: comparaison de deux populations vivant en milieu montagnard et en milieu cultivé de plaine. Ph. D. Thesis. Neuchâtel, France: Neuchâtel University.
- Fleishman E, Ray C, Sjögren-Gulve P, Boggs CL, Murphy DD. 2002. Assessing the roles of patch quality, area and isolation in predicting metapopulation dynamics. Conservation Biology 16:706–716. doi:10.1046/j.1523-1739.2002.00539.x.
- Frank LG, Woodroffe R. 2001. Behaviour of carnivores in exploited and controlled populations. In: Gittleman JL, Funk SM, Macdonald D, Wayne RK, editors. Carnivore conservation. Cambridge: Cambridge University Press. pp. 419–442.
- Frey SN, Conover MR, Cook G. 2007. Successful use of neck snares to live-capture red fox. Human-Wildlife Conflicts 1:21–23.
- Fritts SH, Mech D. 1981. Dynamics, movements, and feeding ecology of a newly protected wolf population in northwestern Minnessota. Wildlife Monographs 80:1–79.
- Gortázar C. 2008. Vulpes vulpes (Linnaeus, 1758). In: Palomo LJ, Gisbert J, editors. Atlas de los Mamíferos terrestres de España. Madrid: Dirección General de Conservación de la Naturaleza-SECEM-SECEMU. 277–279.
- Graham K, Beckerman AP, Thirgood S. 2005. Human–predator–prey conflicts: Ecological correlates, prey losses and patterns of management. Biological Conservation 122:159–171. doi:10.1016/j.biocon.2004.06.006.
- Hanski I. 2009. Metapopulations and spatial population processes. In: Levin S, editor. The Princeton guide to ecology. Princeton: Princeton University Press. 177–185.
- Harding EK, Doak DF, Albertson JD. 2001. Evaluating the effectiveness of predator control. The non-native red fox as a case study. Conservation Biology 15:1114–1122.
- Heinemeyer KS, Ulizio TJ, Harrison RL. 2008. Natural sings: Tracks ans scats. In: Long RA, MacKay P, Ray J, Zielinski W, editors. Noninvasive survey methods for carnivores. Washington: Island Press. 45–74.
- Henry C, Lafontaine L, Mouches A. 1988. Le blaireau (Meles meles Linnaeus, 1758). Encyclopèdie des Carnivores de France. vol. 7. París: Société Française pour l’Étude et la Protection des Mammifères.
- Heydon MJ, Reynolds JC. 2000a. Fox (Vulpes vulpes) management in three contrasting regions of Britain, in relation to agricultural and sporting interests. Journal of Zoology 251:237–252. doi:10.1111/j.1469-7998.2000.tb00607.x.
- Heydon MJ, Reynolds JC. 2000b. Demography of rural foxes (Vulpes vulpes) in relation to cull intensity in three contrasting regions of Britain. Journal of Zoology 251:265–276. doi:10.1111/j.1469-7998.2000.tb00609.x.
- Holekamp KE, Ogutu JO, Dublin HT, Frank LG, Smale L. 1993. Fission of a spotted hyena clan: Consequences of prolonged female absenteeism and causes of female emigration. Ethology 93:285–299. doi:10.1111/j.1439-0310.1993.tb01210.x .
- Hurlbert SH. 1984. Pseudoreplication and the design of ecological field experiments. Ecological Monographs 54:187–211. doi:10.2307/1942661.
- Jędrzejewski W, Sidorovich V. 2010. The art of tracking animals. Białowieża: Mammal Research Institute Polish Academy of Science.
- Kruuk H, Gorman M, Parrish T. 1980. The use of 65Zn for estimating populations of carnivores. Oikos 34:206–208. doi:10.2307/3544184.
- Larivière S, Jennings AP. 2009. Family Mustelidae (weasels and relatives). In: Wilson DE, Mittermeir RA, editors. Handbook of the mammals of the world. Vol 1. Carnivores, Barcelona: Lynx Edicions. 564–656.
- Libois R, Waechter A. 1991. La fouine (Martes foina Erxleben, 1777). Encyclopèdie des Carnivores de France. vol. 10. París: Société Française pour l’Étude et la Protection des Mammifères.
- Lindström ER, Brainerd SM, Helldin JO, Overskaug K. 1995. Pine marten–red fox interctions: a case of intraguild predation?. Annales Zoologici Fennici 32:123–130.
- Lozano J, Virgós E, Cabezas-Díaz S, Mangas JG. 2007. Increase of large game species in Mediterranean areas: Is the European wildcat (Felis silvestris) facing a new threat?. Biological Conservation 138:321–329. doi:10.1016/j.biocon.2007.04.027.
- Macdonald DW, Buesching CD, Stopka P, Henderson J, Ellwood SA, Baker SE. 2004. Encounters between two sympatric carnivores: Red foxes (Vulpes vulpes) and European badgers (Meles meles). Journal of Zoology 263:385–392. doi:10.1017/S0952836904005400.
- Macdonald D, Newman C. 2005. Flexibilidad ecológica en el tejón euroasiático: ganándose su camino hacia la sociabilidad. In: Virgós E, Revilla E, Mangas JG, Domingo-Roura X, editors. Ecología y conservación del tejón en ecosistemas mediterráneos. Málaga: SECEM. 173–196.
- Mangas JG, Lozano J, Cabezas-Díaz S, Virgós E. 2008. The priority value of scrubland habitats for carnivore conservation in Mediterranean ecosystems. Biodiversity Conservation 17:43–51. doi:10.1007/s10531-007-9229-8.
- McDonald RA, Harris S. 2002. Population biology of stoats Mustela erminea and weasels Mustela nivalis on game estates in Great Britain. Journal of Applied Ecology 39:793–805. doi:10.1046/j.1365-2664.2002.00757.x.
- Mills MGL, Hofer H. 1998. Hyaenas. Status survey ans conservation action plan. Gland: IUCN.
- Molina-Vacas G, Bonet-Arbolí V, Rafart E, Rodríguez-Teijeiro JD. 2009. Spatial ecology of European badger (Meles meles) in Mediterranean habitats of the North-Eastern Iberian Peninsula II: Habitat selection. Vie et Milieu 59:273–246.
- Mortelliti A, Boitani L. 2008. Interaction of food resources and landscape structure in determining the probability of patch use by carnivores in fragmented landscapes. Landscape Ecology 23:285–298. doi:10.1007/s10980-007-9182-7.
- Mulder JL. 1990. The stoat Mustela erminea in the Duch dune region, its local extintion, and posible cause: The arrival of the red fox Vulpes vulpes. Lutra 33:1–21.
- Muñoz-Igualada J, Shivik JA, Domínguez FG, González LM, Aranda A, Fernández M, Alves C. 2010. Traditional and new cable restraint systems to capture fox in central Spain. Journal of Wildlife Management 74:181–187. doi:10.2193/2008-603.
- Muñoz-Igualada J, Shivik JA, Domínguez FG, Lara J, González LM. 2008. Evaluation of cage-traps and cable restraint devices to capture red foxes in Spain. Journal of Wildlife Management 72:830–836. doi:10.2193/2007-198.
- Neal E, Cheeseman C. 1996. Badgers. London: T and A.D. Poyser.
- Norman GR, Streiner DL. 2008. Biostatistics: The bare essentials. Ontario: Norman and Streiner.
- Padial JM, Ávila E, Sánchez JM. 2002. Feeding habits and overlap among red fox (Vulpes vulpes) and stone marten (Martes foina) in two Mediterranean mountain habitats. Mammalian Biology - Zeitschrift für Säugetierkunde 67:137–146. doi:10.1078/1616-5047-00021.
- Palomares F, Caro TM. 1999. Interspecific killing among Mammalian Carnivores. The American Naturalist 153:492–508. doi:10.1086/303189.
- Peña D. 1994. Estadística. Modelos y Métodos. 2. Modelos lineales y series temporales. Madrid: Alianza Editorial.
- Prigioni C, Balestrieri A, Remonti L, Cavada L. 2008. Differential use of food and habitat by sympatric carnivores in the eastern Italian Alps. Italian Journal of Zoology 75:173–184. doi:10.1080/11250000701885521.
- Reig S. 2008. Martes foina (Erxleben, 1777). In: Palomo LJ, Gisbert J, editors. Atlas de los Mamíferos terrestres de España. Madrid: Dirección General de Conservación de la Naturaleza-SECEM-SECEMU. 305–307.
- Revilla E, Casanovas JG, Virgós E. 2008. Meles meles (Linnaeus, 1758). In: Palomo LJ, Gisbert J, editors. Atlas de los Mamíferos terrestres de España. Madrid: Dirección General de Conservación de la Naturaleza-SECEM-SECEMU. pp. 308–311.
- Revilla E, Palomares F, Fernández N. 2001. Characteristics, location and selection of diurnal resting dens by Eurasian badgers (Meles meles) in a low density area. Journal of Zoology 255:291–299. doi:10.1017/S0952836901001388.
- Reynolds JC, Goddard HN, Brockless MH. 1993. The impact of local fox (Vulpes vulpes) removal on fox populations at two sites in southern England. Gibier Faune Sauvage 10:319–334.
- Reynolds JC, Tapper SC. 1996. Control of mammalian predators in game management and conservation. Mammal Review 26:127–155. doi:10.1111/j.1365-2907.1996.tb00150.x.
- Sillero-Zubiri C. 2009. Family Canidae (dogs). In: Wilson DE, Mittermeir RA, editors. Handbook of the mammals of the world Carnivores. Vol 1. Barcelona: Lynx Edicions. pp. 352–446.
- Sillero-Zubiri C, Switzer D. 2004. Management of wild canids in human-dominated lanscapes. In: Sillero-Zubiri C, Hoffmann M, Macdonald DW, editors. Canids: Foxes, wolves, jackals and dogs. status survey and conservation action plan. Gland, Switzerland and Cambridge: IUCN/SSC Canid Specialist Group. 257–266.
- Smuts GL. 1978. Effects of population reduction on the travels and reproduction of lion in Kruger National Park. Carnivore 1:61–72.
- Virgós E. 2001a. Relative value of riparian woodlands in landscapes with different forest cover for medium-sized Iberian carnivores. Biodiversity and Conservation 10:1039–1049. doi:10.1023/A:1016684428664.
- Virgós E. 2001b. Role of isolation and habitat quality in shaping species abundance: A test with badgers (Meles meles L.) in a gradient of forest fragmentation. Journal of Biogeography 28:381–389. doi:10.1046/j.1365-2699.2001.00552.x.
- Virgós E, Casanovas JG. 1998. Distribution patterns of the stone marten (Martes foina Erxleben, 1777) in Mediterranean mountains of central Spain. Zeitschrift fur Säugetierkunde 63:193–199.
- Virgós E, García FJ. 2002. Patch occupancy by stone martens Martes foina in fragmented landscapes of central Spain: The role of fragment size, isolation and habitat structure. Acta Oecologica 23:231–237. doi:10.1016/S1146-609X(02)01142-6.
- Virgós E, Revilla E, Mangas JG. 2005. Factores que determinan la distribución y abundancia del tejón (Meles meles) a escal regional. In: Virgós E, Revilla E, Mangas JG, Domingo-Roura X, editors. Ecología y conservación del tejón en ecosistemas mediterráneos. Málaga: SECEM. 149–172.
- Virgós E, Travaini A. 2005. Relationship between small-game hunting and carnivore diversity in central Spain. Biodiversity and Conservation 14:3475–3486. doi:10.1007/s10531-004-0823-8.
- Webbon CC, Baker PJ, Harris S. 2004. Faecal density counts for monitoring changes in red fox numbers in rural Britain. Journal of Applied Ecology 41:768–779. doi:10.1111/j.0021-8901.2004.00930.x.
- Whitfield DP, McLeod DRA, Watson J, Fielding AH, Haworth PF. 2003. The association of grouse moor in Scotland with the illegal use of poisons to control predators. Biological Conservation 114:157–163. doi:10.1016/S0006-3207(03)00019-3.
- Woodroffe R. 2001. Strategies for carnivore conservation: Lessons from contemporary extinctions. In: Gittleman JL, Funk SM, Macdonald DW, Wayne RK, editors. Carnivore conservation. Cambridge: Cambridge University Press. pp. 61–92.