Abstract
The role of eyes was discussed in the beaked sea snake (Enhydrina schistosa) living in the open sea. Fine scanning electron microscope (SEM) images of the cellular arrangement were taken based on histological preparations of the retina. The retina possesses the usual layers of the vertebrate eye and is composed of only cone photosensitive cells lacking rods. Ophidian double cones were not found in the cell composition. Measurements of cone densities and dimensions showed their density in the ventral quadrant was more than that in the dorsal, temporal and nasal quadrants. The ventral specialized retina could be corresponding to surveillance of aerial predators. The presence of three-layered spectacle, single-type, large and dispersed photosensitive cells was evidence of the low visual capability of the eyes in E. schistosa.
Introduction
The beaked sea snake of subfamily Hydrophiinae is an aggressive, fully aquatic, true sea snake (Rasmussen Citation2001). This species, like the other sea snakes, is distributed in tropical and subtropical areas of the Indian and Pacific oceans (Volsøe Citation1939; Heatwole Citation1999; Rasmussen & Elmberg Citation2009). True sea snakes are viviparous and have many morphological adaptations to a fully marine life (Keogh Citation1998; Rasmussen et al. Citation2011), and are preyed upon by sea eagles and some predatory fishes such as tiger sharks (Heatwole & Cogger Citation1994). General anatomy (Samuel Citation1944; Kasturirangan Citation1951; Bergman Citation1956; Cogger Citation1975), venom (Tamiya Citation1975), feeding behaviors (Voris et al. Citation1978), reproduction and growth (Voris & Jayne Citation1979) of Enhydrinaschistosa have been studied. The eyes of vertebrates, despite possessing a similar overall appearance and structure, differ widely in retinal architecture and configuration, which is well related to ambient environment and life characteristics. A few studies were carried out on retinal morphology and vision mode of sea snakes by Hibbard and Lavergne (Citation1972) on Yellow bellied sea snake (Pelamis platurus), and Hart et al. (Citation2012) on Lapemis curtus and Acalyptophis peronii.
The purpose of the present study is to describe the morphology of the retina and the role of the visual sense in activities of E. schistosa.
Materials and methods
Ten adult specimens of E. schistosa ranging in length from 101 to 110 cm were collected in daylight from Iranian waters of the Gulf of Oman (Chabahar Bay, 60°28´22˝E, 25°17´23˝N). After species identification, the specimens were anesthetized with chloroform for 30 minutes and then were fixed as whole in Bouin’s solution for 48 hr (approximately five times the volume of snake body, and a change was made at the 24th hr) and later preserved permanently in 10% formalin solution to be transferred to the laboratory. A general description of eye size, relative to head, lens vs eye diameter, estimated focal distance and pupil measurement would improve knowledge of visual adaptations and define the species as microphthalmic.
The eyes were small (about 1/15 of head size), situated on the mid part of the head, and lifted above at the base of a slow narrowing snout, which ends bluntly. The pupil was large with little change in diameter. The lens was small compared to marine fishes, about 40% of vitreous space. The sclera was quite thick and protective.
The right eyes were dissected out and, after removing spectacles, processed for histological preparation. The eyes were not and could not be quarter-sectioned and hence, from the whole eye, 5-µm-thick sections were taken in coronal, sagittal and transverse (tangential) planes by Leica rotary microtome. The lens was soft enough to pass the blade through and histological sections were stained using the haematoxylin and eosin staining method. Sections were examined by light microscope and digital photographs were taken and assessed by TSView version 6.2.4.5. Also, a few known sections were prepared to be scanned by scanning electron microscope (SEM) (LEO 1430VP). Each selected section was glued on a 1 × 1 cm coverslip, deparaffinized with xylene and cleared with 90% methanol several times. The coverslip bearing the tissue was then mounted on a specimen stub, gold covered and scanned, and micrographs were taken. By this method, pre-studied histological tissue sections could be investigated further for magnified details. To assess the retinal regional visual ability, the eye was virtually divided into four quadrants. The cone number was counted and diameter measured in a 1-mm area of tangential sections. The mean was calculated and recorded. The spectacle was separately carbon dioxide (CO2) fractured and positioned on stub for scanning. A few micrographs were processed using Adobe Photoshop CS3 for suitable orientation and scale presentation.
Results
Each eye was surrounded by massive muscular tissue and protected by a spectacle in front of the cornea. The fractured spectacle revealed a triple-layered structure (). The lens was small, spherical and relatively soft.
All 10 usual layers of vertebrate eyes could be observed in the retina (). The pigment epitheliar layer was thin and possessed hexagonal-shaped epithelial cells. Photoreceptors were of only cone type and rods were absent. Cones were quite large, spaced in between and arranged in rows (, d). Two morphologically different cone types were identified: large single cones (LSC) and small single cones (SSC) (, c). A single cone was composed of three parts: (1) an outer segment close to the pigment layer, (2) an ellipsoid which was quite large and without oil droplets and (3) a myoid that was motionless and at minimum length (). The outer segment was about 2/3 the length of the ellipsoid () and covered by pigment bar granules in response to light (, ). The ellipsoid in large cones was distinct with a diameter of 6–10 µm. Small cones had a diameter of 2–4 µm and the outer segment did not reach the pigmented epithelial layer. Myoid, as a stalk of cone cells, was least distinguished. The inner ward of the ellipsoid was almost in contact with the outer limiting membrane. Cone nuclei were prominent and their numerical counts corresponded with the number of cone cells. Both outer and inner nuclear layers were prominent. The outer nuclear layer was composed of only nuclei of cone cells. There are certain connecting elements of unknown nature among the cones. We did not find either Mosaic or row cone arrangement.
Figure 2. Enhydrina schistosa. a: Light microscope micrographs showing 10 layers of retina. Notice the ribbons of pigment beads, choroid capillary and a row of cells composed of small and large cones. There is a distance between pigmented epithelial layer and the choroid, which is an artefact. b: Light microscope micrograph. Magnified cone cells showing their outer segment, large ellipsoid and short myoid. The nuclei of cones are prominent and the outer limiting membrane is well observed. The photoreceptor layer is devoid of rod cells. Large and small cones are present. c: Scanning electron microscope (SEM) micrograph. Tangential sectioning through near outer segment and outer segment of cones. Connecting elements are present between cones. Pigment bar granules cover the outer segment. A single small cone is situated among large cones. d: The position of cones to outer limiting membrane and pigmented epithelial layer is shown. Longitudinal section of cones reveals their inner structure. The connecting elements and pigment bar granules covering the outer segment of cone cell on the left are visible. CC: choroid capillary; R: ribbons of pigment; LSC: large single cone; PE: pigmented epithelium; SSC: small single cone; Ce: connecting elements; OS: outer segment; OLM: outer limiting membrane; Cn: cone nucleus; INL: inner nuclear layer; Pba: pigment bar granules; EL: ellipsoid.
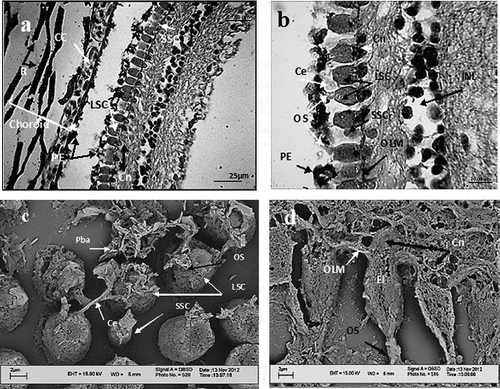
In the choroid, the pigment ribbons showed a contexture of innumerable, many-layered, bead-like granules (). On the other hand, there were rout-directed bar-like pigment granules () covering the cone outer segments that originated from pigmented epithelial cells.
Figure 3. a: Scanning electron microscope (SEM) micrographs, pigment ribbons in choroid. They are composed of pigment bead granules. b: Pigment bar granules are carried within the routs (canals), which are the microtubules of epithelial cells. The routs are shown by white outlining. Pbe: pigment bead granules; Pba: pigment bar granules; R: ribbons of bead granules; MY: myeloid; El: ellipsoid; OS: outer segment.
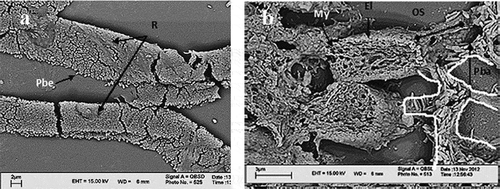
In the eye cup has been divided into four virtual quadrants, and cone cell number (density) and diameter are estimated. The ventral quadrant had the highest cone density (13,730/mm2 for LSC) and the temporal quadrant had the minimum cell number (9880/mm2 for LSC). The cell diameter was lowest in the ventral quadrant and highest in temporal quadrant.
Discussion
A 10-layered retina is a common feature in most vertebrates of all classes. Certain physiological and environmental factors affect the structural details of the retina not only in different classes of vertebrates but also even in smaller taxa. Snakes exhibit many unusual and unique features making them not only able to withstand harsh environmental conditions but also capable of living in different niches. Terrestrial or amphibious snakes are sensitive to infrared, vibration and noise making them fine hunters even during the night without depending much on vision.
In snakes, eyelids are fused to form transparent coverings over the corneas, known as spectacles. Sivak (Citation1977) proposed that spectacles themselves have an important refraction function eliminating the cornea as a refraction element. In Enhydrina schistosa, the almost-flat cornea has assisted the three-layered spectacles as a refractor, though a large and spherical lens also helps in light refraction. The retina of E. schistosa possessed only a single cone type of photoreceptors, as was observed in Lapimis curtus and Acalyptophis peronii (Hibbard & Lavergne Citation1972; Hart et al. Citation2012). This is quite different from reports on terrestrial or amphibious snakes. Sillman et al. (Citation2001) reported a duplex retina in Boa constrictor which was dominated by rods, and cones were of a single either small or large type. A similar result was obtained during an investigation of Python regius, as rods dominated the duplex retina which consisted of only 10% cone cells (Sillman et al. Citation1999).
Why do retinas in sea snakes not possess rod cells when these creatures are predators in dimly lit areas and able to feed even in total darkness (Voris et al. Citation1978)? Rod cells are highly light-sensitive but their flash response time is slow, therefore they can detect objects in the dark but are not suitable for detecting quick movements, whereas cones are less light-sensitive and their flash response time is fast so that cones mediate daylight vision and are more suitable to detect moving objects. On the other hand, the resolving power of an eye can be increased by increasing the density of photoreceptors, i.e. the retinal gain (Shand Citation1997). Further improvement in resolution at a given eye size can be obtained at the level of the retina by having an area of high cone density. Such an area of high cone density corresponds with the main visual axis (Tamura & Wisbey Citation1963; Pankhurst Citation1989; Browman et al. Citation1990). The presence of only cone cells in E. schistosa brings expectations, if not of fine vision then an at least normal, daylight-sensitive one. But the beaked sea snake does not appear to possess a vivid visual sense.
Voris et al. (Citation1978), during an investigation of feeding behavior in E. schistosa, observed that the beaked sea snake did not follow prey directly and never struck at prey from a resting position, but during the course of its cruising the snake might encounter and strike a fish. He also mentioned that if prey was dropped, the snake could not pick it up but would snap in all directions trying to relocate it. A similar result was obtained for the sea snake Aipysurus laevis when a live swimming fish was ignored by the snake during feeding (Hart et al. Citation2012). This is evidence that vision was not important in prey detection and sea snakes could carry out successful predation in total darkness (Voris et al. Citation1978). To assess the visual acuity of sea snakes, the retinal topography was studied (Hughes Citation1977; Hart et al. Citation2012). Anatomical estimation was performed by enumeration of ganglion cells and neurons. Temporal and Nasal areas were recognized as specialized areas, notwithstanding the number of photoreceptors. In E. schistosa of the present study, the number of cones was taken as an estimating parameter of the topographic pattern and visual acuity. Photoreceptors can be compared to the number of pixels in the sensor of a photographic camera: the greater the number of pixels, the sharper the image taken would be. The investigation of fishes also provides similar results where good resolution is demanded of the high density of visual cells (Levine & MacNichol Citation1979; Nicol Citation1989). Increased spatial resolving power is mediated by tightly packed arrays of small photoreceptors. However, the maximum diameter for a receptor outer segment even in a small eye is approximately 1 µm (Collin & Shand Citation2003). Below this diameter, the photoreceptors fail to act as wave guides (Kirschfeld Citation1976; Land Citation1999). In E. schistosa, the outer segment diameter is quite small (about 2 µm) and, therefore, cones would not be efficient enough to receive sufficient light. The large inner segment may be a source of glycogen and energy (Collin & Shand Citation2003) or certain products of mitochondria functioning as a short-wave absorbing cut-off filter and tuning the incoming light before it strikes the outer segment (Avery & Bowmaker Citation1982). In E. schistosa, cones did not show protractile myoid and thus do not participate in the retinomotor response in the presence of light. In spite of the non-protractile myeloid, the outer segments of cones were surrounded by pigment bar granules which occur in response to light intensity as a part of the retinomotor response (). In fishes, various sizes of eyes, the position of the eye on the head, the structure of the cornea (thickness and curvature) and lens (shape and diameter), morphology of the retina, the visual neural pathway, types of cone cells, eye movement and retinomotor response are greatly influenced by water depth, turbidity, flow speed, temperature, feeding habits and food availability, bringing about different levels of visual fields and acuity (Ota et al. Citation1999; Collin & Shand Citation2003). As sea snakes live, feed and in some species reproduce in the open sea (E. schistosa), the presence of a single type of photoreceptors, a motionless eye, the absence of protractile myoid and spectacle covering, make it difficult to expect the visual sense to be very effective and confront ecological factors that may affect the spectral tuning of snake visual pigments (Hart et al. Citation2012). Cone activity, though slow, does not seem to be affected by light intensity, tidal movement (McCosker Citation1975; Voris & Voris Citation1983) and water turbidity (Loew & Lythgoe Citation1978; Levine & MacNichol Citation1982). Such a degree of low-power vision might not be very efficient in avoiding predators, or quick prey identification; however, the cone distribution pattern provides a clearer part of an image compared to others, as presented in planktivoros Osmerus eperlanus (Reckel et al. Citation2003). Higher cone density in the ventral quadrant might give the animal an extension of the upward vision and awareness against aerial predators such as sea eagles (Rasmussen & Elmberg Citation2009; Hart et al. Citation2012).
Conclusion
Based on the eye and vision characteristics in E. schistosa including small eyes, the absence of rods, limited types of cones, the small diameter of cone outer segments, the presence of spectacles and the low number of photoreceptors, it is concluded that eyes function at the level of light-intensity recognition and provide an ambiguous image. Vision therefore is assessed as an auxiliary sense during chore activities.
Acknowledgements
The authors are grateful to Mr. Afsari, the director of Sistan and Baluchestan Environment State Office, and Mr. Hoseini, the director of Chabahar Marine Environment Center, and also Mr. Soltanpur, Mr. Delavar, Mr. Najafi and Mrs. Foroghi for providing laboratory facilities and the possibility to access the specimens. The present work was supported financially by the research deputy, University of Guilan.
References
- Avery JA, Bowmaker JK. 1982. Visual pigments in the four – eyed fish Anableps anableps. Nature 298:62–64. doi:10.1038/298062a0.
- Bergman RAM. 1956. L’anatomie de Enhydrina schistosa D. Archives Néerlandaises de Zoologie 11:127–142. doi:10.1163/036551656X00111.
- Browman HI, Gordon WC, Evans BI, O’Brien WJ. 1990. Correlation between histological and behavioral measures of visual acuity in a zooplanktivorous fish, the white crappie (Pomxis annularis). Brain, Behavior and Evolution 35:85–97. doi:10.1159/000115858.
- Cogger HG. 1975. Sea snakes of Australia and New Guinea. In: Dunson WA, editor. The biology of the sea snakes. Baltimore: University Park Press. pp. 59–139.
- Collin SP, Shand J. 2003. Retinal sampling and the visual field in fishes. In: Collin SP, Marshall NJ, editors. Sensory processing in aquatic environments. New York: Springer-Verlag. pp. 139–169.
- Hart NS, Coimbra JP, Collin SP, Westhoff G. 2012. Photoreceptor types, visual pigments, and topographic specializations in the retinas of hydrophiid sea snakes. The Journal of Comparative Neurology 520:1246–1261. doi:10.1002/cne.22784.
- Heatwole H. 1999. Sea snakes. Sydney: University of New South Wales Press.
- Heatwole H, Cogger H. 1994. Sea snakes of Australia. In: Gopalakrishnakone P, editor. Sea snake toxicology. Singapore: Singapore University Press. pp. 167–205.
- Hibbard E, Lavergne J. 1972. Morphology of the retina of the sea-snake, Pelamis platurus. Journal of Anatomy 112:125–136.
- Hughes A. 1977. The topography of vision in mammals of contrasting life style: Comparative optics and retinal organization. In: Crescitelli F, editor. The visual system in vertebrates. Berlin: Springer.
- Kasturirangan LR. 1951. Placentation in the sea snake, Enhydrina schistosa (Daudin). Proceedings of the Indian Academy of Sciences, Sec. B 34:1–32.
- Keogh JS. 1998. Molecular phylogeny of elapid snakes and a consideration of their biogeographic history. Biological Journal of the Linnean Society 63:177–203. doi:10.1111/j.1095-8312.1998.tb01513.x.
- Kirschfeld K. 1976. The resolution of lens and compound eyes. In: Zettler F, Weiler R, editors. Neural principles in vision. Berlin: Springer-Verlag.
- Land MF. 1999. Visual optics: The sand lance eye breaks all the rules. Current Biology 9:R286–R288. doi:10.1016/S0960-9822(99)80180-8.
- Levine JS, MacNichol EF Jr. 1979. Visual pigments in teleost fishes: Effects of habitat, microhabitat and behavior on visual system evolution. Sensory Processes 3:95–131.
- Levine JS, MacNichol EF Jr. 1982. Color vision in fishes. Scientific American 246:140–149. doi:10.1038/scientificamerican0282-140.
- Loew ER, Lythgoe JN. 1978. The ecology of cone pigments in teleost fishes. Vision Research 18:715–722. doi:10.1016/0042-6989(78)90150-5.
- McCosker JE. 1975. Feeding behavior of Indo-Australian Hydrophiidae. In: Duson WA, editor. The biology of sea snakes. Baltimore: University Park Press. pp. 217–232.
- Nicol JAC. 1989. The eyes of fishes. Oxford: Clarendon Press.
- Ota D, Francese M, Ferrero EA. 1999. Vision in the grass goby, Zosterisessor ophiocephalus (Teleostei, Gobiidae): A morphological and behavioural study. Italian Journal of Zoology 66:125–139. doi:10.1080/11250009909356247.
- Pankhurst NW. 1989. The relationship of ocular morphology to feeding modes and activity periods in shallow marine teleosts from New Zealand. Environmental Biology of Fishes 26:201–211. doi:10.1007/BF00004816.
- Rasmussen AR. 2001. Sea snakes. In: Carpenter KE, Niem VH, editors. FAO species identification guide for fishery purposes, the living marine resources of the Western Central Pacific. Volume 6. Rome: F.A.O. pp. 3987–4008.
- Rasmussen AR, Elmberg J. 2009. ‘Head for my tail’: A new hypothesis to explain how venomous sea snakes avoid becoming prey. Marine Ecology 30:385–390. doi:10.1111/j.1439-0485.2009.00318.x.
- Rasmussen AR, Murphy JC, Ompi M, Gibbons JW, Uetz P. 2011. Marine reptiles. PLOS – One 6:e27373. doi:10.1371/journal.pone.0027373.
- Reckel F, Hoffmann B, Melzer RR, Horppila J, Smola U. 2003. Photoreceptors and cone patterns in the retina of the smelt Osmerus eperlanus (L.) (Osmeridae: Teleostei). Acta Zoologica (Stockholm). 84:161–170. doi:10.1046/j.1463-6395.2003.00142.x.
- Samuel M. 1944. Studies on the corpus luteum in Enhydrina schistosa (Daudin) and Hydrophis cyanocinctus (Daudin) of the Madras coast. Proceedings of the Indian Academy of Sciences, B 20:143–174.
- Shand J. 1997. Ontogenetic changes in retinal structure and visual acuity: A comparative study of coral-reef teleosts with differing post-settlement lifestyles. Environmental Biology of Fishes 49:307–322. doi:10.1023/A:1007353003066.
- Sillman AJ, Carver JK, Loew ER. 1999. The photoreceptors and visual pigments in the retina of boid snake, the ball python (Python regius). Journal of Experimental Zoology 202:1931–1938.
- Sillman AJ, Johnson JL, Loew ER. 2001. Retinal photoreceptors and visual pigments in Boa constrictor imperator. Journal of Experimental Zoology 290:359–365. doi:10.1002/jez.1076.
- Sivak JG. 1977. The role of the spectacle in the visual optics of the snake eye. Vision Research 17:293–298. doi:10.1016/0042-6989(77)90093-1.
- Tamiya N. 1975. Sea snake venoms and toxin. In: Dunson WA, editor. The biology of the sea snakes. Baltimore: University Park Press. pp. 385–415.
- Tamura T, Wisbey SJ. 1963. The visual sense of pelagic fishes especially the visual axis and accommodation. Bulletin of Marine Science of the Gulf and Caribbean 13:433–448.
- Volsøe H. 1939. The sea-snakes of the Iranian Gulf and the Gulf of Oman. Danish Scientific Investigation in Iran 1:9–45.
- Voris HK, Jayne BC. 1979. Growth, reproduction and population structure of a marine snake, Enhydrina schistosa (Hydrophiidae). Copeia 1979:307–318. doi:10.2307/1443419.
- Voris HK, Voris HH. 1983. Feeding strategies in marine snakes: An analysis of evolutionary, morphological, behavioral and ecological relationships. American Zoologist 23:411–425.
- Voris HK, Voris HH, Liat LB. 1978. The food and feeding behavior of a marine snake, Enhydrina schistosa (Hydrophiidae). Copeia 1978:134–145. doi:10.2307/1443834.