Abstract
The 27 extant species of the family Sphyraenidae represent one of the major groups of piscivorous teleost fishes in tropical and subtropical marine waters. In spite of their ecological importance, currently, no phylogenetic hypothesis is available for this group, and we do not know the tempo of evolution of this clade. In this study, we used a supermatrix approach to assemble a dataset of three mitochondrial loci for 20 sphyraenid species, and time-calibrated this new phylogeny. Our study supports the existence of three main groups of barracudas, which we labelled the “S. barracuda” group, the “S. obtusata” group and the “S. sphyraena” group. The timetree indicates a Late Paleocene age (~57 Ma) for the origin of the groups, and a Middle Eocene (~45 Ma) timing for the beginning of the radiation of extant lineages. Most extant species appear to belong to phylogenetic lineages dating to the Miocene (~5 to 23 Ma). Our study reveals multiple shifts between coral reef-associated and non-reef (usually more pelagic) habitats, as well as two independent origins of large body size within this group.
Introduction
Much effort has been devoted during the past 10 years to investigate the tempo of diversification of many coral reef-associated fish lineages such as moray eels, snake eels and allies (Reece et al. Citation2010; Santini et al. Citation2013b); pufferfishes, triggerfishes and allies (Alfaro et al. Citation2007; Dornburg et al. Citation2008, Citation2011; Santini et al. Citation2013c, Citation2013d, Citation2013e); wrasses and parrotfishes (Alfaro et al. Citation2009; Cowman et al. Citation2009; Kazancioglu et al. Citation2009); surgeonfishes (Klanten et al. Citation2004; Sorenson et al. Citation2013); butterflyfishes (Cowman & Bellwood Citation2011); damselfishes (Cowman & Bellwood Citation2011; Frédérich et al. Citation2013); cardinalfishes (Cowman & Bellwood Citation2011); and requiem sharks (Sorenson et al. Citation2014). While our understanding of the timing of origin and evolution of reef-associated fish lineages has been greatly increased by these studies, the vast majority of species within these groups tend to be durophagous forms feeding on benthic macroinvertebrates such as crustaceans or shellfish, herbivores or planktivores, with a few lineages having evolved highly specialized diets such as corallivory in butterflyfishes. Very few members of these lineages, with the exception of eels and requiem sharks, feed mostly or exclusively on other fishes (and even many species of moray eels feed largely on cephalopods or crustaceans), and very little is currently known about the time of origin and radiation of the major piscivorous teleost groups, such as groupers, jacks and pompanos, snappers and barracudas.
The 27 extant species of barracudas (family Sphyraenidae) are one of the major groups of large coastal piscivores in tropical and subtropical marine habitats. Most barracudas are found in coastal areas, often in close association with scleractinian coral reefs, but several species have a more pelagic distribution and are known to cross large tracts of open water (Daly-Engel et al. Citation2012). Barracudas possess an elongated, fusiform body and a large mouth, with a lower jaw that is usually longer than the upper one; both jaws carry many sharp and fang-like teeth of different sizes. Most species range in size from 60 to 150 cm, even though a few, such as Sphyraena barracuda (Edwards, 1771), S. guachancho (Cuvier, 1829) and S. afra (Peters, 1844), can reach lengths of ~2 m (). Barracudas tend to be opportunistic predators, with a hunting technique that relies on ambushing a prey thanks to their ability to sustain bursts of high swimming speed over short distances. Most species are known to form schools as either juveniles or adults, with schools containing hundreds of individuals in some cases. Some species, however, tend to be solitary or only live in small groups, and adult individuals of some schooling species can sometimes live solitary lives (Froese & Pauly Citation2014). Adult barracudas are one of the few teleost groups known for unprovoked attacks on humans, especially swimmers and divers that carry shiny metal objects, and in some Indo-Pacific islands, they are as feared as sharks. In spite of their negative reputation, barracudas are popular with sport-fishermen and, being a popular food fish in many tropical countries, are known to be responsible for several cases of ciguatera poisoning, a phenomenon caused by dinoflagellate toxins that tend to accumulate in predators at the top of the food chain in tropical marine ecosystems.
Table I. List of sphyraenid species included in this study with data on total length (TL), habitat type (reef, non-reef) and biogeographic region.
Currently, very little is known about the evolutionary history of sphyraenid fishes. Traditionally, barracudas were thought to be related to tunas, snake mackerels and allies, and, on the basis of selected morphological characters, they have been suggested as the sister group to all remaining members of the perciform suborder Scombroidei (Johnson Citation1986). A number of molecular studies, however, have conclusively shown that the traditional Scombroidei is not a monophyletic group (i.e., Betancur-R et al. Citation2013; Miya et al. Citation2013; Near et al. Citation2013; and many others), and that the Sphyraenidae belong within a group that includes jacks and pompanos (Carangidae), flatfishes (Pleuronectiformes), and a number of other lineages previously assumed to be completely unrelated (e.g., archerfishes, family Toxotidae; and nile perches and snooks, family Centropomidae). To date, however, no comprehensive phylogenetic study exists for barracudas. The only published peer-reviewed molecular studies to include more than four of the 27 extant species are the molecular taxonomic study of Doiuchi and Nakabo (Citation2007) on the Sphyraena obtusata group, based on ~800 base pairs (bp) of the mitochondrial cytochrome b (Cytb) gene for seven species, and the phylogeographic study of Daly-Engel et al. (Citation2012) of the great barracuda (S. barracuda), which included a ~630 bp fragment of Cytb for 10 species of Sphyraena. Rabosky et al.’s (Citation2013) megaphylogeny of the ray-finned fishes also included 14 sphyraenid species, but had ~90% missing data, with most barracuda species only represented by two or three of the 13 loci used in that study.
For this reason, we used a supermatrix approach to assemble a dataset containing sequence data for three mitochondrial loci for 20 species of sphyraenid fishes, and two outgroups. Our molecular phylogeny was then calibrated using the oldest sphyraenid fossil, in order to produce a molecular timetree that could allow us to investigate the tempo of evolution of barracudas.
Materials and methods
Phylogenetic analyses
We used the PhyLoTa browser version 1.5 (Sanderson et al. Citation2008), based on GenBank release 194 (15 February 2013) to determine which among the nuclear and mitochondrial genes commonly used in vertebrate phylogenetics included the highest number of sphyraenid species. Sequences for 16, 13 and nine species were available in GenBank (as of March 2014) for the mitochondrial markers cox1, Cytb, and 16s, respectively, with no other gene being available for more than four species. We thus downloaded all sphyraenid sequences found in GenBank for these three loci, as well as for two species of Centropomidae, which several recent studies have shown to be closely related to barracudas (Betancur-R et al. Citation2013; Near et al. Citation2013). We aligned each locus using MUSCLE (Multiple Sequence Comparison by Log Expectation; Edgar Citation2004), checked them by eye and trimmed the 5’ and 3’ ends to minimize the amount of missing data using MEGA (Molecular Evolutionary Genetics Analyses) 5 (Tamura et al. Citation2011). We ran gene tree analyses in MEGA 5 (Tamura et al. Citation2011) using the neighbor-joining method to test for potentially misidentified sequences and to identify whether disagreement exists among the various gene trees. We retained the longest sequence when multiple sequences were available for one species (Table S1). We concatenated the three aligned loci using Mesquite 2.75 (Maddison & Maddison Citation2011). Our final data matrix consisted of 630 bp for cox1, 792 bp for Cytb and 549 bp for 16s, for a total of 1971 nucleotides.
We selected the best-fitting models of sequence evolution from the candidate pool of models that can be utilized in MrBayes 3.2 (Ronquist et al. Citation2012) using corrected Akaike Information Criterion scores (AICc) with jModelTest (Posada Citation2008) without including the proportion of invariant sites parameter (Yang Citation2006). The generalized-time reversible model plus gamma (GTR + G) model was selected as the most appropriate model for all loci.
We ran Bayesian analyses using MrBayes 3.2 (Ronquist et al. Citation2012) after each gene partition had been assigned the model selected by jModelTest (Posada Citation2008). We ran two analyses each for 10 million generations with four chains (three heated, one cold), sampling every 1000 generations. We visually inspected the trace files in Tracer 1.5 (Drummond et al. Citation2012) to verify that the chains had reached convergence, and after discarding the first million generations as burn-in, we combined the remaining trees to obtain a 50% majority rule consensus tree.
We used BEAST (Bayesian Evolutionary Analysis Sampling Trees) 1.8 (Drummond et al. Citation2012) to infer a relaxed clock molecular timetree. We used †Sphyraena bolcensis, a likely stem sphyraenid known from the 50-Ma Ypresian of Monte Bolca (Italy) to assign a minimum age to the split between sphyraenids and their sister group, and the age of the Nardò deposits from Southern Italy, which contain some of the oldest remains of the percomorph fishes sensu Betancur-R et al. (Citation2013) and Near et al. (Citation2013), to establish the soft upper bound (offset 83.5). We ran two sets of analyses, one in which all three loci were individually partitioned and assigned the GTR + G model selected by jModelTest and another in which all mitochondrial loci were treated as a single partition. We used an uncorrelated lognormal (UCLN) model, assigned a birth-death prior to the rates of cladogenesis and used an exponential prior on the calibration point. For each partition scheme, we ran two sets of analyses with 10 million generations each, and sampled every 1000 generations. We inspected the chains for convergence in Tracer 1.5 (Drummond et al. Citation2012). We found that the effective sample size (ESS) for all parameters was well over 200, indicating the convergence of the various chains, removed the first 10% of the trees as burn-in and used LogCombiner to merge the remaining trees, and TreeAnnotator (Drummond et al. Citation2012) to obtain a timetree.
Comparative phylogenetic analyses
We used Fishbase (Froese & Pauly Citation2014) to obtain information on maximum body size (reported as total length, TL, the distance between tip of snout and longest lobe of caudal fin), habitat association (coral reef vs. non-reef) and biogeography (), and used R version 3.02 (R Development Core Team Citation2014) and Mesquite (Maddison & Maddison Citation2011) to investigate the evolution of these features. We used Mesquite to map the evolution of habitat association, and biogeographic distribution on a timetree using both a parsimony and a maximum likelihood approach, and Analysis of Phylogenetics and Evolution (APE) (Paradis et al. Citation2004) to reconstruct the ancestral state of body size using a maximum likelihood reconstruction approach with an MK1 model.
Results
Phylogenetic analysis and divergence estimate
The MrBayes analysis recovered high support for the monophyly of the Sphyraenidae with a posterior probability (PP) of 1.0 (). All sphyraenid species included in our study fall within three distinct clades, which appear in a polytomy. The first one includes a series of lineages branching off sequentially: Sphyraena jello (Cuvier, 1829), followed by S. putnamae (Jordan & Seale, 1905), S. barracuda, S. ensis (Jordan & Gilbert, 1882) and S. guanchancho. The second clade includes Sphyraena forsteri (Cuvier, 1829), S. obtusata (Cuvier, 1829), S. flavicauda (Rüppell, 1838), and finally a polytomy with S. chrysotaenia (Klunzinger, 1884), S. iburiensis (Doiuchi & Nakabo, 2005) and S. pinguis (Günther, 1874). The third clade includes a lineage formed by Sphyraena africana (Gilchrist & Wardlaw 1910), S. acutipinnis (Day, 1876) and S. japonica (Bloch & Schneider, 1801), sister to a group composed of S. argentea (Girard, 1854) + S. viridensis (Cuvier, 1829), S. helleri (Jenkins, 1901), S. idiastes (Heller & Snodgrass, 1903), S. picudilla (Poey, 1860) and S. sphyraena (Linnaeus, 1758). All three sphyraenid clades, as well as most nodes within each of these, are supported by PP of at least 0.91.
Figure 1. Bayesian phylogenetic hypothesis inferred with MrBayes 3.2. Numbers below branches indicate posterior probability support for each clade.
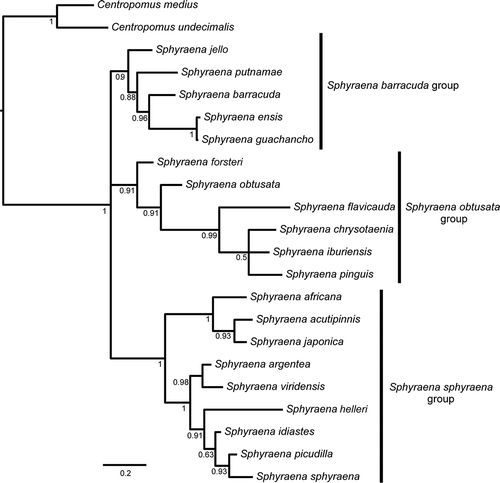
The topologies of the BEAST timetrees obtained from analyses under the different partition schemes, as well as the divergence time estimates for the various nodes on the trees, are almost identical. For this reason, we will show and discuss only the results of the analysis performed when all three loci are considered as a single partition. The timetree topology () is almost identical to the topology of the MrBayes analyses. The only differences are that rather than being in the polytomy with the other two clades, the clade including Sphyraena sphyraena is the sister taxon to the remaining barracudas, and within the Sphyraena barracuda clade, S. iburiensis is sister to S. flavicauda. The timing of the radiation of barracudas indicates a stem age of 57 Ma, suggesting that the split with the sister taxon occurred slightly before the Paleocene/Eocene boundary, and a Middle Eocene age for the origin of the crown sphyraenids (45 Ma, 26–64 Ma 95% highest posterior density, or HPD). The crown age of two of the three clades dates to the Oligocene: the Sphyraena sphyraena clade is 28 Ma (14–44 Ma 95% HPD), while the Sphyraena obtusata group is 31 Ma (16–48 Ma 95% HPD). The third clade, the Sphyraena barracuda group, dates to the Early Miocene (21 Ma, 8–36 Ma 95% HPD). Virtually all the nodes, even within the two older clades, appear to have originated during the Miocene (23.5–5.3 Ma), suggesting that the extant barracuda species belong to relatively old lineages. The only exceptions with Pliocene or Pleistocene age are the Sphyraena guachancho vs. S. ensis split, dated at just 1 Ma (0.1–3 Ma 95% HPD), and the split between S. barracuda and S. picudilla, dated at 4 Ma (0.9–8 Ma 95% HPD).
Body size and habitat
The ancestral state reconstruction of body size on the BEAST chronogram reveals that barracudas with large body size evolved at least twice: once within the Sphyraena barracuda group and once within the S. sphyraena group, with most species in these two groups being close to or over 1 m in length, and some taxa reaching 2 m. The third clade (the Sphyraena obtusata group) is composed of smaller species, including the smallest Sphyraena species (Sphyraena iburiensis and S. chrysotaenia) (Figure S1). The maximum parsimony and maximum likelihood mapping of the habitat association (reef vs. non-reef) shows multiple transitions between reef and non-reef habitats ( and S2). The largest species in our study, Sphyraena barracuda and S. guanchancho, as well as S. afra which is not included in our sampling, are all non-reef species. With the exception of the Sphyraena sphyraena clade, which includes mostly species found in the Atlantic Ocean or the Eastern Pacific, most extant species of barracudas are found in the Indo-Western Pacific (Figure S3). Even though several barracuda species have wide geographic distributions, the only true circumglobal species is the great barracuda (S. barracuda), which is known from all the major oceans as well as the Mediterranean Sea. Interestingly, this species had already been found to have rates of dispersal more similar to those of pelagic, as opposed to reef-affiliated, species, and the young age of its lineage indicates a dispersal potential that is likely much higher than that of other barracudas.
Discussion
Phylogeny of sphyraenids
Our study produced the most comprehensive molecular phylogeny of Sphyraenidae, one of the major groups of piscivores on coral reefs, and the only one so far that has been time-calibrated using a sphyraenid fossil. Very little work has so far been published on sphyraenid relationships: Doiuchi and Nakabo (Citation2007), on the basis of a 799 bp fragment of the mitochondrial cytochrome b gene, found that Sphyraena iburiensis, S. obtusata and S. pinguis formed a well-supported group to the exclusion of four additional sphyraenid species: S. africana, S. forsteri, S. helleri and S. japonica. Our analyses also recovered a clade formed by Sphyraena iburiensis, S. obtusata and S. pinguis, even if this grouping also includes other species missing from the Doiuchi and Nakabo (Citation2007) study. Daly-Engel et al. (Citation2012) analyzed a ~630 bp fragment of Cytb and produced an unrooted tree of Sphyraena in which several subclades congruent with our topology can be observed: S. pinguis, S. iburiensis and S. obtusata; S. africana and S. japonica; S. viridensis and S. sphyraena. Rabosky et al. (Citation2013) included 14 species of sphyraenids in their ray-finned fish megaphylogeny study, and recovered a sphyraenid topology that appears to be fairly different from that of our study. Sphyraena putnamae is the sister group to all other sphyraenids in the maximum likelihood tree that was used also for the time calibration of the ray-finned fish tree. Some of the same clades can be recognized in both the Rabosky et al. (Citation2013) and our tree, in spite of an only-partially-overlapping sampling (e.g., the above-mentioned Sphyraena iburiensis, S. obtusata and S. pinguis clade or the S. viridensis, S. sphyraena and S. idiastes clade). In general, however, the Rabosky et al. (Citation2013) topology appears to be much more pectinate than our tree, and little support is present for most nodes. The Rabosky et al. (Citation2013) timetree was based on a maximum likelihood analysis of a 13-gene dataset that included almost 8000 species of ray-finned fishes. While the sphyraenid sampling included a much greater number of species than that found in any other study of teleost phylogenetics, the high proportion of missing data (~90% across the entire matrix) makes us confident that the topology presented in this study is a more realistic representation of the correct barracuda tree of life. Most sphyraenid subclades of our tree also appear to match groups discussed by De Sylva (Citation1984) in his study of sphyraenid development. De Sylva (Citation1984) scored a number of morphological traits across extant species of sphyraenids, including presence and type of gill rakers, type of scales, position of the first dorsal fin, type of pigmentation, teeth shape and presence of a fleshy knob on the lower jaw. Several characters were scored in both adults and larvae (i.e., fleshy knob on jaws, pigmentation), while others were only recorded for adults. Da Sylva’s (Citation1984) “diagram of relationships” (his Fig. 283, p. 534), although not obtained through a formal cladistic analysis, suggests the existence of five groups. All of these largely match groups found in our analyses, when the partially overlapping sampling of the two studies is accounted for: the S. pinguis + S. flavicauda + S. chrysotaena + S. obtusata subclade is supported by, among other features, the presence of two gill rakers and of one or two stripes of lateral pigmentation in adults; the S. acutipinnis + S. africana + S. japonica subclade is supported by five features, including the presence of a single gill raker; the S. idiastes + S. argentea + S. picudilla + S. viridensis + S. sphyraena subclade is supported by six traits, including the lack of lateral pigmentation markings on the body of adults and the presence of conical, as opposed to flattened, teeth; the S. ensis + S. guachancho + S. putnamae + S. jello + S. barracuda subclade, which matches two of De Sylva’s (Citation1984) groups, is supported by the absence of gill rakers and the presence of the first dorsal fin behind the pelvis.
In regard to the tempo of sphyraenid evolution, our study differs from the Rabosky et al. (Citation2013) study in inferring a much younger age for the origin of the crown sphyraenids; our relaxed Bayesian clock analysis hypothesized an age of ~45 Ma vs. the ~62 Ma of the Rabosky et al. (Citation2013) study based on penalized likelihood. Almost all nodes in the Rabosky et al. (Citation2013) tree are inferred to have an age older than the Miocene, and the younger split in the tree (that between Sphyraena barracuda and S. guachancho) appears to be ~14 Ma. The ages found in our study, while younger than those of the Rabosky et al. (Citation2013) megaphylogeny, appear to closely match the pattern of barracuda evolution observed in the fossil record, where remains of barracudas are relatively common in deposits of Middle Eocene or younger age. As previously mentioned, the earliest evidence of the group is the Ypresian (about 50 Ma) putative stem sphyraenid Sphyraena bolcensis from Monte Bolca, Italy. This taxon was described and illustrated by Agassiz (Citation1833–1844) in his monumental Les recherches sur les poissons fossiles as a member of the genus Sphyraena. However, its attribution to this genus has never been tested by a comprehensive osteological comparative analysis with the extant representatives of the genus Sphyraena; this taxon is badly in need of revision. The Eocene presence of barracudas is also testified to by rare otoliths from the Lutetian Lede Sands (about 47 Ma), Belgium, referred to the species Sphyraena fluctuosa (see Nolf Citation1973). The Oligocene history of these fishes is documented by articulated skeletal remains from Chiavon, northeastern Italy (Sphyraena intermedia; Bassani Citation1889) and Istehbanât, Iran (Sphyraena longimana; Arambourg Citation1967), and otoliths (e.g., Schwarzhans Citation1994). In the Miocene, the fossil record of barracudas is represented by articulated skeletal remains, abundant isolated teeth and otoliths. Articulated skeletal remains are known from the Miocene deposits of the Maros District, in the southern part of Sulawesi, Indonesia (Sphyraena crassidens; De Beaufort Citation1926), the Middle Miocene (Badenian, around the Langhian-Serravallian boundary, about 14.0–13.5 Ma (Carnevale et al. Citation2012; Carnevale & Harzhauser Citation2013) of St. Margarethen, Eisenstadt-Sopron Basin, Austria (Sphyraena sp.; Schultz Citation1998), and the Lower to Middle Miocene deposits of Sagor, Croatia (Sphyraena suessi; e.g., Gorjanović-Kramberger Citation1891). Moreover, Switchenska (Citation1968) described the fossil genus Parasphyraena from the Middle Miocene deposits of Russia. Isolated teeth assigned to the genus Sphyraena have been reported from several localities of Europe, the Atlantic Coastal Plain and Japan (e.g., Nishimoto & Ohe Citation1982; Marsili et al. Citation2007; Carnevale & Godfrey, Citationin press). The possible existence of extant species (e.g., Sphyraena aff. afra) in the Middle Miocene is testified to by otoliths (see e.g., Radwanska Citation1992). Therefore, the fossil record appears to be consistent with the radiation patterns suggested by the timetree, revealing an Oligocene–Miocene worldwide diversification of the genus Sphyraena.
Interestingly, in spite of the younger ages inferred in our study compared to those of Rabosky et al. (Citation2013), most sphyraenid lineages appear to be older than 5 Ma, thus failing to match the pattern observed in many other predominantly reef-associated teleost groups (i.e., Cowman & Bellwood Citation2011; Frédérich et al. Citation2013; Santini et al. Citation2013c), and to large pelagic predators (Santini & Sorenson Citation2013; Santini et al. Citation2013a), all of which experienced significant radiations during the Pliocene and Pleistocene. The differences in the tempo of radiation of these groups were probably linked to a number of environmental factors. The establishment during the past 8–10 Ma of the modern upwelling regime (Jacobs et al. Citation2004) likely greatly influenced the availability of food sources for large pelagics, but may have had less dramatic influences for reef groups such as barracudas, which may have had abundant prey available before large pelagics did. Similarly, greater dispersal abilities in barracudas compared to many other reef fishes (Daly-Engel et al. Citation2012) may have allowed barracudas to be less susceptible to barriers that arose during the Pleistocene sea-level changes, and reduced the opportunities for vicariant speciation in sphyraenids.
The mapping on the chronogram of the habitat association shows several transitions between coral reefs and non-reef habitats, which harbour all the largest species ( and S2), while the biogeographic distribution of the species included in this study reveals that one of the three sphyraenid clades is distributed mostly in the Atlantic and Eastern Pacific, while the other two clades are predominantly Indo-West or Western Pacific, with one species, the great barracuda, attaining an almost circumglobal distribution (Figure S3).
Conclusions
This study produced the most comprehensive phylogeny of barracudas to date, including 20 of the 27 extant species (~75% of extant diversity), as well as a new timescale for sphyraenid diversification. Barracudas are divided into three strongly supported clades, with a distinct biogeographic component: two clades are predominantly found in the Indian and Pacific Oceans, while the third clade is mostly formed by Eastern Pacific and/or Atlantic species. Barracudas likely originated in the Late Paleocene, and their diversification had started by the Middle Eocene (45 Ma), in agreement with the fossil record of the oldest sphyraenids known from the Ypresian (~50 Ma) of Monte Bolca, Italy. Barracudas experienced multiple transitions between reefs and non-reef habitats, and most extant species belong to lineages that date to the Oligo–Miocene, making these fairly old when compared to other predominantly reef-associated fish groups and to large pelagic predators, in which Pliocene diversification has played a fundamental role.
Table S1
Download MS Word (38.5 KB)Figure S3
Download TIFF Image (1,010.3 KB)Figure S2
Download TIFF Image (1.3 MB)Figures S1
Download TIFF Image (750.7 KB)Acknowledgements
This work was made possible by a Lagrange visiting fellowship to FS by Istituto per ľInterscambio Scientifico (ISI). We thank the editor and two anonymous reviewers for comments that helped improve a previous version of this paper. GC was supported by an ex-60% 2012 grant of the Università degli Studi di Torino.
References
- Agassiz L. 1833–1844. Recherches sur les Poissons Fossiles. Nauchâtel: Petitpierre.
- Alfaro ME, Brock CD, Banbury BL, Wainwright PC. 2009. Does evolutionary innovation in pharyngeal jaws lead to rapid lineage diversification in labrid fishes? BMC Evolutionary Biology 9:255. doi:10.1186/1471-2148-9-255.
- Alfaro ME, Santini F, Brock CD. 2007. Do reefs drive diversification in marine teleosts? evidence from the pufferfish and their allies (Order Tetraodontiformes). Evolution 61:2104–2126. doi:10.1111/j.1558-5646.2007.00182.x.
- Arambourg C. 1967. Résultats Scientifiques de la Mission C. Arambourg en Syrie et en Iran (1938–1939). II. Les Poissons Oligocènes de l’Iran. Notes et Mémoires sur le Moyen-Orient 8:1–210.
- Bassani F. 1889. Ricerche sui pesci fossili di Chiavon (Strati di Sotzka – Miocene inferiore). Atti della Reale Accademia di Scienze Matematiche e Fisiche di Napoli 6:1–96.
- Betancur-R R, Broughton RE, Wiley EO, Carpenter K, López JA, Li C, Holcroft NI, Arcila D, Sanciangco M, Cureton IIJC, Zhang F, Buser T, Campbell MA, Ballesteros JA, Roa-Varon A, Willis S, Borden WC, Rowley T, Reneau PC, Hough DJ, Lu G, Grande T, Arratia G, Ortí G. 2013. The tree of life and a new classification of Bony fishes. PLOS Currents Tree of Life. doi:10.1371/currents.tol.53ba26640df0ccaee75bb165c8c26288.
- Carnevale G, Godfrey SJ. in press. Miocene bony fishes of the Calvert, Choptank, St. Marys and Eastover Formations, Chesapeake Group, Maryland and Virginia. In: Godfrey SJ, editor. The geology and vertebrate paleontology of the Calvert cliffs. Bloomington: Indiana University Press.
- Carnevale G, Harzhauser M. 2013. Middle Miocene rockling (Teleostei, Gadidae) from the Paratethys (St. Margarethen in Burgenland, Austria). Bulletin of Geosciences 88:609–620. doi:10.3140/bull.geosci.1432.
- Carnevale G, Harzhauser M, Schultz O. 2012. The Miocene gadid fish Palimphemus anceps Kner, 1862: A reappraisal. Geodiversitas 34:625–643. doi:10.5252/g2012n3a9.
- Cowman PF, Bellwood DR. 2011. Coral reefs as drivers of cladogenesis: Expanding coral reefs, cryptic extinction events, and the development of biodiversity hotspots. Journal of Evolutionary Biology 24:2543–2562. doi:10.1111/j.1420-9101.2011.02391.x.
- Cowman PF, Bellwood DR, van Herwerden L. 2009. Dating the evolutionary origins of wrasse lineages (Labridae) and the rise of trophic novelty on coral reefs. Molecular Phylogenetics and Evolution 52:621–631. doi:10.1016/j.ympev.2009.05.015.
- Daly-Engel TS, Randall JE, Bowen BW. 2012. Is the Great Barracuda (Sphyraena barracuda) a reef fish or a pelagic fish? The phylogeographic perspective. Science of the Total Environment 0:98–105.
- De Beaufort LF. 1926. On a collection of marine fishes from the Miocene of South Celebes. Jaarboek van het Mijnwezen in Nederlandsch Oost-Indië 1925:113–148.
- De Sylva DP. 1984. Sphyraenoidei: development and relationships. In: Moser HG, Richards WJ, Cohen DM, Fahay MP, Kendall Jr AW, Richardson SL, editors. Ontogeny and systematics of fishes. Special Publication No. 1. Lawrence, KS: American Society of Ichthyology and Herpethology. pp. 335–354.
- Doiuchi R, Nakabo T. 2007. Molecular evidence for the taxonomic status of three species of the Sphyraena obtusata group (Perciformes: Sphyraenidae) from East Asia. Ichthyological Research 54:313–316. doi:10.1007/s10228-007-0401-7.
- Dornburg A, Santini F, Alfaro ME. 2008. the influence of model averaging on clade posteriors: An example using the triggerfishes (Family Balistidae). Systematic Biology 57:905–919. doi:10.1080/10635150802562392
- Dornburg A, Sidlauskas B, Sorenson L, Santini F, Near T, Alfaro ME. 2011. The influence of an innovative locomotor strategy on the phenotypic diversification of triggerfish (Family: Balistidae). Evolution 65:1912–1926. doi:10.1111/j.1558-5646.2011.01275.x.
- Drummond AJ, Suchard MA, Xie D., Rambaut A. 2012. Bayesian phylogenetics with BEAUti and the BEAST 1.7. Molecular Biology and Evolution 29:1969–1973. doi:10.1093/molbev/mss075.
- Edgar RC. 2004. MUSCLE: multiple sequence alignment with high accuracy and high throughput. Nucleic Acids Research 32:1792–1797. doi:10.1093/nar/gkh340.
- Frédérich B, Sorenson L, Santini F, Slater GJ, Alfaro ME. 2013. Iterative ecological radiation and convergence during the evolutionary history of damselfishes (Pomacentridae). The American Naturalist 181:94–113. doi:10.1086/668599.
- Froese R, Pauly D. 2014. FishBase. Available: http://www.fishbase.org . Accessed Mar 2014 20.
- Gorjanović-Kramberger D. 1891. Palaeoichthyoložki Prilozi. Dio II. Rad Jugoslavenske Akademije Znanosti i Umjetnosti. u Zagrebu, Mat-Prir. Razr 106:59–129.
- Jacobs DK, Haney TA, Louie KD. 2004. Genes, diversity, and geologic process on the Pacific coast. Annual Review of Earth and Planetary Sciences 32:601–652. doi:10.1146/annurev.earth.32.092203.122436.
- Johnson GD. 1986. Scombroid phylogeny: An alternative hypothesis. Bulletin of Marine Science 39:1–41.
- Kazancioglu E, Near TJ, Hanel R, Wainwright PC. 2009. Influence of sexual selection and feeding functional morphology on diversification rate of parrotfishes (Scaridae). Proceedings of the Royal Society of London, Series B 276:3439–3446.
- Klanten SO, van Herwerden L, Choat JH, Blair D. 2004. Patterns of lineage diversification in the genus Naso (Acanthuridae). Molecular Phylogenetics and Evolution 32:221–235. doi:10.1016/j.ympev.2003.11.008.
- Maddison WP, Maddison DR. 2011. Mesquite: A modular system for evolutionary analysis, version 2.75. Available: http://www.mesquiteproject.org . Accessed Nov 2013 15.
- Marsili S, Carnevale G, Danese E, Bianucci G, Landini W. 2007. Early Miocene vertebrates from Montagna della Maiella, Italy. Annales de Paléontologie 93:27–66. doi:10.1016/j.annpal.2007.01.001.
- Miya M, Friedman M, Satoh TP, Takeshima H, Sado T, Iwasaki W, Yamanoue Y, Nakatani M, Mabuchi K, Inoue JG, Poulsen JY, Fukunaga T, Sato Y, Nishida M. 2013. Evolutionary origin of the scombridae (Tunas and Mackerels): Members of a paleogene adaptive radiation with 14 other pelagic fish families. PLoS ONE 8:e73535. doi:10.1371/journal.pone.0073535.
- Near TJ, Dornburg A, Eytan RI, Keck B, Smith WL, Kuhn KL, Moore JA, Price SA, Burbrink FT, Friedman M, Wainwright PC. 2013. Phylogeny and tempo of diversification in the superradiation of spiny-rayed fishes. Proceedings of the National Academy of Sciences 110:12738–12743. doi:10.1073/pnas.1304661110.
- Nishimoto H, Ohe F. 1982. Teeth of fossil Sphyraena of the Miocene Mizunami Group, central Japan. Bulletin of the Mizunami Fossil Museum 9:85–102.
- Nolf D. 1973. Deuxième note sur les téléostéens des Sables de Lede (Eocène belge). Bulletin de la Société belge de Géologie, de Paléontologie et d’Hydrologie 81:95–109.
- Paradis E, Claude J, Strimmer K. 2004. APE: Analyses of phylogenetics and evolution in R language. Bioinformatics 20:289–290. doi:10.1093/bioinformatics/btg412.
- Posada D. 2008. JModelTest: Phylogenetic model averaging. Molecular Biology and Evolution 25:1253–1256. doi:10.1093/molbev/msn083.
- R Development Core Team. 2014. R: A language and environment for statistical computing. Vienna, Austria. Available: http://www.R-project.org . Accessed Apr 2014 22.
- Rabosky DL, Santini F, Eastman J, Smith SA, Sidlauskas B, Chang J, Alfaro ME. 2013. Rates of speciation and morphological evolution are correlated across the largest vertebrate radiation. Nature Communications 4:1958. doi:10.1038/ncomms2958.
- Radwanska U. 1992. Fish otoliths in the Middle Miocene (Badenian) deposits of southern Poland. Acta Geologica Polonica 42:141–328.
- Reece J.S., Bowen BW, Smith DG, Larson A. 2010. Molecular phylogenetics of moray eels (Muraenidae) demonstrates multiple origins of a shell-crushing jaw (Gymnomuraena, Echidna) and multiple colonizations of the Atlantic Ocean. Molecular Phylogenetics and Evolution 57:829–835. doi:10.1016/j.ympev.2010.07.013.
- Ronquist F, Teslenko M, van der Mark P, Ayres DL, Darling A, Hohna S, Larget B, Liu L, Suchard MA, Huelsenbeck JP. 2012. MrBayes 3.2: Efficient bayesian phylogenetic inference and model choice across a large model space. Systematic Biology 61:539–542. doi:10.1093/sysbio/sys029.
- Sanderson MJ, Boss D, Chen D, Cranston KA, Wehe A. 2008. The PhyLoTA browser: Processing GenBank for molecular phylogenetics research. Systematic Biology 57:335–346. doi:10.1080/10635150802158688.
- Santini F, Carnevale G, Sorenson L. 2013a. First molecular scombrid timetree (Percomorpha: Scombridae) shows recent radiation of tunas following invasion of pelagic habitat. Italian Journal of Zoology 80:210–221. doi:10.1080/11250003.2013.775366.
- Santini F, Kong X, Sorenson L, Carnevale G, Mehta RS, Alfaro ME. 2013b. A multi-locus molecular timescale for the origin and diversification of eels (Order: Anguilliformes). Molecular Phylogenetics and Evolution 69:884–894. doi:10.1016/j.ympev.2013.06.016.
- Santini F, Nguyen M, Sorenson L, Waltzek TB, Alfaro JWL, Eastman JM, Alfaro ME. 2013c. Do habitat shifts drive diversification in teleost fishes? An example from the pufferfishes (Tetraodontidae). Journal of Evolutionary Biology 26:1003–1018. doi:10.1111/jeb.12112.
- Santini F, Sorenson L. 2013. First molecular timetree of billfishes (Istiophoriformes: Acanthomorpha) shows a Late Miocene radiation of marlins and allies. Italian Journal of Zoology 80:481–489. doi:10.1080/11250003.2013.848945.
- Santini F, Sorenson L, Alfaro ME. 2013d. A new multi-locus timescale reveals the evolutionary basis of diversity patterns in triggerfishes and filefishes (Balistidae, Monacanthidae; Tetraodontiformes). Molecular Phylogenetics and Evolution 69:165–176. doi:10.1016/j.ympev.2013.05.015.
- Santini F, Sorenson L, Marcroft T, Dornburg A, Alfaro ME. 2013e. A multilocus molecular phylogeny of boxfishes (Aracanidae, Ostraciidae; Tetraodontiformes). Molecular Phylogenetics and Evolution 66:153–160. doi:10.1016/j.ympev.2012.09.022.
- Schultz O. 1998. Tertiärfossilien Österreichs. Vienna: Goldschneck-Verlag.
- Schwarzhans W. 1994. Due Fisch-Otolithen aus dem Oberoligozän der Niederrheinischen Bucht. Systematik, Palökologie, Palaöbiogeographie, Biostratigraphie und Otolithen-Zonierung. Geologisches Jahrbuch A 140:1–248.
- Sorenson L, Santini F, Alfaro ME. 2014. The effect of habitat on modern shark diversification. Journal of Evolutionary Biology 27:1536–1548. doi:10.1111/jeb.12405.
- Sorenson L, Santini F, Carnevale G, Alfaro ME. 2013. A multi-locus timetree of surgeonfishes (Acanthuridae, Percomorpha), with revised family taxonomy. Molecular Phylogenetics and Evolution 68:150–160. doi:10.1016/j.ympev.2013.03.014.
- Switchenska AA. 1968. A new genus from the family Sphyraenidae from the Middle Miocene of Transcaucasia. In: Ocherki po filogenii i sistematike iskopaemykh ryb I beschelyustnykh. Moscow: Nauka [in Russian]. pp. 157–161.
- Tamura K, Peterson D, Peterson N, Stecher G, Nei M, Kumar S. 2011. MEGA5: Molecular evolutionary genetics analysis using maximum likelihood, evolutionary distance, and maximum parsimony methods. Molecular Biology and Evolution 28:2731–2739. doi:10.1093/molbev/msr121.
- Yang Z. 2006. Computational molecular evolution. Oxford: Oxford University Press.