Abstract
As in animals and other multicellular organisms, protozoan ciliates communicate via diffusible signalling pheromones. These pheromones have been identified in the culture supernatant of various species. However, their isolation and definitive structural characterization have been achieved only in species of Blepharisma and Euplotes. With the exception of the two B. japonicum pheromones represented by a tryptophan-related molecule and a glycoprotein, all the other pheromones isolated from Euplotes species are proteins that vary in extension between 38 and 109 amino acids. They form species-specific families of structurally homologous members, in full accord with their multi-allelic determination at an apparently single genetic locus. The determination of the Nuclear Magnetic Resonance (NMR) solution structures of E. raikovi and E. nobilii pheromones has provided direct evidence that, although structurally unique, Euplotes pheromones adopt a common, species-specific three-helix folding. This close structural homology among members of the same family well accounts for the pheromone capacity to compete with one another in cell receptor binding reactions and elicit context-dependent, autocrine (growth-promoting) or paracrine (mating-inducing) cell responses.
Introduction
Half a century ago, Karlson and Lüscher (Citation1959) proposed the new word “pheromone” to refer to the chemicals used to communicate among members of the same species. Since then, pheromones have been described pervasively in all three kingdoms of multicellular life. However, single-celled microorganisms also synthesize substances which fully satisfy the definition of pheromones, which is “substances which are secreted to the outside by an individual and received by a second individual of the same species in which they release a specific reaction such as a definite behavior or a developmental process” (Karlson & Lüscher Citation1959, p. 56).
Diffusible (water-borne) pheromones in protozoan ciliates were first identified more than 70 years ago (Kimball Citation1942) in Euplotes patella (Müller, 1773) Ehrenberg, 1838, which is one of the dozens of Euplotes Ehrenberg [1830] species that have colonized every aquatic habitat of our planet. To investigate the genetic mechanism of mating types that controls conjugation (or mating), Kimball (Citation1942) obtained effective intra-clonal (or selfing, or homotypic) mating pairs (i.e. pairs between cells taken from the same culture which originated from a single isolate, hence between cells which were genetically identical) by suspending cell cultures of a given mating type with cell-free filtrates of cultures expressing different, compatible mating types. Clearly, this finding provided evidence (albeit indirect) that E. patella cells secrete substances capable of inducing mating pair formation between cells other than the ones from which they are secreted. Because of this activity, these cell type-specific substances were for a long time exclusively considered to be “sex factors” and, consistently with this view, denominated “gamones” to emphasize a supposedly unique role in ciliate mating interactions (Miyake Citation1974). The term “gamone” (which is a contracted form of “gametenhormon”) was originally coined by Hartmann and Schartau (Citation1939) and used by Weise (Citation1961) to indicate the mating type-specific substances that are produced by male and female gametes of heterothallic species of the green alga, Chlamydomonas Ehrenberg, 1833, for interacting and uniting into zygotes.
However, the term gamone used for the ciliate mating-type substances was regarded as misleading (Luporini & Miceli Citation1986), first because it assumes that ciliates interacting for mating are functionally equivalent to physiologically complementary female and male gametes, whereas they are vegetative cells (gamonts) that resemble hermaphroditic organisms which will produce two gametic nuclei (a migratory/male one plus a stationary/female second one) only as a consequence of their mating interactions and temporary fusion in mating pairs, and, second, because ciliate species endowed with a binary mating system (like sexes) are an exception rather than the rule. Multiple systems which comprise indefinite numbers of different and mutually compatible mating types appear to be much more frequent (Dini & Nyberg Citation1993; Phadke & Zufall Citation2009).
In light of these considerations, the ciliate mating-type substances were thus regarded as “pheromones” to indicate a larger spectrum of activities, not limited to the induction of mating (sex) (Luporini & Miceli Citation1986). As a matter of fact, ciliate pheromones are now known to function not only as non-self (paracrine-like, or heterologous) mating induction signals, but also as self (autocrine, or autologous) signals which primarily bind to the same cells from which they are secreted and promote their vegetative (mitotic) growth (Vallesi et al. Citation1995).
In determining the ciliate pheromone structures, direct chemical analysis of the purified material has often been hampered by the tiny, micro-molar concentrations in which pheromones are usually recovered from the cell-free filtrates. Therefore, this analysis has been largely integrated and/or replaced with genetic approaches that seek to deduce the pheromone amino acid sequences from the knowledge of the structure of the pheromone genes. These genes differentiate from the transcriptionally silent mating-type (mat) genes of the germinal chromosomic genome of the cell micronucleus and are expressed, after amplification to thousands of copies, in the somatic genome of the macronucleus, which is characterized by a very eccentric sub-chromosomic organization (Jahn & Klobutcher Citation2002).
Pheromone-secreting species
Pheromone-secreting species have been identified on the basis of successful mating induction assays, in which selfing mating pairs have been induced by suspending clonal cell samples with filtrates of cultures of other, genetically different cells. However, their identification has also been inferred from other assays based on chemo-attraction between cells of different mating types (Sonneborn Citation1978), or attempts to shorten the interval of time (known as “waiting period”) that cells of different mating types usually require to form stable mating pairs after having been mixed together (Esposito et al. Citation1976).
Although the overall number of known pheromone-secreting species is quite meager, the distribution of these species in distant clades of the ciliate phylogenetic tree implies that pheromone secretion is a phenomenon of general occurrence in ciliates. In Heterotrichea Stein, 1859, it has been reported in Blepharisma japonicum (Suzuki, 1954) Giese, 1973, B. americanum Suzuki, 1954, B. musculus Penard, 1922, B. stoltei Isquith, 1966 and B. tropicum Bhandary, 1962 (Miyake & Bleyman Citation1976); in Spirotrichea Butschli, 1889, in Euplotes patella (Kimball Citation1942; Sato Citation1983; Akada Citation1985, Citation1986), E. woodruffi Gaw, 1939 (Kosaka Citation1990), E. raikovi Agamaliev, Citation1966 (Luporini et al. Citation1983; Miceli et al. Citation1983), E. octocarinatus Carter, 1972 (Weischer et al. Citation1985), E. nobilii Valbonesi and Luporini, 1990 (Felici et al. Citation1999; Alimenti et al. Citation2002, Citation2003), E. crassus (Dujardin) Kahl, 1932 (Alimenti et al. Citation2011) and Oxytricha bifaria Stokes, 1887 (Esposito et al. Citation1976); in Litostomatea Small and Lynn, 1981, in Dileptus anser (O.F. Müller, 1786) Dujardin, 1841 (Tavrovskaja Citation1979; Yudin et al. Citation1990); in Phyllopharyngea de Puytorac et al., 1974, in Tokophrya infusionum (Stein, 1859) Bütschli, 1889 and T. lemnarum (Stein, 1859) Entz, 1903 (Sonneborn Citation1978). Among these pheromone-secreting species, pheromones have effectively been isolated and their structures determined to varying degrees of complexity only in five species, namely B. japonicum, E. raikovi, E. octocarinatus, E. nobilii and E. crassus. These structures are described below, starting from those of B. japonicum in which pheromones have for the first time been isolated and purified to homogeneity.
Blepharisma mating types and pheromones
In a manner typical of heterotrichous ciliates, such as Stentor Oken, 1815, Folliculina Lamark, 1816, Climacostomum Stein, 1859 and others, clonal cultures of every species of Blepharisma Perty, 1849 form fertile mating pairs in an unpredictable and spontaneous manner, thus implying that this ciliate conjugates without the genetic control of a mating-type mechanism. Sex occurs in Blepharisma species in the systematic form of intra-clonal conjugation, or selfing. Nevertheless, by serially isolating unmated cells from selfing cultures of B. japonicum, it was eventually possible to obtain two distinct, temporarily non-selfing cell lines (one phenotypically characterized by a pink pigmentation, and the other one, albino, by no pigmentation) which formed mating pairs only after having been mixed together (Isquith & Hirshfield Citation1968). Though these lines were clearly the result of a manual selection (not of a genetic selection), they were taken as proxies of two intraspecific complementary mating types, I and II (Miyake & Beyer Citation1973), and as such used in all the structural and functional studies on B. japonicum pheromones, disregarding the cautionary remark by Isquith and Hirshfield (Citation1968, p. 516) that they “arose in the laboratory and probably would have not survived in nature”.
Two B. japonicum pheromones have been structurally characterized as two chemically unrelated molecules: one molecule designated as Gamone 1, or Blepharmone, from the supernatant of type-I cell cultures, and the second one designated Gamone 2, or Blepharismone, from the supernatant of type-II cell cultures (Kubota et al. Citation1973; Miyake & Beyer Citation1974). Gamone 1 was identified with a species-specific glycoprotein active in pico-gram concentrations in inducing mating between type-II cells. Determination of its macronuclear coding sequence (Sugiura & Harumoto Citation2001) showed that it is synthesized as a cytoplasmic precursor (pre-pro-gamone-1) destined to be proteolytically cleaved for the pre- and pro-segment removal and release of a mature protein of 272 amino acids plus six N-linked sugar molecules. In the secreted protein, polar and aromatic amino acids are present in much larger amounts (Asn 10.3%, Ser 10.3%, Tyr 8.1%, Trp 5.1%) than charged ones (Glu 1.5%, Arg 2.2%, Lys 3.7%). Purified preparations of Gamone 1 were reported to be very unstable at ambient temperatures – quite a striking trait for an environmental signal – and needed to be stored at freezing temperatures and protected by the addition of serum albumin (Miyake & Beyer Citation1974). In contrast, Gamone 2 has been identified with a very stable tryptophan derivative, namely a calcium-3-(2’-formyl-amino-5’-hydroxybenzoil) lactate (), which appears to be synthesized also by other species of Blepharisma in addition to B. japonicum (Miyake & Bleyman Citation1976). This molecule has also been obtained through chemical synthesis (Entzeroth & Jaenicke Citation1981), and its laboratory copies are active like the original ones at nanomolar concentrations in inducing chemo-attraction and mating of type-I cells (Sugiura et al. Citation2010).
Figure 1. Chemical structure of the Blepharisma japonicum tryptophan-related pheromone designated as Gamone-2, or Blepharismone. After Kubota et al. (Citation1973).
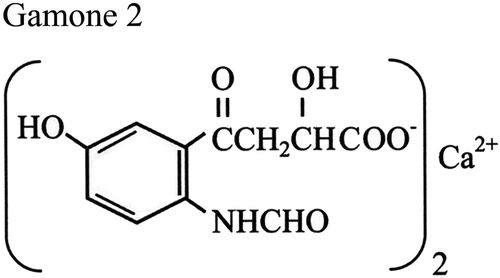
Despite the substantial differences that distinguish Gamones 1 and 2 in structure, behavior, activity and evolution – the non-species-specific Gamone 2 has been inferred to precede the evolution of the species-specific Gamone 1 (Miyake & Bleyman Citation1976) – the two pheromones have been assumed to engage in a very symmetric interplay. In a model known as the “gamone-receptor hypothesis” (Miyake Citation1981), Gamones 1 and 2 would find their specific receptors on type II and type I cells, respectively, and these two symmetric gamone receptor-binding reactions would determine the activation of a positive feedback loop responsible for the physiological transformation of B. japonicum vegetative cells into sexually complementary mating partners. This model has been criticized, first, because it does not satisfy the striking structural asymmetry between Gamones 1 and 2 (Luporini & Miceli Citation1986), and, second, because it does not account for (i) the wild-type selfing behavior of B. japonicum, (ii) the instability of the supposed mating types I and II and (iii) the inability of in vitro-induced intra-clonal (selfing) mating pairs (i. e., I–I, and II–II), unique among all ciliates, to switch on the meiotic maturation of the micronucleus. An alternative model overcomes these problems. Since the constitutive selfing behavior of B. japonicum implies that this species did not evolve a genetic mating-type mechanism capable of effectively controlling the ensuing of mating, it was hypothesized that the B. japonicum wild-type cells all regularly co-secrete both Gamones 1 and 2, which interact with one another, forming glycoprotein/tryptophan-derivative complexes that are the effective cell mating-inducing signals (Luporini & Miceli Citation1986).
Euplotes mating types
In contrast with the lack of information on the genetic determination of Blepharisma mating types and their associated gamones, the genetic base of Euplotes mating types has been known since the pioneering work carried out by Kimball (Citation1942) in E. patella. Analyses of formal (Mendelian) genetics (uniquely based on the inspection of mating mixtures for detecting the presence or lack of mating pair formation) have shown that Euplotes species inherit their mating types via multiple series of alleles which segregate at an apparently single mat (or mt) locus of the micronuclear germinal genome, and are expressed, in the form of gene-size DNA molecules amplified to thousands copies, in the transcriptionally active somatic genome of the macronucleus (Dini & Nyberg Citation1993).
Euplotes raikovi pheromones
Among the four Euplotes species that have so far been analyzed for their pheromone structures, E. raikovi deserves a particular mention as the first ciliate in which these structures have been resolved up to the level of three-dimensional architecture. Originally described from the Caspian Sea by Agamaliev (Citation1966) for its unique pattern of eight fronto-ventral cirri, this sand-dwelling psammophilic species was later found to have a wide distribution in temperate seas. Its use in the pheromone study started with a group of strains collected from the seashore of Porto Recanati on the Adriatic coast of Italy (Miceli et al. Citation1981). The general constitutive capacity of these strains to secrete biologically active pheromones was promptly revealed by the application of the usual mating induction assays (Luporini et al. Citation1983). Using a very effective purification procedure involving only three chromatographic steps (Raffioni et al. Citation1988), it was soon realized that these pheromones (also designated “Euplomones”, by merging “Euplotes” and “pheromones” into a single term) were secreted throughout the cell life cycle regardless of the developmental or physiological cell competence to mate, as well as in amounts that made it feasible to pursue direct chemical analyses of their structures. It was usually possible to obtain 100_200 μg of pure protein from 1 L of cell-free filtrate (Raffioni et al. Citation1992), and this quantitative pheromone production appears to have no match in other ciliate species.
Nine distinct amino acid sequences, designated Er-1, Er-2, Er-7, Er-10, Er-11, Er-20, Er-21, Er-22 and Er-23 (where E and r stand for Euplomone and raikovi, respectively, and the numbers classify progressive pheromone characterizations), have been determined for the E. raikovi pheromone family (Raffioni et al. Citation1992; Giuseppe et al. Citation2002; Luporini et al. Citation2005). As is the case for the B. japonicum Gamone 1 and exocytotic proteins in general, these E. raikovi pheromones also appear to be synthesized as pre-pro-pheromones and secreted after two proteolytic cleavages that remove the pre (signal-peptide) and pro segments (Miceli et al. Citation1991). Of the 75 amino acid residues (86 in the deviant family member, Er-23) that form these precursors, 19 belong to the signal peptide, 16_18 to the pro segment, and 37_40 to the secreted form (51 in Er-23). For all molecules, the signal peptide sequence is fully conserved. It contains all the structural elements necessary for the pre-pro-pheromone translocation across membranes. Only a slightly wider sequence variability characterizes the pro segment.
Instead, maximal variability in the amino acid sequences characterizes the mature, secreted pheromones (A). Only the positions of six cysteines are fully conserved in the nine E. raikovi pheromone sequences. Sequence identity between any two pheromones may be as low as 26%, as is the case, for instance, of pheromones Er-1 and Er-2, which are co-secreted by the same cells heterozygous for co-dominant mat genes (Luporini et al. Citation1986). Notwithstanding this wide amino acid sequence variability, the determination of the three-dimensional architectures of pheromones Er-1, Er-2, Er-10, Er-11, Er-22 and Er-23 by NMR spectroscopy and (limited to Er-1) X-ray crystallography has shown that these molecules all share a common structural folding (B). This is provided by a complex of three right-handed α helices (five in Er-23, of which the additional two are very short and single-turn helices), which take a a nearly parallel and up-down-up orientation (Brown et al. Citation1993; Luginbühl et al. Citation1994; Mronga et al. Citation1994; Ottiger et al. Citation1994; Weiss et al. Citation1995; Liu et al. Citation2001; Zahn et al. Citation2001). The three helices are linked together by closely spaced and tightly conserved disulfide bonds, two of which involve potential alternative cysteine pairings that have been found to be consistent with major NMR constraints and the formation of native disulfide isomers (Brown et al. Citation1993). The structural stability of E. nobilii pheromones, which is an essential feature of signalling molecules that must remain active for a long time in the extracellular milieu, is such that there is no unfolding and disruption of the secondary structures upon heating up to 95°C (Geralt et al. Citation2013).
Figure 2. Amino acid sequences and three-dimensional NMR solution structures of Euplotes raikovi pheromones. A, sequence alignment based on the Clustal W algorithm and optimized by deliberate gap insertions. Cysteine residues (six in all sequences with the exception of the eccentric Er-23 sequence including 10 cysteines) are shadowed, indicated by progressive Roman numerals and connected by lines according to their pairings in disulfide bridges. The residue numbers of each sequence are reported on the right. Fully conserved residues are marked by filled dots, and a light dot indicates a position characterized by a variation of a single amino acid. In pheromones with known secondary structures, the sequence segments with helical organization are enclosed in boxes. B, NMR structures. The α-helices (h) are numbered progressively from the molecule amino-terminus (N) to the carboxy-terminus (C), and represented as cylinders along with a 310 turn helical motif.
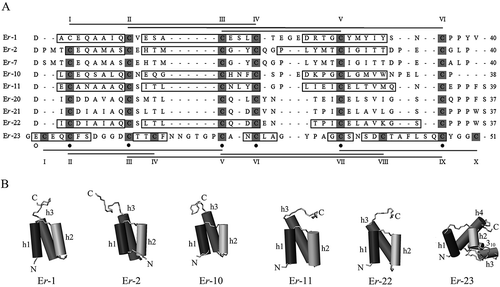
The most apparent structural personalization of each pheromone lies in the carboxy-terminal domain of the molecules (Raffioni et al. Citation1992; Luginbühl et al. Citation1994). This domain carries extensive variations in both length and geometry of the sequence segment subsequent to the last cysteine, as well as in the topology of the disulfide bond that connects this cysteine to the second one conservatively positioned at the top of the first helix. Other remarkable structural earmarks are provided by variations in the organization of the second helix and the geometry of the loop that connects this helix to the third one.
Consistent with their multi-allelic determination at a single genetic locus, E. raikovi pheromones thus form a homologous protein family whose members are at the same time clearly distinct from one another because of unique structural traits, and characterized by largely overlapping folding patterns. Each member can thus compete, to different degrees of affinity, with any other family member for binding to the cell pheromone receptors (Ortenzi & Luporini Citation1995). These receptors have tentatively been identified with membrane-bound pheromone isoforms generated, through a splicing mechanism of intron-like sequences, from the same macronuclear genes that specify the soluble pheromones (Miceli et al. Citation1992; Ortenzi et al. Citation2000). Because of their origin from the same gene, each cell type would be characterized by a structural equivalence between its soluble pheromone and the extracellular carboxy-terminal domain of the pheromone receptor. This context has suggested that the pheromone/receptor interactions on the cell surface can be fully mimicked by the interactions that take place among pheromone molecules which are packed in an orderly fashion into a crystal lattice, and involve all three helices (and the three faces, a, b and c, that they delimit) of each molecule, figured as a pyramid with a triangular base (Weiss et al. Citation1995). Strong pheromone/receptor binding appears to be derived from the cooperative utilization of several weak interactions generated from the formation of two distinct types of dimer (). The first one, symmetrical, involves the first and second helices which delimit the face a of each monomer; the second one, asymmetrical, involves the third helix which delimits, together with the second helix, face b and, together with the first helix, face c. This model, that has been supported by immunological analyses (Vallesi et al. Citation1995, Citation2005), has the virtue of providing a univocal molecular explanation for the pheromone/receptor interactions of autocrine (self) type that are associated to the cell growth activity, and of paracrine (non-self) type that are responsible for inducing cells to temporarily unite in mating pairs (which in ciliates, it is worth pointing out, may equally involve cells which are genetically different, or cells which are genetically identical).
Figure 3. Pheromone/receptor interactions on the cell surface. The model is based on a crystallographic analysis of the Euplotes raikovi pheromone Er-1 and predicts that strong pheromone/receptor binding emerges as a consequence of the cooperative utilization of weak interactions involving all the three Er-1 helices in the formation of two distinct types of dimer (Weiss et al. Citation1995). A, autocrine (autologous) interactions between diffusible and membrane-bound pheromone (receptor) molecules produced by the same cell type. The protein–protein complexes are internalized and their internalization activates a cell growth signaling pathway (Vallesi et al. Citation2005, Citation2010a). B and C, paracrine (heterologous) interactions between diffusible and membrane-bound pheromone (receptor) molecules produced by cells of different types (e.g. I and II); the protein–protein complexes are not internalized and cells use their membrane-bound pheromone (receptor) molecules to stabilize their unions in mating pairs. Diagram B illustrates the case in which cells of a given type form homotypic (selfing, or intraclonal) mating pairs after having been suspended with a pheromone produced by another cell type. Diagram C illustrates the case in which cells of two different types form heterotypic mating pairs after having been mixed together with their secreted pheromones.
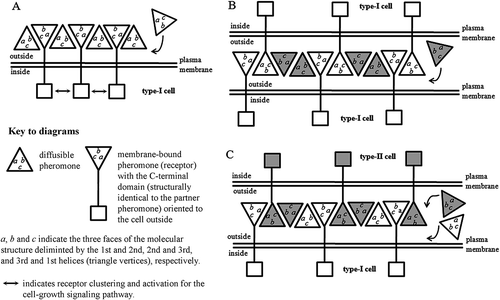
Euplotes nobilii pheromones
With regard to genetic determination and structure, the E. raikovi pheromone family finds a fully matching counterpart with the E. nobilii pheromone family. This is consistent with the very close phylogenetic kinship that links these two Euplotes species which, however, differ significantly in ecology (Jiang et al. Citation2010; Yi et al. Citation2012). Whereas E. raikovi lives in temperate sea waters, E. nobilii is a cold-loving (psychrophilic) species distributed in the freezing Antarctic and Arctic coastal waters (Di Giuseppe et al. Citation2011, Citation2013). Given this context, E. nobilii pheromones were investigated to identify the structural variations that distinguish pheromone families of two distinct species, as well as the modifications in structure and function that psychrophilic signalling proteins in general have adaptively evolved to preserve their biological activity at sub-zero temperatures.
Seven E. nobilii pheromones (collectively designated as “En”) are known for their primary amino acid sequences (A): three (En-1, En-2, and En-6) are specific to Antarctic strains and four (En-A1, En-A2, En-A3 and En-A4) are specific to Arctic ones (Vallesi et al. Citation2009, Citation2012). The NMR solution structures are known only for four of them, which could be purified in larger amounts, namely En-1, En-2, En-6 and En-A1 (Pedrini et al. Citation2007; Placzek et al. Citation2007; Di Giuseppe et al. Citation2011) (B).
Figure 4. Amino acid sequences and three-dimensional NMR solution structures of Euplotes nobilii pheromones. A, sequence alignment based on the Clustal W algorithm and optimized by deliberate gap insertions. Cysteine residues are shadowed, indicated by progressive Roman numerals and connected by lines according to their pairings in disulfide bridges. The residue numbers of each sequence are reported on the right. Fully conserved residues are marked by filled dots, and light dots indicate the positions characterized by variations of a single amino acid. In pheromones with known secondary structures, the sequence segments with helical organization are enclosed in boxes. B, NMR structures. The α-helices (h) are numbered progressively from the molecule amino-terminus (N) to the carboxy-terminus (C), and represented as cylinders along with a 310 turn helical motif.
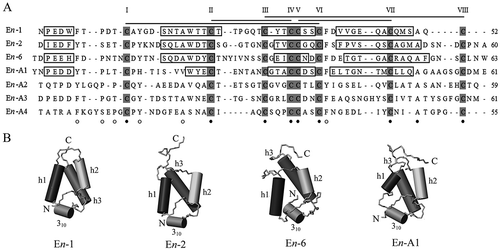
As in E. raikovi pheromones, E. nobilii pheromones also have structures based on an up-down-up, three-helix bundle stabilized by closely spaced disulfide bonds. However, in apparent relationship with their adaptation to cold, they present marked variations in amino acid composition as well as in the conformation of particular molecular domains (Alimenti et al. Citation2009). They are richer in polar and aromatic amino acids, poorer in hydrophobic ones, and have higher indexes of global hydrophilicity. At the conformational level, the lengths of their three core α-helices are markedly reduced, with a consequent major extension of the regions that are devoid of regular secondary structures. These extensions involve two regions in particular. The first one is the amino-terminal region which is formed by a long segment of 14_17 amino acids and includes only an irregular single-turn helix. The second one is the loop that bridges the first two helices and extends for 5–10 amino acids. This architectural predominance of random coiled domains over regularly structured domains appears to be functionally associated with an enhanced flexibility of the molecular backbone. In effect, molecules with a more flexible backbone may better counterbalance the negative thermodynamic effects of polar waters. In full accord with their higher structural plasticity, the E. nobilii pheromones have been found to be much less thermodynamically stable than E. raikovi pheromones. They unfold upon heating at only 55_70°C (Cazzolli et al. Citation2013; Geralt et al. Citation2013).
Euplotes octocarinatus and E. crassus pheromones
In comparison to E. raikovi and E. nobilii pheromones, much less information is available on the structures of E. octocarinatus and E. crassus pheromones, for which only the primary amino acid sequences are known from their respective macronuclear DNA coding sequences. These pheromones are synthesized in much lower concentrations, rendering studies of their three-dimensional conformations impractical. In E. octocarinatus, a maximum of 0.5 μg of pure protein has usually been recovered from 1 L of culture supernatant (Dieckhoff et al. Citation1987), while 15_20 μg/L are usually obtained from supernatant preparations of E. crassus (Alimenti et al. Citation2011).
Nine pheromone amino acid sequences have been determined in E. octocarinatus, which is a lacustrine species not closely allied to E. raikovi, E. nobilii and E. crassus, and is characterized by a distribution apparently limited to North America (Möllenbeck & Heckmann Citation1999). As shown in , they vary between 85 and 109 amino acids with 10 cysteines (eight in the family member annotated as “Phr4”), so that they are practically twice as long as the E. raikovi and E. nobilii pheromone sequences (Brünen-Nieweier et al. Citation1998; Möllenbeck & Heckmann Citation1999). Only four of the 10 cysteines have conserved counterparts in E. raikovi and E. nobilii pheromones, implying that the E. octocarinatus pheromones share only two disulfide bonds and few structural motifs in common with those of E. raikovi and E. nobilii. In addition, they appear to be unique in Euplotes for functioning as chemo-attractants between cells of different mating types (Kuhlmann et al. Citation1997).
Figure 5. Amino acid sequences of Euplotes octocarinatus pheromones. The pheromone designations are as reported in Brünen-Nieweier et al. (Citation1998) and Möllenbeck and Heckmann (Citation1999), where the presence or absence of an asterisk indicates pheromones isolated from strains of presumably different “syngens” (i.e. genetic species). Sequence alignment is based on the Clustal W algorithm and optimized by deliberate gap insertions. Cysteine residues are shadowed and residue numbers of each sequence are reported on the right. Fully conserved residues are marked by filled dots, and light dots indicate the positions characterized by variations of a single amino acid.
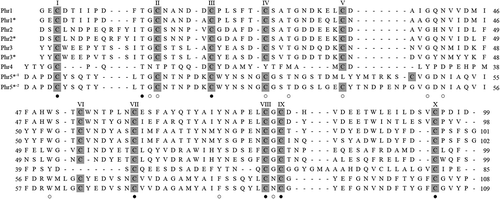
The pheromones that have been structurally characterized most recently are those of E. crassus (Alimenti et al. Citation2011; Vallesi et al. Citation2014). They have traditionally been thought of as insoluble membrane-bound proteins and, as such, difficult to extract, purify and characterize. This conclusion was drawn from observing that no homotypic (intraclonal) mating pair was formed by E. crassus cultures suspended with heterologous cell-free filtrates. These unsuccessful in vitro mating inductions were taken to indicate a lack of pheromones in solution and to predict that E. crassus pheromones must be membrane-bound proteins (Miyake Citation1981). To obtain evidence that E. crassus pheromones are, instead, constitutively secreted as in other Euplotes species, it was necessary to undertake interspecific mating induction assays on E. raikovi cells (Alimenti et al. Citation2011), based on the notion that cross-mating reactions are relatively frequent between Euplotes species in correlation with their high-multiple (virtually open) mating systems (Nobili et al. Citation1978; Valbonesi et al. Citation1992; Kuhlmann & Sato Citation1993).
Unlike E. raikovi, E. nobilii and E. octocarinatus, in which (as noted above) there is convergent evidence for a pheromone control based on multiple alleles at a single locus, in E. crassus, results from molecular approaches appeared to conflict with the traditional paradigm warranting an analogous control. These results were found to be more consistent with a duplication of the E. crassus mat locus, and the consequent production of two distinct pheromone sub-families (Alimenti et al. Citation2011; Vallesi et al. Citation2014). One sub-family has been proposed to include the cell type-specific pheromones controlled by alleles at the mat locus which is orthologous to the mat locus of other Euplotes species, while the second sub-family would include a new species of pheromones controlled by alleles at the paralogous mat locus. It is not known which functions characterize these new pheromones with respect to the cell type-specific ones. Preliminary evidence suggests that they might behave like adaptor or scaffold proteins that selectively interact with the cell type-specific pheromones to mediate and reinforce their receptor binding reactions.
So far, only three complete sequences (designated Ec-1, Ec-2 and Ec-3) of 45 amino acids with 10 cysteines have been determined for the E. crassus cell type-specific pheromones controlled at the orthologous mat locus, and a single sequence (designated Ec-α) of 56 amino acids with eight cysteines has been determined for the pheromones controlled at the paralogous mat locus. As shown in , the sequence comparison of these pheromones makes it evident that the major structural differences between the Ec-α pheromone and the other pheromones are due to the evolution of an unusually extended hydrophilic domain, that lies centrally in the molecule, contains no cysteine and is rich in glycine residues. This domain likely takes a random-coil conformation, which suggests that it might favor an increased structural plasticity of the Ec-α pheromone and hence the capability of this pheromone to interact with the other cell type-specific ones.
Figure 6. Amino acid sequences of Euplotes crassus pheromones. A, sequence alignments of pheromones which are presumed to be specified by alleles at a mat locus orthologous to that one of other Euplotes species. Cysteine residues are shadowed and residue numbers of each sequence are reported on the right. Fully conserved residues are marked by filled dots, and light dots indicate the positions characterized by variations of a single amino acid. B, sequence of the Ec-α pheromone, the only pheromone with a known primary structure among those which are presumed to be specified by alleles at a duplicated (paralogous) mat locus.

Concluding remarks
The knowledge of the structures of ciliate pheromones is currently limited to a few species of Blepharisma and Euplotes. This appears to be quite odd, considering that over 70 years have passed since these chemical signals were identified in ciliate culture filtrates (Kimball Citation1942). Even more odd is the length of time that was needed to characterize the structure and mechanism of expression of the mating type genes responsible for the pheromone specificities. These genes were identified in 1937 by Sonneborn in Paramecium Müller, 1773, and soon after in Euplotes (Kimball Citation1942), Tetrahymena Furgason, 1940 (Elliott & Nanney Citation1952), Stylonychia Ehrengerg, 1830 (Downs Citation1956) and other ciliates. However, their structure and mechanisms of expression have only recently been determined, and only in Tetrahymena (Cervantes et al. Citation2013) and Paramecium (Singh et al. Citation2014), for which full genome mapping is available (Aury et al. Citation2006; Eisen et al. Citation2006). In Euplotes, knowledge remains limited to the expressed macronuclear versions of the mating type genes (Vallesi et al. Citation2009, Citation2010b, Citation2012), and nothing is yet known about the unexpressed micronuclear versions of these genes.
While this length of time necessary to shed definitive light on the genetic determinants of ciliate mating types is reasonably explained by the previous lack of very effective experimental tools in molecular genetics, the same does not hold for the limited knowledge of the ciliate pheromone structures. Considering that very effective analytical procedures in protein chemistry have been available for a long time, the causes of this limited knowledge must be sought elsewhere. An objective cause certainly resides in the fact that ciliates usually secrete only tiny amounts of their pheromones, and thus painstaking efforts are required to accumulate liters and liters of cell-free filtrates as starting material for the pheromone preparations. A second and more subjective cause is that the identification of pheromone-secreting species has so far relied on the outcome of mating induction assays. Both success and failure in inducing mating pair formation within clonal cell cultures suspended with heterologous cell-free filtrates have been taken as evidence to discriminate between pheromone-secreting and pheromone non-secreting species. However, a lack of pheromones in solution cannot be taken as the possible reason for a failed mating induction. Another obvious reason may be that cells have an intrinsic resistance to forming intra-clonal (homotypic) mate pairs in response to in vitro heterologous pheromone interactions. Therefore, for the identification of new pheromone-secreting species, it would be expedient to overcome the limits of the mating induction assays and implement bioassays in which cell-free filtrates are analyzed to identify pheromones in solution through other pheromone activities such as the induction of chemo-attraction and cell multiplication, as was successfully done in D. anser (Afon’kin & Yudin Citation1987; Yudin et al. Citation1990).
Also, it might be helpful to assess ciliate pheromone cross-reactions and cross-talking with other cell signalling systems. This perspective is prompted by the observation that the three-helix structural folding of Euplotes pheromones shares relevant similarities with a variety of helical and disulfide-rich small proteins, such as mollusk attractins, sea anemone peptide toxins, anaphylotoxins and others (Cheek et al. Citation2006), which all drive cell communication. Quite striking is the capability of Euplotes pheromones (Er-1 in particular) to effectively interact with mammalian lymphocyte cell lines and condition their mitotic growth by binding to receptor subunits of the cytokine interleukine-2 (Vallesi et al. Citation1998; Cervia et al. Citation2013). We can presently only speculate on whether these cross-reactions reflect genuine molecular relationships via divergent evolution, or represent chance structural convergence. Independently of which alternative is valid, their occurrence reinforces the concept that the evolution of cell signalling mechanisms via diffusible pheromones precedes the origin of multicellular life, and stimulates new research in structural biology of ciliate pheromones from a biotechnological and applied perspective.
Acknowledgements
Grants from the Ministero dell’Istruzione, dell’Università e della Ricerca (MIUR) and Programma Nazionale di Ricerche in Antartide (PNRA) provided financial support for the research carried out in the authors’ laboratory. Advice from Mrs. Sheila Beatty in editing the English usage was greatly appreciated.
References
- Afon’kin SY, Yudin AL. 1987. Distribution of Dileptus anser (Ciliata, Holotricha, Gymnostomatida) of various genotypes for mat locus in the concentration gradient of their gamones. Acta Protozoologica 26:91–100.
- Agamaliev FG. 1966. New species of the psammobiotic ciliates of the Western cost of the Caspian Sea. Acta Protozoologica 4:169–183.
- Akada R. 1985. Mating types and mating-inducing factors (gamones) in the ciliate Euplotes patella syngen 2. Genetical Research 46:125–132. doi:10.1017/S0016672300022618.
- Akada R. 1986. Partial characterization of the mating‐inducing factors (gamones) produced by mating type VI cells in the ciliate Euplotes patella syngen 2. Journal of Experimental Zoology 237:287–290. doi:10.1002/jez.1402370215.
- Alimenti C, Ortenzi C, Carratore V, Luporini P. 2002. Structural characterization of a protein pheromone from a cold-adapted (Antarctic) single-cell eukaryote, the ciliate Euplotes nobilii. FEBS Letters 514:329–332. doi:10.1016/S0014-5793(02)02393-1.
- Alimenti C, Ortenzi C, Carratore V, Luporini P. 2003. Structural characterization of En-1, a cold-adapted protein pheromone isolated from the Antarctic ciliate Euplotes nobilii. Biochimica et Biophysica Acta (BBA) - General Subjects 1621:17–21. doi:10.1016/S0304-4165(03)00011-4.
- Alimenti C, Vallesi A, Federici S, Di Giuseppe G, Fernando D, Carratore V, Luporini P. 2011. Isolation and structural characterization of two water-borne pheromones from Euplotes crassus, a ciliate commonly known to carry membrane-bound pheromones. Journal of Eukaryotic Microbiology 58:234–241. doi:10.1111/j.1550-7408.2011.00535.x.
- Alimenti C, Vallesi A, Pedrini B, Wüthrich K, Luporini P. 2009. Molecular cold-adaptation: Comparative analysis of two homologous families of psychrophilic and mesophilic signal proteins of the protozoan ciliate, Euplotes. IUBMB Life 61:838–845. doi:10.1002/iub.228.
- Aury J-M, Jaillon O, Duret L, Noel B, Jubin C et al. 2006. Global trends of whole-genome duplications revealed by the ciliate Paramecium tetraurelia. Nature 444:171–178.
- Brown LR, Mronga S, Bradshaw RA, Ortenzi C, Luporini P, Wüthrich K. 1993. Nuclear magnetic resonance solution structure of the pheromone Er-10 from the ciliated protozoan Euplotes raikovi. Journal of Molecular Biology 231:800–816. doi:10.1006/jmbi.1993.1327.
- Brünen-Nieweier C, Christoph Weiligmann J, Hansen B, Kuhlmann H-W, Möllenbeck M, Heckmann K. 1998. The pheromones and pheromone genes of new stocks of the Euplotes octocarinatus species complex. European Journal of Protistology 34:124–132. doi:10.1016/S0932-4739(98)80022-9.
- Cazzolli G, Škrbić T, Guella G, Faccioli P. 2013. Unfolding thermodynamics of cysteine-rich proteins and molecular thermal-adaptation of marine ciliates. Biomolecules 3:967–985. doi:10.3390/biom3040967.
- Cervantes MD, Hamilton EP, Xiong J, Lawson MJ, Yuan D et al. 2013. Selecting one of several mating types through gene segment joining and deletion in Tetrahymena thermophila. PLoS Biology 11:e1001518.
- Cervia D, Catalani E, Belardinelli MC, Perrotta C, Picchietti S, Alimenti C, Casini G, Fausto AM, Vallesi A. 2013. The protein pheromone Er-1 of the ciliate Euplotes raikovi stimulates human T-cell activity: Involvement of interleukin-2 system. Experimental Cell Research 319:56–67. doi:10.1016/j.yexcr.2012.10.007.
- Cheek S, Krishna SS, Grishin NV. 2006. Structural classification of small, disulfide-rich protein domains. Journal of Molecular Biology 359:215–237. doi:10.1016/j.jmb.2006.03.017.
- Di Giuseppe G, Barbieri M, Vallesi A, Luporini P, Dini F. 2013. Phylogeographical pattern of Euplotes nobilii, a protist ciliate with a bipolar biogeographical distribution. Molecular Ecology 22:4029–4037. doi:10.1111/mec.12363.
- Di Giuseppe G, Erra F, Dini F, Alimenti C, Vallesi A, Pedrini B, Wuthrich K, Luporini P. 2011. Antarctic and Arctic populations of the ciliate Euplotes nobilii show common pheromone-mediated cell-cell signaling and cross-mating. Proceedings of the National Academy of Sciences USA 108:3181–3186. doi:10.1073/pnas.1019432108.
- Dieckhoff HS, Freiburg M, Heckmann K. 1987. The isolation of gamones 3 and 4 of Euplotes octocarinatus. European Journal of Biochemistry 168:89–94. doi:10.1111/j.1432-1033.1987.tb13391.x.
- Dini F, Nyberg D. 1993. Sex in ciliates. In: Jones JG, editor. Advances in microbial ecology. New York: Plenum Press. pp. 85–153. doi:10.1007/978-1-4615-2858-6_3.
- Downs LE. 1956. The breeding system in Stylonychia putrina. Proceedings of the Society for Experimental Biology and Medicine 93:586–587. doi:10.3181/00379727-93-22829.
- Eisen JA, Coyne RS, Wu M, Wu D, Thiagarajan M et al. 2006. Macronuclear genome sequence of the ciliate Tetrahymena thermophila, a model eukaryote. PLoS Biology 4:1620–1642.
- Elliott AM, Nanney DL. 1952. Conjugation in Tetrahymena. Science 116:33–34. doi:10.1126/science.116.3002.33.
- Entzeroth M, Jaenicke L. 1981. Synthese von Blepharismon, dem wiedermolekularen konjugationshormon von Blepharisma japonicum. Zeitschrift für Naturforschung 36c:180–182.
- Esposito F, Ricci N, Nobili R. 1976. Mating-type-specific soluble factors (gamones) in cell interaction of conjugation in the ciliate Oxytricha bifaria. Journal of Experimental Zoology 197:275–281. doi:10.1002/jez.1401970208.
- Felici A, Alimenti C, Ortenzi C, Luporini P. 1999. Purification and initial characterization of two pheromones from the marine Antarctic ciliate, Euplotes nobilii. Italian Journal of Zoology 66:355–360. doi:10.1080/11250009909356277.
- Geralt M, Alimenti C, Vallesi A, Luporini P, Wüthrich K. 2013. Thermodynamic stability of psychrophilic and mesophilic pheromones of the protozoan ciliate Euplotes. Biology 2:142–150. doi:10.3390/biology2010142.
- Giuseppe G, Miceli C, Zahn R, Damberger F, Wuthrich K, Luporini P. 2002. A structurally deviant member of the Euplotes raikovi pheromone family: Er-23. The Journal of Eukaryotic Microbiology 49:86–92. doi:10.1111/j.1550-7408.2002.tb00347.x.
- Hartmann M, Schartau O. 1939. Untersuchungen über die Befruchtungsstoffe der Seeigel. I. Biologischen Zentralblatt 59:571–587.
- Isquith IR, Hirshfield HI. 1968. Non-Mendelian inheritance in Blepharisma intermedium. The Journal of Protozoology 15:513–516. doi:10.1111/j.1550-7408.1968.tb02165.x.
- Jahn CL, Klobutcher LA. 2002. Genome remodeling in ciliated protozoa. Annual Review of Microbiology 56:489–520. doi:10.1146/annurev.micro.56.012302.160916.
- Jiang J, Zhang Q, Warren A, Al-Rasheid KAS, Song W. 2010. Morphology and SSU rRNA gene-based phylogeny of two marine Euplotes species, E. orientalis spec. nov. and E. raikovi Agamaliev, 1966 (Ciliophora, Euplotida). European Journal of Protistology 46:121–132. doi:10.1016/j.ejop.2009.11.003.
- Karlson P, Lüscher M. 1959. ‘Pheromones’: A new term for a class of biologically active substances. Nature 183:55–56. doi:10.1038/183055a0.
- Kimball RF. 1942. The nature and inheritance of mating types in Euplotes patella. Genetics 27:269–285.
- Kosaka T. 1990. Methods for inducing selfing, selfing and its role in the life cycle of Euplotes woodruffi syngen 3 (Ciliophora). Journal of Eukaryotic Microbiology 37:33–39.
- Kubota T, Tokoroyama T, Tsukuda Y, Koyama H, Miyake A. 1973. Isolation and structure determination of blepharismin, a conjugation initiating gamone in the ciliate Blepharisma. Science 179:400–402. doi:10.1126/science.179.4071.400.
- Kuhlmann H-W, Brünen-Nieweler C, Heckmann K. 1997. Pheromones of the ciliate Euplotes octocarinatus not only induce conjugation but also function as chemoattractants. The Journal of Experimental Zoology 277:38–48. doi:10.1002/(SICI)1097-010X(19970101)277:1<38::AID-JEZ4>3.0.CO;2-C.
- Kuhlmann H-W, Sato K. 1993. Interspecific mating reactions between Euplotes octocarinatus and Euplotes patella syngen 2. European Journal of Protistology 29:24–31. doi:10.1016/S0932-4739(11)80293-2.
- Liu A, Luginbühl P, Zerbe O, Ortenzi C, Luporini P, Wüthrich K. 2001. NMR structure of the pheromone Er-22 from Euplotes raikovi. Journal of Biomolecular NMR 19:75–78. doi:10.1023/A:1008332922256.
- Luginbühl P, Ottiger M, Mronga S, Wüthrich K. 1994. Structure comparison of the pheromones Er-1, Er-10, and Er-2 from Euplotes raikovi. Protein Science 3:1537–1546. doi:10.1002/pro.5560030919.
- Luporini P, Alimenti C, Ortenzi C, Luporini P. 2005. Ciliate mating types and their specific protein pheromones. Acta Protozoologica 44:89–101.
- Luporini P, Miceli C. 1986. Mating pheromones. In: Gall JG, editor. The molecular biology of ciliated protozoa. New York: Academic Press. pp. 263–299.
- Luporini P, Miceli C, Ortenzi C. 1983. Evidence that the ciliate Euplotes raikovi releases mating inducing factors (gamones). Journal of Experimental Zoology 226:1–9. doi:10.1002/jez.1402260102.
- Luporini P, Raffioni S, Concetti A, Miceli C. 1986. The ciliate Euplotes raikovi heterozygous at the mat genetic locus coreleases two individual species of mating pheromone: Genetic and biochemical evidence. Proceedings of the National Academy of Sciences USA 83:2889–2893. doi:10.1073/pnas.83.9.2889.
- Miceli C, Concetti A, Luporini P. 1983. Isolation of the mating-inducing factor of the ciliate Euplotes. Experimental Cell Research 149:593–598. doi:10.1016/0014-4827(83)90371-3.
- Miceli C, La Terza A, Bradshaw RA, Luporini P. 1991. Structural characterization of mating pheromone precursors of the ciliate protozoan Euplotes raikovi. High conservation of pre and pro regions versus high variability of secreted regions. European Journal of Biochemistry 202:759–764. doi:10.1111/j.1432-1033.1991.tb16430.x.
- Miceli C, La Terza A, Bradshaw RA, Luporini P. 1992. Identification and structural characterization of a cDNA clone encoding a membrane-bound form of the polypeptide pheromone Er-1 in the ciliate protozoan Euplotes raikovi. Proceedings of the National Academy of Sciences USA 89:1988–1992. doi:10.1073/pnas.89.5.1988.
- Miceli C, Luporini P, Bracchi P. 1981. Morphological description, breeding system, and nuclear changes during conjugation of Euplotes raikovi Agamaliev from Mediterranean Sea. Acta Protozoologica 20:215–224.
- Miyake A. 1974. Cell interaction in conjugation of ciliates. Current Topics in Microbiology and Immunology. 64:49–77.
- Miyake A. 1981. Physiology and biochemistry of conjugation in ciliates. In: Levandowsky M, Hutner SH, editors. Biochemistry and physiology of protozoa. 2nd ed. New York: Academic Press. pp. 125–198.
- Miyake A, Beyer J. 1973. Cell interaction by means of soluble factors (gamones) in conjugation of Blepharisma intermedium. Experimental Cell Research 76:15–24. doi:10.1016/0014-4827(73)90413-8.
- Miyake A, Beyer J. 1974. Blepharmone: A conjugation-inducing glycoprotein in the ciliate Blepharisma. Science 185:621–623. doi:10.1126/science.185.4151.621.
- Miyake A, Bleyman L K. 1976. Gamones and mating types in the genus Blepharisma and their possible taxonomic application. Genetical Research 27:267–275. doi:10.1017/S0016672300016451.
- Möllenbeck M, Heckmann K. 1999. Characterization of two genes encoding a fifth so far unknown pheromone of Euplotes octocarinatus. European Journal of Protistology 35:225–230. doi:10.1016/S0932-4739(99)80041-8.
- Mronga S, Luginbühl P, Brown LR, Ortenzi C, Luporini P, Bradshaw RA, Wüthrich K. 1994. The NMR solution structure of the pheromone Er-1 from the ciliated protozoan Euplotes raikovi-NMR structure of the pheromone Er-1. Protein Science 3:1527–1536. doi:10.1002/pro.5560030918.
- Nobili R, Luporini P, Dini F. 1978. Breeding system, species relationships and evolutionary trends in some marine species of Euplotidae (Ciliata Hypotrichida). In: Battaglia B, Beardmore J, editors. Marine Organisms: Genetics, Ecology and Evolution. New York: Plenum Press. pp. 591–616.
- Ortenzi C, Alimenti C, Vallesi A, Di Pretoro B, La Terza A, Luporini P. 2000. The autocrine mitogenic loop of the ciliate Euplotes raikovi: The pheromone membrane-bound forms are the cell binding sites and potential signaling receptors of soluble pheromones. Molecular Biology of the Cell 11:1445–1455. doi:10.1091/mbc.11.4.1445.
- Ortenzi C, Luporini P. 1995. Competition among homologous polypeptide pheromones of the ciliate Euplotes raikovi for binding to each other’s cell receptors. The Journal of Eukaryotic Microbiology 42:242–248. doi:10.1111/j.1550-7408.1995.tb01573.x.
- Ottiger M, Szyperski T, Luginbühl P, Ortenzi C, Luporini P, Bradshaw RA, Wüthrich K. 1994. The NMR solution structure of the pheromone Er-2 from the ciliated protozoan Euplotes raikovi. Protein Science 3:1515–1526. doi:10.1002/pro.5560030917.
- Pedrini B, Placzek WJ, Koculi E, Alimenti C, La Terza A, Luporini P, Wüthrich K. 2007. Cold-adaptation in sea-water-borne signal proteins: Sequence and NMR structure of the pheromone En-6 from the Antarctic ciliate Euplotes nobilii. Journal of Molecular Biology 372:277–286. doi:10.1016/j.jmb.2007.06.046.
- Phadke SS, Zufall RA. 2009. Rapid diversification of mating systems in ciliates. Biological Journal of the Linnean Society 98:187–197. doi:10.1111/j.1095-8312.2009.01250.x.
- Placzek WJ, Etezady-Esfarjani T, Herrmann T, Pedrini B, Peti W, Alimenti C, Luporini P, Wüthrich K. 2007. Cold-adapted signal proteins: NMR structures of pheromones from the Antarctic ciliate Euplotes nobilii. IUBMB Life 59:578–585. doi:10.1080/15216540701258165.
- Raffioni S, Luporini P, Chait BT, Disper SS, Bradshaw RA. 1988. Primary structure of the mating pheromone Er-1 of the ciliate Euplotes raikovi. Journal of Biological Chemistry 263:18152–18159.
- Raffioni S, Miceli C, Vallesi A, Chowdhury SK, Chait BT, Luporini P, Bradshaw RA. 1992. Primary structure of Euplotes raikovi pheromones: Comparison of five sequences of pheromones from cells with variable mating interactions. Proceedings of the National Academy of Sciences USA 89:2071–2075. doi:10.1073/pnas.89.6.2071.
- Sato K. 1983. Induction of selfing by cell-free fluid from crosses in Euplotes patella syngen 2. Japanise Journal of Protozoology 16:20–21.
- Singh DP, Saudemont B, Guglielmi G, Arnaiz O, Goût J-F, Prajer M, Potekhin A, Przybòs E, Aubusson-Fleury A, Bhullar S, Bouhouche K, Lhuillier-Akakpo M, Tanty V, Blugeon C, Alberti A, Labadie K, Aury J-M, Sperling L, Duharcourt S, Meyer E. 2014. Genome-defence small RNAs exapted for epigenetic mating-type inheritance. Nature 509:447–452. doi:10.1038/nature13318.
- Sonneborn TM. 1978. Genetics of cell-cell interactions in ciliates. In: Lerner RA, Bergsma D, editors. The molecular basis of cell-cell interaction. New York: Alan R Riss. pp. 417–427.
- Sugiura M, Harumoto T. 2001. Identification, characterization, and complete amino acid sequence of the conjugation-inducing glycoprotein (blepharmone) in the ciliate Blepharisma japonicum. Proceedings of the National Academy of Sciences USA 98:14446–14451. doi:10.1073/pnas.221457698.
- Sugiura M, Shiotani H, Suzaki T, Harumoto T. 2010. Behavioural changes induced by the conjugation-inducing pheromones, gamone 1 and 2, in the ciliate Blepharisma japonicum. European Journal of Protistology 46:143–149. doi:10.1016/j.ejop.2010.01.002.
- Tavrovskaja MV. 1979. Intraspecific intercellular interactions in the ciliate Dileptus anser. Journal of Protozoology 26:35A–36A.
- Valbonesi A, Ortenzi C, Luporini P. 1992. The species problem in a ciliate with a high-multiple mating type system, Euplotes crassus. The Journal of Protozoology 39:45–54. doi:10.1111/j.1550-7408.1992.tb01282.x.
- Vallesi A, Alimenti C, Di Giuseppe G, Dini F, Pedrini B, Wüthrich K, Luporini P. 2010b. The water-born protein pheromones of the polar protozoan ciliate, Euplotes nobilii: Coding genes and molecular structures. Polar Science 4:237–244. doi:10.1016/j.polar.2010.03.007.
- Vallesi A, Alimenti C, Federici S, Di Giuseppe G, Dini F, Guella G, Luporini P. 2014. Evidence for gene duplication and allelic codominance (not hierarchical dominance) at the mating type locus of the ciliate, Euplotes crassus. Journal of Eukaryotic Microbiology 61: doi:10.1111/jeu.12140.
- Vallesi A, Alimenti C, La Terza A, Di Giuseppe G, Dini F, Luporini P. 2009. Characterization of the pheromone gene family of an Antarctic and Arctic protozoan ciliate, Euplotes nobilii. Marine Genomics 2:27–32. doi:10.1016/j.margen.2009.04.001.
- Vallesi A, Alimenti C, Pedrini B, Di Giuseppe G, Dini F, Wüthrich K, Luporini P. 2012. Coding genes and molecular structures of the diffusible signalling proteins (pheromones) of the polar ciliate, Euplotes nobilii. Marine Genomics 8:9–13. doi:10.1016/j.margen.2012.03.004.
- Vallesi A, Ballarini P, Di Pretoro B, Alimenti C, Miceli C, Luporini P. 2005. The autocrine, mitogenic pheromone-receptor loop of the ciliate Euplotes raikovi: Pheromone-induced receptor internalization. Eukaryotic Cell 4:1221–1227. doi:10.1128/EC.4.7.1221-1227.2005.
- Vallesi A, Di Pretoro B, Ballarini P, Apone F, Luporini P. 2010a. A novel protein kinase from the ciliate Euplotes raikovi with close structural identity to the mammalian intestinal and male-germ cell kinases: Characterization and functional implications in the autocrine pheromone signaling loop. Protist 161:250–263. doi:10.1016/j.protis.2009.12.002.
- Vallesi A, Giuli G, Bradshaw RA, Luporini P. 1995. Autocrine mitogenic activity of pheromones produced by the protozoan ciliate Euplotes raikovi. Nature 376:522–524. doi:10.1038/376522a0.
- Vallesi A, Giuli G, Ghiara P, Scapigliati G, Luporini P. 1998. Structure-function relationships of pheromones of the ciliate Euplotes raikovi with mammalian growth factors: Cross-reactivity between Er-1 and interleukin-2 systems. Experimental Cell Research 241:253–259. doi:10.1006/excr.1998.4056.
- Weischer A, Freiburg M, Heckmann K. 1985. Isolation and partial characterization of gamone 1 of Euplotes octocarinatus. FEBS Letters 191:176–180. doi:10.1016/0014-5793(85)80003-X.
- Weise L. 1961. Gamone. Fortschritte der Zoologie 13:119–145.
- Weiss MS, Anderson DH, Raffioni S, Bradshaw RA, Ortenzi C, Luporini P, Eisenberg D. 1995. A cooperative model for receptor recognition and cell adhesion: Evidence from the molecular packing in the 1.6-Å crystal structure of the pheromone Er-1 from the ciliated protozoan Euplotes raikovi. Proceedings of the National Academy of Sciences USA 92:10172–10176. doi:10.1073/pnas.92.22.10172.
- Yi Z, Kartz LA, Song W. 2012. Assessing whether alpha-tubulin sequences are suitable for phylogenetic reconstruction of Ciliophora with insights into its evolution in euplotids. PLoS ONE 7: e40635. doi:10.1371/journal.pone.0040635.
- Yudin AL, Afon’kin SY, Parfenova EU. 1990. Mating pheromones in the ciliate Dileptus anser. Tsitologyia 32:107–116. (In Russian with English summary).
- Zahn R, Damberger F, Ortenzi C, Luporini P, Wüthrich K. 2001. NMR structure of the Euplotes raikovi pheromone Er-23 and identification of its five disulfide bonds. Journal of Molecular Biology 313:923–931. doi:10.1006/jmbi.2001.5099.