Abstract
The composition and structure of epifaunal assemblages associated with the non-native Gracilaria vermiculophylla (Rhodophyta; Florideophyceae: Gracilariales) was analyzed and compared to those associated with the native Ulva rigida (Chlorophyta; Ulvophyceae: Ulvaceae). In a shallow coastal lagoon of the northwestern Adriatic Sea, we collected samples of each species from two different sites over a 5-year period. Epifaunal assemblages differed according to the habitat attributes provided by each type of seaweed. Amphipods comprised most of the epifanual abundance on U. rigida, while gastropods comprised it on G. vermiculophylla. The architecturally more complex G. vermiculophylla supported higher species richness and diversity of associated macrofauna. Differences in structural complexity of the two seaweeds (irregularly branched vs flat sheet-like thalli) allow different (taxonomically and functionally) epifaunal assemblages.
Introduction
Gracilaria vermiculophylla (Ohmi) Papenfuss (Florideophyceae: Gracilariales), is an invasive Rhodophyta recently recorded in the Po Delta lagoons (Sfriso et al. Citation2010). The species, which is native to Japan and Korea, was first recorded on the northern coasts of the Atlantic Sea in 2002 (Rueness Citation2005; Thomsen et al. Citation2007) and has rapidly colonised many European coasts and the lagoons of the Northern Adriatic Sea (Sfriso et al. Citation2012). In the Adriatic Sea, the most probable introduction vectors were clam farms, which imported the Manila clam Ruditapes philippinarum (Adams & Reeve, 1850) (Sfriso et al. Citation2012). This coarsely branched seaweed is particularly well adapted to low-energy, shallow soft bottom environments, like lagoons, harbours and inlets (Rueness Citation2005).
In the marine environment, seaweeds are of great ecological importance, particularly as primary producers and as habitat formers, providing space, shelter and food for a variety of associated epifaunal organisms. Several papers have dealt with the role of marine seaweeds in structuring epifaunal assemblages, some of them comparing how different species of macroalgae influence the abundance, richness and diversity of associated assemblages (Chemello & Milazzo Citation2002). Seaweeds are three-dimensional biogenic habitats with a structural complexity that changes between and within macroalgal species. We may expect that patterns of composition and abundance of associated epifaunal organisms are influenced by the arrangement of structural elements within the habitat (Gee & Warwick Citation1994).
This study, carried out in a low-energy lagoon in the Northern Adriatic, focused on assemblages of epifauna associated with the invasive G. vermiculophylla, in comparison with the epifauna associated to the most common native seaweed Ulva rigida C. Agardh, 1822 (Chlorophyta; Ulvophyceae: Ulvaceae). No spatial and/or temporal studies of species composition and abundance of the macrofauna associated with seaweeds have been conducted in the Northern Adriatic lagoons. Our main goal was to analyse the composition and abundance of epifaunal assemblages associated with two habitat-forming algae, the non-native G. vermiculophylla and the native U. rigida. The two species have differences in structure; thus, distinctly different macrofaunal responses might be expected, with the more structurally complex G. vermiculophylla harbouring more abundant and diverse assemblages of invertebrates. In this study, we took advantage of the presence of the two seaweeds in the same environment (an Adriatic shallow lagoon) to test the following hypotheses: (i) the composition of macrofaunal (> 0.5 mm) assemblages associated with the two seaweeds differ because they have different structure; (ii) at a small spatial scale of observation, higher levels of habitat architecture (e.g. seaweed structure) support higher community abundance and diversity; (iii) patterns of composition and community structure of macrofaunal assemblages associated with the two seaweeds are consistent over a long time frame (5 years).
Materials and methods
Study site
The Marinetta Lagoon () is a microtidal, shallow water body (average depth 1 m) located in the northernmost part of the Po River Delta. The lagoon is connected to the Adriatic Sea by a quite narrow mouth (about 100 m wide), and has a surface area of 11.5 km2. It is grossly divided into two parts by the terminal trait of the Po di Levante, a secondary deltaic branch of the Po River. The lagoon receives fresh water through the Po di Levante, whose discharge is controlled by a sluice and is regulated according to rainfall patterns. The western part of the lagoon has sandy bottoms, and part of it is utilised to rear the Manila clam Ruditapes philippinarum, while the eastern part of the lagoon is characterised by shelly–muddy bottoms and by reduced hydrodynamism; the annual salinity range is 29–11 (Vincenzi et al. Citation2014), depending on fresh water discharge from the Po di Levante and, thus, from precipitation.
Field methods
In the Marinetta Lagoon, we chose two sites dominated by the two seaweeds. Site G (45°02ʹ47.78ʹʹN; 12°22ʹ09.73ʹʹE) had a depth of 100 cm, and was dominated by Gracilaria vermiculophylla. Site U (45.03ʹ19.11ʹʹN; 12°22ʹ46.45ʹʹE) was shallower (40 cm depth) and was dominated by Ulva rigida. The main environmental conditions (salinity, dissolved oxygen, sediment texture) at the two sites were similar, with site G slightly more confined (sensu Guelorget & Perthuisot Citation1983) than site U (Mistri Citation2010).
Seaweeds and associated macrofauna samples were collected according to McIntyre et al. (Citation1984), by pulling a cylinder (radius: 15 cm) with one end closed by a net of 0.5-mm mesh horizontally for 100 cm of path length (volume of water sampled: 70 L) through the seaweed mat. Each sample was carefully placed into a plastic bag and taken to the laboratory for further sorting. Using this procedure, we were able to sample motile organisms associated with seaweeds. Each site was sampled in triplicate with seasonal frequency, from 2006 to 2010. In the laboratory, the seaweeds were washed and then sieved through 0.5-mm mesh to recover macrofaunal organisms. We also scrutinized the entire thalli of seaweeds to find the epibionts attached to them. Then, all organisms collected were identified to the lowest possible taxonomic level. Each species was assigned a feeding strategy, based on literature data; four major trophic groups were distinguished: suspension feeders (SF), deposit feeders (DF), predators (P) and herbivores (H).
Dry weight (DW) of seaweeds was calculated after drying them for 48 h at 60°C.
Data analysis
Non-parametric permutational multivariate analysis of variance (PERMANOVA; Anderson et al. Citation2008) was used to test hypotheses about differences of epifaunal assemblages. This approach partitions the variability in the original dissimilarity matrix according to the full multifactorial design, with tests of individual terms obtained using permutations. Analyses followed a three-factor orthogonal design, with two habitats (G. vermiculophylla and U. rigida; fixed), four seasons (spring, summer, fall and winter; fixed), and 5 years (2006–2010; random). P-values were provided using unrestricted (9999) permutation of raw abundance data. When low unique values in the permutation distribution were available, asymptotical Monte Carlo P-values were used instead of permutational P-values. In addition, responses of assemblages were visualized by non-metric multidimensional scaling (nMDS) on the basis of Bray–Curtis dissimilarity matrices on fourth-root transformed density data. The SIMPER (similarity percentage) procedure was used to identify the percentage contribution of each taxon to the Bray–Curtis dissimilarity between the averages of groups. Taxa were considered important if their contribution to percentage dissimilarity was > 2%. Multivariate analyses were conducted using Primer v. 6 and PERMANOVA (Primer-E Ltd., Plymouth, UK).
Changes in number of species (S), number of individuals (N) and diversity (Shannon–Wiener’s H’) were analysed using analyses of variance (ANOVA). When significant differences between main factors or their interactions were found, Tukey’s HSD (honest significant difference) tests were used as a posteriori comparison. The homogeneity of variances was examined using Levene’s test, and data were transformed when necessary to remove heteroscedasticity (Underwood Citation1997).
Results
In our field survey, seaweed highest average biomass was 85.9 (± 28 standard deviation, SD) g DW 70 L−1 for Gracilaria vermiculophylla, and 65.7 (± 15.2 SD) g DW 70 L−1 for Ulva rigida (). G. vermiculophylla and U. rigida biomass significantly influenced total macrofaunal abundance, and at both sites, significantly positive patterns (site G: r = 641; site U: r = 0.709; both P < 0.05) were shown. For this reason, the density of each macrofaunal taxon was expressed as the number of animals per g DW of seaweed.
Figure 2. Average amount of macroalgae (as gDW) at the study sites (site G: StG; site U: StU; black: Gracilaria vermiculophylla; Grey: Ulva rigida; bars are SD). Ma06: March 2006; Ju06: June 2006; etc.
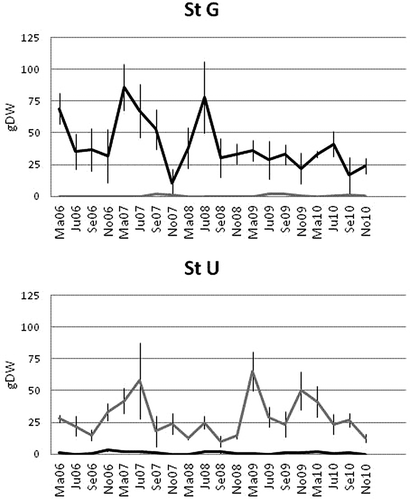
shows the average taxonomic composition of faunal community at the two sites. Three groups (in decreasing order of overall abundance: Amphipoda, Mollusca and Polychaetes) accounted for over 95% of all individuals observed. At site U, amphipods comprised most of the epifaunal abundance, accounting for 61% of the total. Bivalves were the second most numerous epifauna at 21% of total. At site G, conversely, gastropods (34% of total) were the most numerous epifauna, followed by amphipods (25.7%), bivalves (25.6%) and polychaetes (11%). At both sites, an increase in abundance of the invasive species Arcuatula senhousia (Benson in Cantor, 1842) (Mollusca: Mytilidae) was registered through time, with more than 10 individuals (ind) g DW in the warmer months of 2010 at site U.
Figure 3. Average composition of epifaunal assemblages at each sampling site (site G: StG; site U: StU; bars are SD).
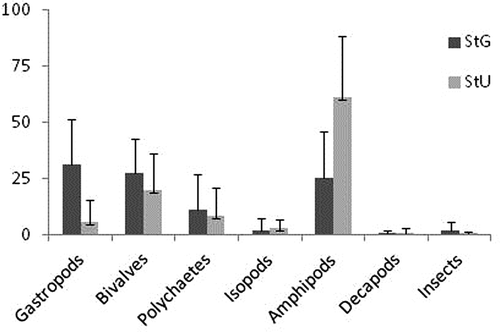
Epifaunal assemblages differed to the habitat attributes provided by each type of seaweed. In fact, the composition of epifaunal assemblages varied significantly between the two sites (PERMANOVA; ); there were significant differences over time, particularly between warm and cold seasons of each year (pairwise tests not reported for brevity). Only in spring 2009 and 2010, the epibenthic assemblages at the two sites did not show significant differences in composition (pairwise test, t = 1.91 and t = 1.84 respectively, both P = 0.07). The non-metric multidimensional scaling (nMDS) plot () shows a clear separation of epifaunal communities from the two sites, with G. vermiculophylla points on the right and U. rigida on the left side of the plot. Dissimilarity between the two sites was 71.24%. The contribution from epifaunal species abundance to the average Bray–Curtis dissimilarity between the sites U and G (through SIMPER analysis; ) showed that differences were mainly due to the average higher proportion of the amphipods Gammarus aequicauda (Martynov, 1931) and Microdeutopus gryllotalpa Costa, 1853, together with A. senhousia, at site U, and the gastropod Ecrobia ventrosa (Montagu, 1803) at site G. At site U, eight taxa contributed to a cumulative similarity of 91.7%, while there were 10 taxa at site G ().
Table I. PERMANOVA: result of main test from unrestricted permutation of raw data.
Table II. Summary of differences in abundance of major epifaunal taxa found on Gracilaria versus Ulva habitats.
Figure 4. Ordination plot of epifaunal assemblages. Point acronyms: year (7: 2007; 8: 2008; etc.); site (G and U), and time (ma: March; ju: June; etc.) of sampling (black triangle: Gracilaria vermiculophylla; white triangle: Ulva rigida).
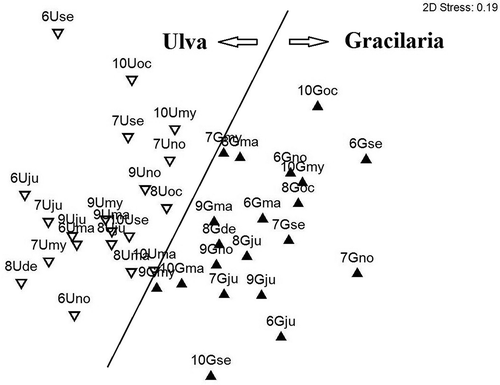
Average species richness (S), abundance (N) and diversity (H’) at the two sites are shown in . Epifaunal assemblages at site G showed a significant higher species richness and diversity (ANOVA; both P = 0.001) with respect to site U.
Figure 5. Epifaunal community descriptors (average values) at the two sites (site G: StG; site U: StU): S, number of species; N, abundance (as N ind g DW−1), and H’, diversity. March 2006: 6ma; June 2006: 6ju; etc.
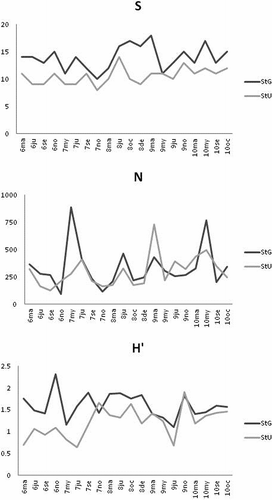
In , the average community composition by trophic groups is shown. Site G was dominated by deposit feeders (41.1%) and by suspension feeders (36.4%), while site U was dominated by herbivores (59.4%) followed by suspension feeders (32.1%), thus reflecting the numerical dominance of different taxa (gastropods at G, and amphipods at U) at the two sites.
Discussion
During our study period, macroalgal mats at the two sites in Marinetta Lagoon were present in relatively small amounts (compared to blooms reported, for example, in Ye et al. Citation2011), and probably for this reason, adverse effects on the biota (e.g. Raffaelli et al. Citation1998) have not been observed. The results of this study indicated that our initial hypotheses were partially supported. Macrofaunal assemblages associated with G. vermiculophylla and U. rigida differed. Macrofaunal assemblages associated with the non-native G. vermiculophylla were more diverse (its morphology created a three-dimensional mosaic of structures and interstitial spaces for different types of invertebrates to occupy), but not more abundant. Patterns of community structure were consistent over time, but community composition changed, mostly due to the spread of the non-indigenous bivalve Arcuatula senhousia.
The importance of seaweed communities as habitats in temperate coastal waters is principally attributed to their complex, three-dimensional structure (Norkko et al. Citation2000). In Marinetta Lagoon, the seaweeds G. vermiculophylla and U. rigida provide extensive vegetative structure on sand and mudflats that are otherwise devoid of macrophyte structure (Sfriso et al. Citation2012). This study has shown that large numbers of epifaunal individuals are associated with them. The most likely sources for colonisation are ambient benthic fauna, and pelagic larvae. Three groups (Amphipoda, Mollusca and Polychaetes) dominated, in terms of abundance, the epifaunal assemblages inhabiting G. vermiculophylla and U. rigida thalli. Our results also show that epifaunal abundances increase with increasing mass of seaweeds, probably reflecting the area provided by them. Byers et al. (Citation2012) also observed that the abundance of G. vermiculophylla epifauna increased with algal biomass in estuarine mudflats of southeastern USA.
Multivariate analysis indicated that structure of epifaunal assemblages differed between seaweeds. Previous works obtained similar results when comparing how different species of macroalgae modulated species richness and diversity of assemblages associated (Chemello & Milazzo Citation2002). Other studies found differences in composition and structure of epifaunal assemblages associated with native and invasive seaweed species, with native species generally supporting higher species richness and diversity (Wikström & Kautsky Citation2004). In our study case, however, other factors rather than the origin of species (i.e. native vs invasive) probably play an important role. On average, species richness and diversity of macrofauna living on the native U. rigida was significantly lower than those on the architecturally more complex, invasive G. vermiculophylla. Thomsen et al. (Citation2013) found that G. vermiculophylla had a strong positive effect on habitat-associated invertebrates in Odense Fjord (Denmark).
The relationship we observed between the two seaweed species and their associated epifauna may be a general pattern, in which more complex thalli like those of G. vermiculophylla encourage a more diversified associated epifauna than do simple and flat-thalloid algae like U. rigida. Cardoso et al. (Citation2004), for example, found richer macrofaunal communities associated to complex G. verrucosa than to flat Ulva intestinalis Linnaeus, 1753 thalli in Mondego Estuary. Schreider et al. (Citation2003) indicated morphological complexity as a key factor in structuring macrobenthic assemblages associated to the brown seaweeds Sargassum globulariaefolium J. Agardh, 1889 and Hormosira banksii (Turner) Decaisne, 1842. It has been suggested that structural differences in habitat can affect numbers of epifauna (Gee & Warwick Citation1994). In the present study, however, structural complexity of seaweeds generally did not influence abundances of epifauna, because there was no difference in abundance of epifauna between the complex G. vermiculophylla and the architecturally simpler U. rigida. Cacabelos et al. (Citation2010) hypothesized that complexity of seaweeds might not be a consistent predictor of number of species of amphipods.
Habitat architecture may influence the composition of the associated fauna through (i) decrement of mortality rate due to predation (“refuge effect”), (ii) decrease of hydrodynamic features (“shelter effect”) and (iii) collectors of species (“filter effect”), strictly related to hydrodynamic processes and the larval supply (Chemello & Milazzo Citation2002). In our study, differences in epifaunal assemblages can be attributed to different seaweed architectures. It is known (Orth Citation1992) that structural complexity of seaweeds may stabilise predator–prey interactions due to the refuge effect. During sampling campaigns, we often collected pipefish (Syngnathus abaster Risso, 1827), and gobies [Knipowitschia panizzae (Verga, 1841), and Pomatoschistus spp.] together with seaweeds, and mostly in the U. rigida habitat. In the Po Delta lagoons, Syngnathidae and Gobiidae are very common and highly related with seaweed-dominated communities (Franzoi et al. Citation1993). It is known that those fish prey on small-sized invertebrates (Ceccherelli et al. Citation1994), including phytal amphipods and polychaetes (Franzoi et al. Citation1993). The more diverse and species-rich assemblages of the most complex G. vermiculophylla habitat may reflect lower predation pressure. Likely due to shelter and collector effects provided by the finely branched G. vermiculophylla, smaller-sized organisms (e.g. Ecrobia ventrosa and Monocorophium insidiosum Crawford, 1937) may have selected their host on the basis of its architectural complexity. Similar results were found by Johnston and Lipcius (Citation2012), and Thomsen et al. (Citation2013).
Considering feeding preferences, herbivorous species such as Gammarus aequicauda and other amphipods that feed mainly on the microphytobenthic film (phytal fauna do not generally feed on the host-plant tissues; Gee & Warwick Citation1994) were found to select the thin sheet-like thalli of U. rigida. It is known (Wikström & Kautsky Citation2004) that epiphytic load may play an important role in determining the structure of phytal assemblages. Among suspension-feeding organisms, some (e.g. Cerastoderma glaucum Bruguière, 1789) were found almost exclusively associated to G. vermiculophylla, while others (e.g. Mytilus galloprovincialis Lamarck, 1819, Ficopomatus enigmaticus Fauvel, 1923) seemed to select U. rigida.
This study carried out at a local scale demonstrated that the two seaweeds supported diversified epifaunal assemblages. The structural complexity of the algae seemed to be of major importance for the structure of epifaunal assemblages. The habitat heterogeneity hypothesis assumes that structurally complex habitats offer more niches and diverse ways of exploiting environmental resources, allowing more species to coexist (MacArthur & Wilson Citation1967). In Marinetta Lagoon, the invasive G. vermiculophylla and the native U. rigida determine the three-dimensional physical structure of the habitat, which is otherwise made up of bare mudflats, providing a heterogeneous habitat for a variety of associated animals. Differences in structural complexity of the two seaweeds (irregularly branched vs flat, sheet-like thalli) allowed different (taxonomically and functionally) epifaunal assemblages.
Acknowledgements
We are grateful to the fishermen of the Delta Nord Consortium (Rosolina, Rovigo) for logistic support during sampling campaigns. Two anonymous reviewers are acknowledged for constructive criticism.
References
- Anderson MJ, Gorley RN, Clarke KR. 2008. PERMANOVA+ for PRIMER: Guide to software and statistical methods. Plymouth, UK: PRIMER-E Ltd.
- Byers JE, Gribben PE, Yeager C, Sotka EE. 2012. Impacts of an abundant introduced ecosystem engineer within mudflats of the southeastern US coast. Biological Invasions 14:2587–2600. doi:10.1007/s10530-012-0254-5.
- Cacabelos E, Olabarria C, Incera M, Troncoso JS. 2010. Effects of habitat structure and tidal height on epifaunal assemblages associated with macroalgae. Estuarine Coastal and Shelf Science 89:43–52. doi:10.1016/j.ecss.2010.05.012.
- Cardoso PG, Pardal MA, Raffaelli D, Baeta A, Marques JC. 2004. Macroinvertebrate response to different species of macroalgal mats and the role of disturbance history. Journal of Experimental Marine Biology and Ecology 308:207–220. doi:10.1016/j.jembe.2004.02.018.
- Ceccherelli VU, Mistri M, Franzoi P. 1994. Predation impact on the meiobenthic harpacticoid Canuella perplexa in a lagoon of the Po River Delta, Italy. Estuaries 17:283–287. doi:10.2307/1352577.
- Chemello R, Milazzo M. 2002. Effect of algal architecture on associated fauna: Some evidence from phytal molluscs. Marine Biology 140:981–990. doi:10.1007/s00227-002-0777-x.
- Franzoi P, Maccagnani R, Rossi R, Ceccherelli VU. 1993. Life cycles and feeding habits of Syngnathus taenionotus and S. abaster (Pisces, Syngnathidae) in a brackish bay of the Po River Delta (Adriatic Sea). Marine Ecology Progress Series 97:71–81. doi:10.3354/meps097071.
- Gee JM, Warwick RM. 1994. Metazoan community structure in relation to the fractal dimensions of marine macroalgae. Marine Ecology Progress Series 103:141–150. doi:10.3354/meps103141.
- Guelorget O, Perthuisot JP. 1983. Le domaine paralique-expression géologiques, biologiques et économiques du confinement. Travaux du Laboratoire de Géologie, Ecole Normale Supérieure de Paris 16:1–136.
- Johnston CA, Lipcius RN. 2012. Exotic macroalga Gracilaria vermiculophylla provides superior nursery habitat for native blue crab in Chesapeake Bay. Marine Ecology Progress Series 467:137–146. doi:10.3354/meps09935.
- MacArthur RH, Wilson EO. 1967. The theory of island biogeography. Princeton: Princeton University Press.
- McIntyre AD, Elliott JM, Ellis DV. 1984. Introduction: Design of sampling programmes. In: Holme NA, McIntyre AD, editors. Methods for the study of marine benthos. Oxford: Blackwell Scientific Publications. pp. 1–26.
- Mistri M. 2010. Carta ittica provinciale delle aree lagunari e vallive (zona C). Bologna: Greentime. 157 pp.
- Norkko J, Bonsdorff E, Norkko A. 2000. Drifting algal mats as an alternative habitat for benthic invertebrates: Species specific responses to a transient resource. Journal of Experimental Marine Biology and Ecology 248:79–104. doi:10.1016/S0022-0981(00)00155-6.
- Orth RJ. 1992. A perspective on plant-animal interactions in seagrasses: Physical and biological determinants influencing plant and animal abundance. In: John DM, Hawkins SJ, Price JH, editors. Plant-animal interaction in the marine benthos. Oxford: Claredon. pp. 147–164.
- Raffaelli D, Raven JA, Poole LA. 1998. Ecological impact of green macroalgal blooms. Oceanography and Marine Biology 36:97–125.
- Rueness J. 2005. Life history and molecular sequences of Gracilaria vermiculophylla (Gracilariales, Rhodophyta), a new introduction to European waters. Phycologia 44:120–128. doi:10.2216/0031-8884(2005)44[120:LHAMSO]2.0.CO;2.
- Schreider MJ, Glasby TM, Underwood A. 2003. Effects of height on the shore and complexity of habitat on abundances of amphipods on rocky shores in New South Wales, Australia. Journal of Experimental Marine Biology and Ecology 293:57–71. doi:10.1016/S0022-0981(03)00185-0.
- Sfriso A, Maistro S, Andreoli C, Moro I. 2010. First record of Gracilaria vermiculophylla (Gracilariales, Rhodophyta) in the Po Delta lagoons, Mediterranean Sea (Italy). Journal of Phycology 46:1024–1027. doi:10.1111/j.1529-8817.2010.00893.x.
- Sfriso A, Wolf MA, Maistro S, Sciuto K, Moro I. 2012. Spreading and autoecology of the invasive species Gracilaria vermiculophylla (Gracilariales, Rhodophyta) in the lagoons of the north-western Adriatic Sea (Mediterranean Sea, Italy). Estuarine Coastal and Shelf Science 114:192–198. doi:10.1016/j.ecss.2012.07.024.
- Thomsen MS, Stæhr PA, Nejrup L, Schiel DR. 2013. Effects of the invasive macroalgae Gracilaria vermiculophylla on two co-occurring foundation species and associated invertebrates. Aquatic Invasions 8:133–145. doi:10.3391/ai.2013.8.2.02.
- Thomsen MS, Wernberg T, Stææhr P, Krause-Jensen D, Risgaard-Petersen N, Silliman BR. 2007. Alien macroalgae in Denmark – a broad-scale national perspective. Marine Biology Research 3:61–72. doi:10.1080/17451000701213413.
- Underwood AJ. 1997. Experiments in ecology: their logical design and interpretation using analysis of variance. Cambridge, MA: Cambridge University Press.
- Vincenzi S, De Leo G, Munari C, Mistri M. 2014. Rapid estimation of potential yield for data-poor Tapes philippinarum fisheries in North Adriatic coastal lagoons. Hydrobiologia 724:267–277. doi:10.1007/s10750-013-1742-z.
- Wikström SA, Kautsky L. 2004. Invasion of a habitat-forming seaweed: Effects on associated biota. Biological Invasions 6:141–150. doi:10.1023/B:BINV.0000022132.00398.14.
- Ye N-H, Zhang X-W, Mao Y-Z, Liang C-W, Xu D, Zou J, Zhuang Z-M, Wang Q-Y. 2011. “Green tides” are overwhelming the coastline of our blue planet: Taking the world’s largest example. Ecological Research 26:477–485. doi:10.1007/s11284-011-0821-8.