Abstract
We review the applications of REL test as a technique for detecting injury and thereby forecasting survivability of transplanted seedlings. The objectives of the present review are to present the fundamentals of this method, assess the relevant literature, present evidence of seasonal variations of REL, describe how REL responds to different stress conditions, suggest priorities for future research as well as practical recommendations for REL testing, and assess leakage of organic compounds as an indicator of root damage. Seasonal changes in REL may be connected with root freezing tolerance that varies among plants of different seed sources and species and thus does not always indicate health state of seedlings. REL technique can be used for assessing frost hardiness of roots under certain conditions (e.g. roots should be sampled into the test tubes prior to the freezing test). It can be used for studying heat stress with certain prerequisites (e.g. series of high temperatures or different exposure times should be used to find a threshold for heat tolerance). In desiccation tolerance assessment, REL presents high variation depending on species. In case of rough handling or hypoxic conditions, the effect depends on certain developmental stages. Additionally, though REL may be useful in quantifying damage caused by cold storage, it should be considered as a relative index of plant quality only since the relationship between REL and survivability could vary depending on cultural and handling practices prior to planting as well as on post-planting environmental conditions. In some cases, REL is correlated with field performance of seedlings, but in other cases the correlation is weak. Factors as species, seed lots, developmental stage of root tissue, season, and bud dormancy intensity may affect REL. Thus, REL must first be calibrated to these factors before it can be reliably used to predict the field performance of all types of seedling stock. Ambient storage, ageing and amino acids and protein leakage are also discussed. Limitations and questions for future research are suggested (e.g. species-dependence and decreasing variation). In general, REL is casually and statistically related with root damage and survivability of seedling. However, it depends on many other factors apart from root damages, and thus it remains a great challenge to improve its reliability.
Introduction
The focus of this paper is to review a method of assessing seedling post-planting survival based on root system integrity. A number of papers and reviews have discussed seedling quality (Sutton, 1979; Ritchie, 1984; Duryea 1985; Puttonen, 1989; Puttonen, 1997; Sampson et al., 1997; Tanaka et al., 1997), however, these works have focused largely on above-ground morphology and whole plant physiological vigor (Grossnickle & Folk, 1993; Mattsson, 1997; Mohammed, 1997), which determines plant growth and development. Few publications have dealt with morphological and physiological attributes of root systems which constitute the most crucial factor for the survivability (Fitter, 1991).
Root system is quite sensitive to environmental and operational stresses. Operational stresses, in particular, might impair root vitality (McKay, Citation1997). These operations may include lifting, storage, grading, insecticide treatment, packaging, dispatch, receipt, and temporary storage or combinations of these. During those operations seedlings are exposed to stresses like high and low temperatures (Lindström & Mattson, Citation1989; Stattin et al., Citation2000), desiccation (McKay & Milner, Citation2000), rough handling (McKay & White, Citation1997), storage (McKay & Mason, Citation1991; McKay, Citation1992; Harper & O'Reilly, Citation2000), and even fire (Chiatante et al., Citation2006). Assessment of fine root vitality, which seedling survivability strongly depends on, is only possible after the roots have suffered damage. However, even in such a case, damage is often invisible or can hardly be measured in a generally acceptable way. An apparently acceptable test is the root electrolyte leakage (REL). It elucidates the root cell membrane integrity as well as changes in membrane functions. Thus, it would be expected to be a valid indicator of root injury and therefore of the seedling survival potential (Ritchie & Landis, Citation2006).
REL has been applied to both fine and tap roots of tree seedlings. However, the literature on the latter is scarce and therefore the present review will focus on fine root electrolyte leakage. The fundamentals of REL will be presented. We review the applications of the REL test as a technique for detecting injury and thereby forecasting survivability of transplanted seedlings (Appendix 1). We evaluate the outputs from REL tests across several stresses. Recommendations for the use of REL test as a viability indicator and its practical use will be presented.
The objectives of the present review are as follows:
-
to present the fundamentals of this method,
-
to provide a concise assessment of literature related to the examination of REL as an indicator of tree seedling quality,
-
to present evidence of seasonal variations of REL,
-
to determine how REL responds to different stress conditions, and
-
to suggest priorities for future research and practical recommendations for REL testing.
Fundamentals of root membranes and leakage
The origins of REL test can be traced back to the early work of Wilner (Citation1955, Citation1960), but McKay and her collaborators were among the first who used REL to assess the survival potential of bare-root nursery stock (McKay & Mason, Citation1991; McKay, Citation1993; Citation1994). The method is based on the fact that in healthy tissues, a high symplastic electrolyte concentration is maintained either by selective transmittance across the membranes or by active transport by cell membrane proteins (Palta et al., Citation1977). When the transmembrane proteins of the cells are injured, then membrane permeability increases and the various components of the symplasm leak out of the cells to the apoplastic space, following the concentration gradient. When such tissues are incubated in distilled or deionised water, the apoplastic ions leak out of the tissue and may be detected by conductivity measurement of the embedding solution. The main ion that contributes to the increased conductivity is K+ (van den Driessche, Citation1969). According to the study of Kocheva et al. (Citation2005) electrolyte leakage would be a biphasic process that is affected by cell wall adsorption capacity in addition to cell membrane permeability. Ions are present in the apoplast of healthy tissues as well. Thus, ion leakage and the succeeding elevated conductivity of the embedding water may be observed also for healthy tissues, and it is affected by annual stage of development of plants as shown below. However, the degree of cellular injury affects the rate of leakage being faster for injured than for healthy tissues (Murray et al., Citation1989). The time required to reach steady state depends on the incubation conditions and probably on tissue type as well (McKay et al., Citation1999). In shoots of red spruce [Picea rubens Sarg. syn P. rubra (Du Roi) Link], an approximate steady state of leaching was reached for injured samples just after 1 week of incubation (Murray et al., Citation1989). However, distinction between non-injured and injured samples may be obtained by a shorter incubation time.
Although a number of studies (cf. Waisel, Citation1962; Vogt & Bloomfield, Citation1991) demonstrated the existence of differences in fine root functionality, no studies were found dealing with changes in REL due to the different types of roots used. Mycorrhizal fungi are often present in tree seedlings. However, it is questionable whether the mycorrhizal population of root systems has any effects on REL values. Monaghan (Citation2002) observed that fine root samples of 3-year-old Douglas fir (Pseudotsuga menziesii (Mirb.) Franco) seedlings containing Mycelium radicis atrovirens mycorrhizas had higher REL values than root samples containing Amphinema byssoides or Tuber sp. The author concluded that the differences in REL values between root samples of each mycorrhizal type may be due to the differences in the mantle structure and thickness between these mycorrhizas.
Methodology
Although REL has been extensively used, it has been slightly modified from the initial protocol described by Wilner (Citation1955) (McKay, Citation1992; Citation1998b) (). For REL test of fine roots (<2 mm diameter), root system is washed in cold tap water to remove soil and then rinsed in deionised water (pH 5 – 7) to remove adsorbed ions. Samples are selected from a particular portion of the root systems. Weight of the root samples ranges between 100 and 500 mg. Individual samples are submerged in 16 ml of distilled or deionised water in 28-ml universal glass bottles (with a ratio of tissue to water of approximately 1/20). Whether sample behaves as the whole system is always an open question in every sample-based research. However, here it is supposed that the sample presents quite similar behavior to the whole root system, because it is immediately examined. On the other hand, we do not refuse that an experimental verification could be an interesting question for future research. The bottles are capped, shaken, and left at room temperature for 24 h. shaking might be continued during the entire incubation time. Then the first conductivity (C1) of the bathing solution is measured using a conductivity probe with a built-in temperature compensation system. Samples are normally killed by autoclaving at 110°C for 10 min. The samples are then cooled to room temperature and a second conductivity (C2) measurement is taken (). REL is expressed as
Figure 1. Schematical representation of root electrolyte leakage test methodology (adapted from Ritchie and Landis, Citation2006).
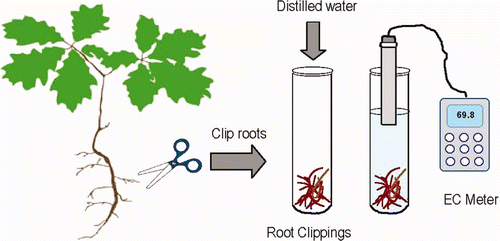
Figure 2. Root electrolyte leakage (REL) with time measured after 1, 2, 3, 4, and 5 h from the time of adding distilled water to root samples of Quercus robur L. seedlings grown in an Irish nursery. Oak roots were previously exposed to four desiccation treatments with durations of 0, 4, 6, and 8 h, according to the methodology outlined in Mortazavi et al. (Citation2004).
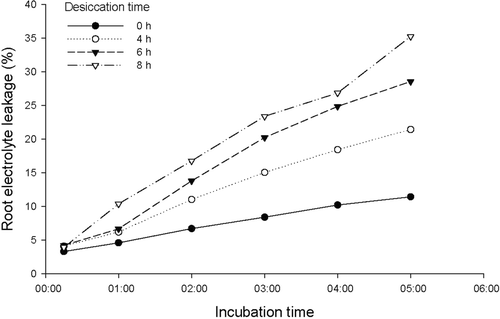
Seasonal changes in REL
REL has seasonal variation due to factors other than root damage (), probably in direct relation to the level of their physiological activity (Zhao et al., Citation1995; Folk et al., Citation1999; Harper & O'Reilly, Citation2000; Perks et al., Citation2001). REL decreased in Douglas fir [Pseudotsuga menziesii (Mirb.) Franco] seedlings from early autumn to mid-winter (McKay, Citation1993, Citation1994; O'Reilly et al., Citation2000). REL of sycamore (Acer pseudoplatanus L.) seedlings, growing in Ireland, declined from 61% in early October to 16% and 18% in January and February, respectively (Mortazavi et al., Citation2004). Such differences do not seem to be directly related to the vitality of the root cells but rather to their physiological status. Changes in REL during winter reflect changes in root membrane properties (Folk et al., Citation1999) that might affect the tolerance of the root system to lifting stress (McKay, Citation1994) During this phase of development, the selectivity of root membranes and their enzyme activity are modified (Larcher, Citation1995; Zhao et al., Citation1995; McKay, Citation1998b). In addition, given that the plasma membrane is one of the primary sites of freezing injury, membranes should be stabilized through alterations in their fluidity to effectively resist against low winter temperatures (Steponkus, Citation1984).
Figure 3. Root electrolyte leakage of Sitka spruce (Picea sitchensis B) seedlings at time of lifting over three lifting seasons in Ireland. Vertical bars on the symbols indicate standard errors, at 5% LSD of the means. (Adapted with permission from O'Reilly et al., Citation2000. © 2000 Springer).
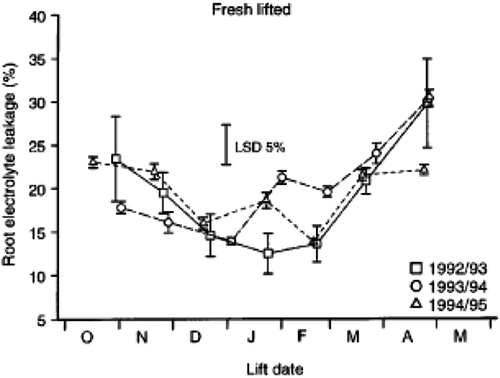
During the over-wintering period, REL varies among tree species (McKay, Citation1992, Citation1993). This may be due to different seed sources, different nursery environments, and different cultural practices (McKay, Citation1992; Lindström & Nyström, Citation1987). Seasonal variation in REL may be connected with root freezing tolerance that varies among plants of different seed sources and species (Lindström & Nyström, Citation1987). We may conclude that low and high REL values do not always indicate healthy or injured roots and the relationship between REL and seedling quality remains unclear.
Stress tolerance assessment
REL has been used to detect and quantify damage in conifer seedlings following cold storage (Mason & McKay, Citation1990; McKay, Citation1992, Citation1993) after rough handling and desiccation (McKay et al., Citation1993; McKay & White, Citation1997) and after exposure to freezing temperatures (Bigras & D'Aoust, Citation1992; McKay, Citation1993; Bigras & Calmé, Citation1994; Colombo, Citation1994; Bigras, Citation1997). The use of the method for this purpose is based on the assumption that the aforementioned stressors affect the permeability of the cell plasma membranes. Consequently, ions leak from symplast to apoplast and further to the incubation solution. The extension of cell membrane injuries are assumed to be related to REL. In the tests, whole plants, whole root systems, or samples of roots are exposed to the stress for different duration and/or at different degrees of strength. The REL test is carried out after stressing. Compared with the aerial organs, roots set special requirements for such tests because the interface between their tissues and the surrounding medium is more compact and roots have no thick cell walls and cuticles. Thus, membrane damage apparently affects ion leakage in real time and immediately after the stress impact. In the following pages, the measurement procedures and results of REL for studying thresholds of roots to various abiotic stressors (freezing, cold, heat, and desiccation) are reviewed. For clarity sake, freezing temperatures are defined wherein as <0°C, cold 0 – 5°C, cool 5 – 10°C, warm temperatures 15 – 25°C, and heat as temperatures >40°C.
Freezing stress
Frost hardiness is defined as the ability of plants to tolerate freezing temperatures without suffering irreversible damage (Lavender, Citation1984; Ritchie, Citation1984; Burr, Citation1990). The use of electrolyte leakage in frost hardiness studies of roots dates back to the beginning of 1930s when the electrical conductivity of the embedding solution of root samples was shown to increase after exposing the roots to freezing temperatures for different durations (Dexter et al., Citation1930, Citation1932). Wilner was among the first who used the electrolyte leakage method to assess frost hardiness in roots (Wilner, Citation1959, Citation1960). Thereafter, this method was used with slight modifications in several studies, mostly for above ground organs (e.g. Flint et al., Citation1967; van den Driessche, Citation1969; Murray et al., Citation1989; Burr et al., Citation1990) but also for roots (e.g. McKay, Citation1994; Ryyppö et al., Citation1998; Sutinen et al., Citation1998).
Frost hardiness assessment of roots is usually based on exposure of roots to one or to a series of freezing temperatures in a controlled freezing treatment, followed by REL test (control plants are left unfrozen). The result of the test depends on the treatment of the samples prior to the freezing test (Repo & Ryyppö, Citation2007) and on the freezing test itself, i.e. rate of cooling and warming (typically 5°C × h−1) and the degree and duration (typically 4 h) of the minimum temperatures (Levitt, Citation1980; Sakai & Larcher, Citation1987). There are basically two approaches in such tests: (i) whole-plant-freezing test where the roots are exposed to frost in situ. Sampling for the REL test is carried out after freezing. (ii) Freezing test in vitro, i.e. roots are sampled and inserted into the test tubes prior to the freezing test. The first approach has the advantage that it allows a comparison of visual injuries, at whole-plant level. Moreover, in situ freezing test approaches real conditions, and thus yields relatively unbiased results of hardiness. On the other hand, a shortcoming of this approach is that ions may leak out of the cells to the surrounding soil prior to REL assessments resulting in lower leakage values (Stattin & Lindström, Citation1999; Coursolle et al., Citation2000; Repo & Ryyppö, Citation2007). Therefore, the second approach is preferred (e.g. Wilner, Citation1961; McKay & Mason, Citation1991; McKay, Citation1994; Ryyppö et al., Citation1998; Sutinen et al., Citation1998). In the pre-treatment, the root system is washed out of the soil, rinsed with deionised water before inserting different root categories or a whole root system into test tubes for the freezing test (McKay, Citation1994; Bigras, Citation1997; McEvoy & McKay, Citation1997; Ryyppö et al., Citation1998; Sutinen et al., Citation1998). Since the impact of temperature on root follows S-curve, nonlinear regression analysis is an appropriate method for the estimation of frost hardiness. The most accurate estimate for frost hardiness may be obtained, if the test includes a series of test temperatures (6 – 7) covering the whole range from non-injurious to the lethal level (Ryyppö et al., Citation1998; Stattin & Lindström, Citation1999). Otherwise, even the sigmoid curve with the highest r-square may fail or may give a biased estimate for frost hardiness.
Theoretically, REL values after a freezing test would range from close to zero for healthy roots to almost 100% for intact and fully injured samples, respectively. However, the range is practically much narrower. Intact root samples may leak ions, depending on cell membrane integrity, when set in distilled/deionised water. The REL values of control samples may range from 20 – 60% () (Bigras, Citation1997; Ryyppö et al., Citation1998). The upper level may stay below 100% because all ions never leak out of the cells during the incubation period even though they have suffered lethal injuries by frost.
Figure 4. Root electrolyte leakage (REL) of fine roots (open circles) and woody roots (solid circles) of Scots pine seedlings after exposure to a series of declining temperatures in a phase during cold acclimation in hydroponics. Frost hardiness, defined as the inflection point of the sigmoid curves fitted to the data, was −4.1 and −3.8°C for fine and woody roots, respectively (Redrawn from Ryyppö et al., Citation1998).
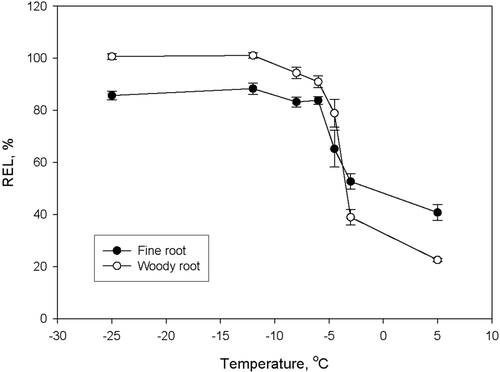
Frost hardiness of fine roots of Japanese larch (Larix leptolepis (Sieb. and Zucc.) Gord.) and hybrid larch (Larix × eurolepis Henry) were studied by means of REL technique throughout the over-wintering period in Britain (McKay & Morgan, Citation2001). In mid-winter, the temperature that caused 50% REL (not the inflection point) was −12°C for Japanese larch and −15°C for hybrid larch ().
Figure 5. Time course of temperature causing 50% electrolyte leakage of fine roots of Japanese larch (Larix leptolepis (Sieb. and Zucc.) Gord.) (•) and hybrid larch (Larix × eurolepis Henry) (▪) in 1990 – 91 (―) and 1991 – 92 (‐‐‐). (Adapted with permission from McKay & Morgan, Citation2001. © 2001 Elsevier).
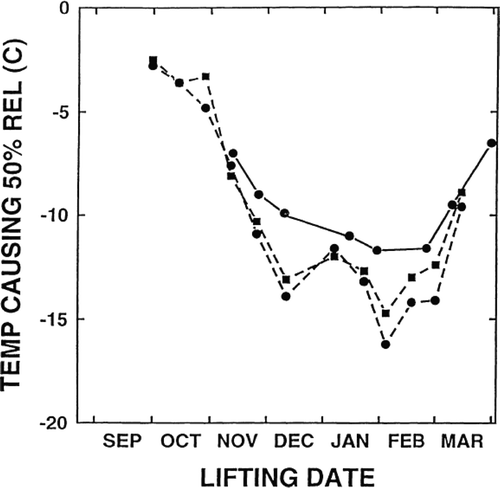
In a rapid test of frost hardiness, one or two freezing temperatures are used followed by REL test (Colombo et al., Citation1995; Stattin et al., Citation2000). Such a test does not result in an exact value of frost hardiness but only in an approximate one. For propose of monitoring, this approach may be valuable since it indicates whether a certain temperature threshold is exceeded or not. Such an application takes place for example in the seedling nurseries where knowledge of the effects of cold storage on the seedlings is needed (Stattin et al., Citation2000). A similar approach was used by Colombo et al. (Citation1995) for studying gradient of relative frost hardiness along the stem and roots of black spruce [Picea mariana (Mill.) B.S.P.] seedlings. A weakness of this approach is that seasonal variations at the basic level of REL may masque the results of the actual damage on the root cells (McKay, Citation1994; McKay & Milner, Citation2000).
In conclusion, REL technique can be used for frost hardiness assessment of roots with certain prerequisites: (i) a test should include a series of frost temperature with an unfrozen control; (ii) roots should be sampled into the test tubes prior to the freezing test to avoid loosing electrolytes into the substrate. A shortcoming in this operation is that the pre-treatment, i.e. washing of the soil from the root system and sample dissection may affect frost hardiness of roots. Additionally, sample freezing may cause different responses in the test tubes than in soil and thereby it may affect cellular injuries.
Heat stress
Most studies on heat stress tolerance of plants using REL are carried out on temperate and boreal regions. To the authors knowledge, no data is available for heat stress effects on REL in some Mediterranean and desert habitats, where surface soil temperatures may reach temperatures in excess of 60°C for several hours per day. The physiological basis of REL studies concerning heat tolerance of plant roots is the same as for studies of frost tolerance, i.e. cell membranes loose their ability to keep their normal selectivity when exposed to temperatures above their tolerance. Experimental procedure in the heat stress test follows the same general principles as given earlier.
The factors that affect plant resistance to high temperatures are the temperature level, the rate of temperature changes, and its duration (cf. freezing stress). When the roots of Douglas fir (Pseudotsuga menziesii (Mirb.) Franco), ash (Fraxinus excelsior L.), sessile oak [Quercus petraea (Matt.)], and sycamore (Acer pseudoplatanus L.) were exposed to high temperatures (45°C or 57°C), the damage, as assessed by using REL technique, increased proportionally to the exposure time () (Folk and Grossnickle, Citation1997, Citation1999; Mortazavi et al., Citation2004). Large differences were observed for different species which may be partly explained by duration of exposure and intensity. Exposure during a few minutes to 57°C was lethal for Douglas fir roots (Folk et al., Citation1999) but several hours at 45°C were required to get the same effect in ash, oak, and sycamore (Mortazavi et al., Citation2004). Survival of Douglas fir seedlings, in the re-growth test decreased when REL values exceeded 20%. None of the seedlings survived when REL values exceeded 30%. Similar to those after cold stress, leakage values following heat stress are affected by the physiological status of the roots too (Folk et al., Citation1999).
Figure 6. The relative electrolyte leakage for taproots of ash (Fraxinus excelsior L.), oak (Quercus petraea Liebl.), and sycamore (Acer pseudoplatanus L.) seedlings after exposure to 45°C for different durations (from 0 to 8 h) at different lifting dates during the annual cycle. (Adapted with permission from Mortazavi et al., Citation2004. © 2004 Springer).
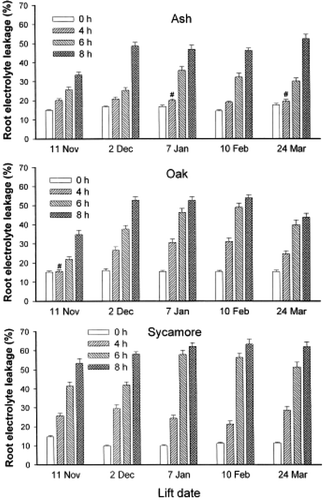
In Douglas fir, REL values between 15% and 25% were sometimes associated with root damage and other times were related to the health state of root systems (Folk et al., Citation1999). The variation in seedling quality and REL values seemed to depend on seed lot and/or on the annual phase of development of the examined plants. None of the experiments proved levelling of the time course of REL values as a result of exposure time. In Douglas fir, REL values of approximately 70% was reached after the longest exposure time (60 min at 57°C) and were associated with root damage (or reduced survival). On the contrary, in ash, oak, and sycamore the REL maxima ranged from 50 – 60% after 8 h of exposure at 45°C () (Mortazavi et al., Citation2004). Thus, REL is species-dependent and it varies with the annual phase of development.
In conclusion, REL can be used to study heat stress tolerance of roots provided that the following procedures are adhered to: (i) soil should be carefully removed from roots; (ii) both high temperature and its duration affect the root injuries, so they should be controlled in the tests; (iii) a series of high temperatures or different exposure times should be used to find a threshold for heat tolerance.
Desiccation/drought stress
Studies using REL to asses the effects of water deficiency in roots or their desiccation may be divided into three categories: (i) root responses to desiccation stress at high temperatures in controlled environment rooms; (ii) root responses to desiccation stress at low temperatures; (iii) root responses to drought during their growing period. The REL tests followed approximately the same schedule as presented above (with slight modifications from study to study), i.e. roots were sampled and inserted into test tubes, incubated in deionised/distilled water, heat-killed, and incubated for another period of time. Electrical conductivity was measured after the first and second incubation periods, and the REL was respectively calculated.
In the experiments with controlled desiccation treatments at 15 – 20°C, the roots were washed out of the soil and exposed to desiccation conditions for different times (from 0 – 24 h) in controlled environment rooms. Air temperature, air humidity, photon flux density, and air flow were controlled (McKay & White, Citation1997; McKay & Milner, Citation2000; McKay & Morgan, Citation2001; Radoglou & Raftoyannis, Citation2001, Citation2002; Brønnum, Citation2005a,Citationb). Root sampling for REL tests took place immediately after the treatment either without or with further rinsing of roots in deionised water. In all studies, REL increased with desiccation time (e.g. McKay & White, Citation1997; Radoglou & Raftoyannis, Citation2001; Brønnum, Citation2005b). When desiccation treatments were repeated throughout the winter, differences in REL between control and exposed seedlings were retained (McKay & Milner, Citation2000; McKay & Morgan, Citation2001). REL of control plants displayed a clear seasonal pattern whose magnitude was dependent on species. This was also reflected in REL after desiccation stress (McKay & Milner, Citation2000; McKay & Morgan, Citation2001). High variation was observed between species and seed lots as well, when REL was correlated with survivability in the re-growth test after the stress (McKay & White, Citation1997; Radoglou & Raftoyannis, Citation2002).
Desiccation of bare-root seedlings during cold storage (1°C or 4°C) for different times (from 2 – 12 weeks) increased REL values in red oak (Quercus rubra L.), pedunculate oak (Quercus robur L.), and radiata pine (Pinus radiata D. Don), but the values of REL declined in Corsican pine (Pinus nigra ssp laricio var Corsicana) (Garriou et al., Citation2000; Mena-Petite et al., Citation2001; Généré et al., Citation2004). The increase in red oak and pedunculate oak depended on the lifting date. In those desiccation studies, REL was closely related to field performance in oak and radiata pine but not in Corsican pine (Garriou et al., Citation2000; Mena-Petite et al., Citation2001).
When seedlings of pubescent oak (Quercus pubescens L.), flowering ash (Fraxinus ornus Willd.), and holm-oak (Quercus ilex) were exposed to drought stress for 50 – 100 days during growing season, REL changed little only or it was slightly reduced (Chiatante et al., Citation2005, Citation2006). This was contrary to the initial hypothesis, as other root measurements indicated a strong decline in root vitality. It is possible that in those studies, electrolytes leaked from damaged roots to the surrounding soil during exposure to drought stress or that some electrolytes were removed during washing.
Other stresses
Mechanical damage caused by rough handling, i.e. dropping to ground from different heights, of bare-roots and container seedlings caused elevated REL for fine roots, suggesting either that the trans-membrane electrolyte transport was impaired or that the cell membranes were ruptured by the treatment (McKay et al., Citation1993; McKay & Milner, Citation2000; McKay & Morgan, Citation2001). In container seedlings of Sitka spruce [Picea sitchensis (Bong.) Carr.], REL increased in proportion to the drop height being 13% and 17% for the control and 3 m of drop height, respectively (McKay et al., Citation1993). Rough handling of bare-root seedlings caused slight elevation of REL in Japanese larch [Larix kaempferi (Lamb.) Carr.] in hybrid larch (Larix x eurolepsis Henry) and in Sitka spruce (McKay & Milner, Citation2000; McKay & Morgan, Citation2001). The effect depended on the stage of development of the plants. It was strongest for the plants that were still growing or for those whose growth had just ceased before entering into dormancy. At that time, REL of Sitka spruce was approximately 30% for control and 70% for rough-treated seedlings (McKay & Milner, Citation2000). Dormant seedlings seemed to be insensitive to rough handling. Increased ion leakage of roots by rough handling was correlated with a delay in their terminal bud burst following spring.
Hypoxic conditions, e.g. during flooding, can induce stress responses in plants. When jack pine (Pinus banksiana Lamb.) seedlings were grown in a hypoxic solution culture for 4 weeks REL increased by 20% as compared with seedlings of a well-aerated conditions (Apostol & Zwiazek, Citation2003). The results suggested that under prolonged hypoxic conditions the sodium and chloride metabolism of those roots were affected and was expressed as damage on the root membranes (Apostol & Zwiazek, Citation2003).
Assessment of storability
Between the time of lifting and planting, seedlings may need to be stored for up to several months under cold or freezing temperatures or for a few weeks under cool or warm temperatures to facilitate nursery and planting operations. Deterioration in quality might occur during handling and storage before planting due to a variety of stresses experienced, perhaps including desiccation and/or depletion of food reserves (Mattson, Citation1997). Seedling's storability is dependent on the stage of physiological development and on the species as well. REL can be used as an indicator of storage readiness of susceptibility to deterioration during storage and of field performance. The effects of cold and ambient storage on REL of forest seedlings are reviewed below.
Cold and freezing storage
Cold (1 – 2°C) or freezing (−2°C to −4°C) storage of forest nursery stock is a common practice in temperate and boreal forest regions (Camm et al., Citation1994). Plants can be lifted from the nursery bed and stored for several months to facilitate lifting and planting operations (Camm et al., Citation1994). Despite the advantages of cold storage, incorrect storage practices, e.g. desiccating conditions, have been implicated in many cases for post-planting failures (McKay & Mason, Citation1991). However, desiccation stress can occur, regardless of package material used, if the plants are kept in storage for very long periods (Colombo, Citation1990; McKay, Citation1993).
Fine roots tend to be more sensitive to handling and storage stresses than the shoots. For this reason, the deterioration of fine root quality during storage is a major factor affecting seedling quality. REL has been widely used to detect root injury following cold storage in both conifer (e.g. McKay & Mason, Citation1991; Lindström & Mattsson, 1994; Stattin et al., Citation2000; Mena-Petite et al., Citation2001) and broad-leaved seedlings (Garriou et al., Citation2000; O'Reilly et al., Citation1999, Citation2002, Citation2003; Généré et al., Citation2004; Brønnum, Citation2005a). Electrolyte leakage from the fine roots of Sitka spruce, Douglas fir, and Japanese larch [Larix leptolepis (Sieb. and Zucc.) Gord.] cold-stored at 1°C for various durations was highly correlated to both survival and height growth for two growing seasons after planting in the field (McKay, Citation1992). Mena-Petite et al. (Citation2001) observed that REL increased steadily over a 15-day storage period at 4°C in pine seedlings (Pinus radiata D. Don) indicating an increase of cellular damage. Furthermore, REL was closely correlated with post-storage survival. In contrast, Généré and Garriou (Citation1999) found that REL was an unreliable predictor of field performance in Douglas fir seedlings following storage at 2°C. REL for fine roots was higher or about the same after cold storage as at the time of lifting in ash, sycamore, and sessile oak sampled from a bare-root nursery in Ireland (O'Reilly et al., Citation2003). While sycamore grew poorly after planting regardless of storage date, ash, and oak lifted to the cold storage from December to March performed well in the field. High REL values following storage were not associated with root deterioration and thus REL was not a reliable indicator of post-planting performance (O'Reilly et al., Citation2003). In conclusion, although REL may be useful in quantifying damage caused by cold storage, it should be considered only as a relative index of plant quality since the relationship between REL and survival could vary depending on cultural and handling practices prior to planting and post-planting environmental conditions (McKay, Citation1992; McKay & White, Citation1997).
Ambient storage (cool to warm temperatures)
Simulated ambient storage at 15°C for 21 days increased REL in pedunculate oak (Quercus robur L.) and Douglas fir seedlings, but the results were not consistent with post-planting performance (Harper & O'Reilly, Citation2000; Cabral & O'Reilly, Citation2005). When Douglas fir seedlings were placed in warm storage for 21 days REL increased from 47 – 63% for seedlings lifted in October and from 38 – 58% in December. However, REL was considered to be a poor indicator of survival potential survival (Harper & O'Reilly, Citation2000). In pedunculate oak, REL oscillated during warm storage (Cabral & O'Reilly, Citation2005). For example, although a 21-day warm storage period reduced plant quality in oak, REL actually declined during the final 7 – 10 days of storage was measured over the course of 21 days and REL values went up and down, i.e. oscillated during the warm storage period. REL might be expected to increase, when root vitality had been deteriorated. Thus, it may be concluded that REL was an unreliable indicator of seedling vitality in oak.
Both root and needle electrolyte leakages were accurate predictors of seedling vitality in radiata pine that had been stored at 10°C for up to 15 days (Mena-Petite et al., Citation2001). Shoot tip electrolyte leakage was an accurate predictor of survival and growth of white pine, red pine, white spruce, and black spruce seedlings following storage at 15°C and 30°C (Maki & Colombo, Citation2001). The authors concluded that this incipient injury (i.e. no visual foliar damage) can reduce seedlings vigor and therefore, may reduce performance potential.
REL as predictor of field performance
REL has been used to predict the field performance of tree seedlings. This was originally proposed by McKay (Citation1992). REL was measured in the spring before planting and after the seedlings had been cold-stored (1°C) for 1 – 7 months during the winter (McKay & Mason, Citation1991). Field survival and height increment of two-year-old Sitka spruce (Picea sitchensis (Bong.) Carr) and Douglas-fir (Pseudotsuga menziesii (Mirb.) Franco) seedlings were measured after a growing season. The highly significant correlation (up to r 2 = 0.90) between REL and both field survival and height growth was found. In another study, two-year-old Japanese larch [Larix leptolepis (Sieb. & Zucc.) Gord.] seedlings were tested after cold-stored at 1°C for 1 – 6 months (McKay, Citation1992). REL was measured at the end of the cold storage period from the fine roots. REL was closely correlated with both survival and height growth after two growing seasons in field. REL was found to be a better predictor of field performance than root growth potential too. Furthermore, REL was proven to be correlated with survival and growth of bare-rooted two-year-old Sitka spruce [Picea sitchensis (Bong.) Carr] and Japanese larch (Larix leptolepis (Sieb. & Zucc.) Gord.) seedlings (McKay, Citation1998b). REL from the fine roots of spruce was closely correlated to survival following direct planting at different times following cold storage (1°C).
In a study with Sitka spruce and Douglas fir seedlings, McKay & White (Citation1997) demonstrated that differences in site conditions strongly influence the survival and growth of seedlings. Therefore, site conditions can also influence the prediction ability of the REL test. REL was a poor indicator of field performance, when survival and growth of seedlings were averaged over all six sites. However, REL was found to be a useful indicator of field performance on sites with low spring rainfall. Field survival of Sitka spruce seedlings was negatively related to REL and it decreased more at high REL values compared to low REL values. Reduction in diameter and height growth of Sitka spruce seedlings was linear as compared with increase in REL. A linear negative relationship was found between the field survival of Douglas fir seedlings and REL but no clear relationship between REL and growth. However, later studies have demonstrated that there is inconsistency in predicting the field performance of seedlings with REL. Studies with Japanese larch and hybrid larch seedlings have shown that REL predicted the field survival to some extent but root growth potential was a better predictor (McKay & Morgan, Citation2001). Similar results were found with Austrian pine seedlings (Pinus nigra Arnold) (Chiatante et al., Citation2002). Bigras (Citation1997) has demonstrated with 6-month-old black spruce (Picea mariana) seedlings that REL differed among fine roots, coarse roots, and whole root systems. REL was correlated with percentage of live root mass in coarse roots and the whole root system but not in fine roots. Percentage of live root dry mass was, in turn, highly correlated with seedling survival and re-growth.
REL has been characterized as a poor predictor of seedling survival. Harper & O'Reilly (Citation2000) demonstrated this with Douglas-fir seedlings, which were lifted on four occasions from October to January and stored at 15°C for up to 21 days. The survival was tested after 0, 7, and 21 days warm storage and 8 weeks growth in the greenhouse environment. REL was found to increase after 7 days storage despite that root growth potential and survival were unaffected. REL slightly increased during the following days up to 21 days in warm storage, but values were almost the same as after 7 days warm storage. On the other hand, storage for up to 21 days greatly decreased root growth potential and survival. Studies with ash (Fraxinus excelsior L.) and sycamore (Acer pseudoplantanus L.) seedlings showed that REL could not predict their field height growth (O'Reilly et al., Citation2002). Seedlings from the same nursery in Ireland were tested at periodic intervals from September to May and also after cold storage at 1°C from the time of lifting until May. REL was very high already in September, but this did not result in poor field performance. The authors conclude that high REL values indicate high cellular activity and thus may not predict field performance.
In contrast to the above results, REL has been found to predict field survival of two-year-old bare root sycamore, one-year-old bare-root flowering ash (Fraxinux ornus L.), and one-year-old, container grown chestnut (Castanea sativa Mill.) seedlings (Radoglou & Raftoyannis, Citation2001). These seedlings were exposed to desiccation (15°C, 50% RH, air movement 0.3 ms−1 and PAR 350 μmol m−2 s−1) and freezing (−5°C) stress for 0, 3, 24, or 48 h. After the stress treatment, seedlings were planted in a field site. REL was found to correlate better to field survival than root moisture content or root water potential.
In summary, REL is in some cases correlated with field performance of seedlings, but in other cases the correlations are week. The factors as species, seed lots, developmental stage of root tissue, season, and bud dormancy intensity may affect REL. Thus, REL must first be calibrated to these factors before it can be reliably used to predict the field performance of all types of seedling stock.
What does ageing of a root imply? Is it an accumulation of damaged systems or is it an independent inherent process? Some roots live for a few weeks only, whereas others survive and function for years (Waisel et al., 2002). A method that reflects the status of the roots and is able to predict the beginning of the death processes should be applicable to both types of roots.
Amino acids and protein leakage
Root production and root decomposition constitute the basic process of the carbon flux in the soils of terrestrial ecosystems. If such a turnover of roots is to be estimated properly, one must be able to distinguish accurately between the living and dead roots. Usually this is done on the basis of the roots' color changes or of the disintegration state of their cortex. Nevertheless, the question remains open whether such criteria can really distinguish between live damaged roots and dead roots (cf. Polomski & Kuhn, Citation2002; Waisel et al., Citation2002). We may also ask whether REL alone may identify the state of approaching death of roots or one should measure leakage rates of amino acids and proteins too. One of the first features that characterize ageing of tissues, or of tissues that have suffered damage, is the loss of selectivity of their cell membranes. Consequently, the cells lose control on the trafficking of materials into and out of their organelles and cells. Under such circumstances, minerals, sugars, amino acids, and polypeptides leak out of the affected cells, i.e. the electrolyte as measured using REL are just a small part of the leakage (cf. Basu et al., Citation1994). In the following text, we will review the leakage of amino acids and proteins of roots as a result of various stresses and by ageing.
Effects of various stresses
Most studies on root leakage of amino acids and proteins have focused on responses to physical and chemical stresses, i.e. hypo-oxidation, high temperatures, salinity, or to bacterial infections. Unfortunately, the effects of mycorrhizal infections were practically untouched. For example, (i) short periods of hypo-oxidation caused damage in root cell membranes of barley (Hordeum vulgare L.) which was observed as leakage of organic and amino acids within one hour (Hiatt & Lowe, Citation1967). (ii) Raising the ambient temperature of lettuce or of Barbarea roots, from 25 – 40°C, caused an increase in the plasma membrane leakage of amino acids. It was speculated that the change in temperature caused a transient change in membrane structure with a subsequent increase in leakage (Wood & Paleg, Citation1974; Hendricks & Taylorson, Citation1976, Citation1979). (iii) Soybean (Glycine max) roots exuded a 28-kDa peptide after exposure to water stress (Bozarth et al., Citation1987). Those investigators suggested that the exuded peptide was associated with cell wall growth, having either a structural role or a catalytic function. When growth had stopped or had changed in rate this protein was lost to the environment. (iv) Roots (or cell cultures) of Zea mays L. exuded various macromolecules, including proteins and pectins, following ionic stress. Apparently, this was interrelated with exposure to aluminum stress and was related to the aluminum resistance of the tested cultivars (Schmohl & Horst, Citation2002). (v) Lycopersicum spp. roots exuded six different polypeptides when plants were starved of phosphorous (Goldstein et al., Citation1989). (vi) Roots of Nicotiana tabacum exuded three polypeptides (11, 29, and 40 kDa) when the plants were subjected to salt stress (Iraki et al., Citation1989). (vii) Amino acids and protein leakage out of roots were also connected with the interrelations with the surrounding microflora. Amino acid loss from Brassica rapa L. roots differed for sterile roots from that of non-sterile ones. It was concluded that leakage of amino acids and of polypeptides supported the subsistence of bacteria and their activity in the rhizosphere. Exudation of isoflavonoids by roots of Medicago sativa L. was demonstrated following inoculation with Rhizobium. (viii) Lycopersicum spp. roots' exudation of amino acids differed in quantity and composition with the growth phase of the plants (Vancura & Hovadik, Citation1965).
When the roots of Ficus spp were exposed to desiccation stress, for 1, 2, or 4 h on the lab bench, their amino acid and polypeptide leakage increased (). Leakage correlated with the weight loss during desiccation treatment (). However, leakage rates differed depending on root age and species, e.g. Phoenix dactylifera, Ficus rubiginosa, Tamarix aphylla var erectus or Acacia. Leakage of young-white roots was relatively low, probably because of the high stability of their membranes. Roots showed enhanced leakage of ninhydrin-reacting substances as they became older, dark-colored, and probably less active too (). Relatively little leakage was observed from dark-brown roots, though more roots of this category were dead.
Figure 7. Leakage of amino acids (determined by the ninhydrin reaction) from ficus (Ficus rubiginosa) aerial roots following dehydration treatment.
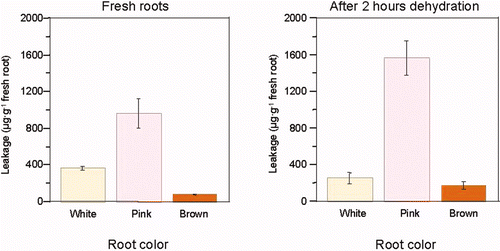
Figure 8. Leakage of amino acids (determined by the ninhydrin reaction) from ficus (Ficus rubiginosa) aerial roots following dehydration treatment.
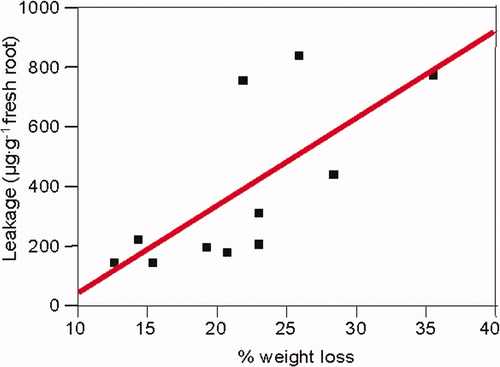
Leakage of amino acids and/or polypeptides out of roots indicates an incipient damage. Such a state may indicate a declined physiological status or the approaching death of roots. If the leakage would correlate with some visual features of roots, e.g. color or appearance of wrinkles on the root surface, the method would be useful for the identification of dying roots in minirhizotron observations too.
Effects of ageing
Waisel & Samocha (Citation2006) reported that leakage of amino acids and of polypeptides would signal the beginning of the dying process in roots. In their study, white to dark brown roots and 3 – 4 cm long root tips of banana (Musa cavendishii), date palm (Phoenix dactylifera), ficus (Ficus rubiginosa), tamarix (Tamarix aphylla var erectus), and poplar (Populus alba) were excised from plants grown in the Sarah Racine Aeroponic Root Laboratory, Tel Aviv, Israel. In addition, aerial roots of ficus were taken from trees of the university garden. According to their results, ninhydrin reaction is apparently not the best test for identification of dead roots because water soluble substances have evidently leached out of roots by rain or irrigation water. Therefore, any subsequent leaching from such roots is rather minor. On the other hand, leakage of ninhydrin-reacting substances from “dying roots”, i.e. roots at the transition state between life and death, was relatively high. At that stage the root cell membranes lose their semi-permeability, and the synplastic organic compounds may leak out of the cells. As all root cells are not damaged at the same time and death of the cortex and bark ensues before the damage in the inner cylinder, we may conclude that the process of “root death” takes place gradually.
To conclude, the leakage pattern of amino acids and proteins were observed to vary between species (Waisel & Samocha, Citation2006). Thus, one should test the leakage behavior of the roots of each species individually, before applying the ninhydrin method as a general diagnostic tool to evaluate the physiological state of roots. It is not known at the tissue and cellular level where the various substances leak out (cf. Van Egeraat, Citation1975). To our best knowledge, all cells are not responding simultaneously and there might be differences in the leakage between cell membranes. Further investigations should be carried out about the relation of amino acids and proteins leakage with the REL value of whole roots.
Conclusions
REL test is a quick, inexpensive, and simple method for assessing physiological status of sample fractions of root system. Results are quantitative, allowing probability values to be assigned to results, and are generally obtained within 48 h. Additionally, this method causes only little damage and, thus, enables the tested plants to be planted in the field after processing. In particular, REL may be useful in quantifying damage caused by cold storage and freezing temperatures. However, single measurements of REL may not predict root vitality and root membrane damage accurately because REL values may vary due to factors other than damage. REL is a dynamic variable and varies seasonally in seedlings regardless of root injury. Therefore, the interpretation of REL must take into account the physiological condition of seedlings as well as the potential for genetic variation between seed sources and nursery practices. Increase in REL is related to decreases in seedling survival and performance potential, but this relation is not universal for all species. REL should be considered as a relative index of plant quality only since the relation between REL and survival varies with cultural and handling practices prior to planting and post-planting environmental conditions. The method can be a useful indicator of seedling damage provided that acceptable thresholds of survival probability will be established and adequately correlated with concrete REL values. It is recommended that REL be used in conjunction with other measures of seedling physiological determinants.
To improve the method and decrease variation, attention should be paid to preserve root membrane integrity. The use of distilled water for such a test may cause by itself membrane damage (Epstein, Citation1961; Waisel, Citation1962; Poovaiah & Reddy, Citation1987). This may be avoided by incubation of root samples, instead of distilled or deionized water, in a solution of low Ca2+ concentration, at a predetermined pH and with an adequate aeration. Further studies are needed to compare the REL values of sampled fractions with those of the whole root system.
Acknowledgements
We specially thank Dr C. O'Reilly for his data contribution.
References
- Apostol , K G and Zwiazek , J J . 2003 . Hypoxia affects root sodium and chloride concentrations and alters water conductance in salt-treated jack pine (Pinus banksiana) seedlings . Trees , 17 : 251 – 257 .
- Basu , U , Basu , A and Taylor , G J . 1994 . Differential exudation of polypeptides by roots of Al-resistant and Al-sensitive cultivars of Triticum aestivum L. in response to aluminium stress . Plant Physiol , 106 : 151 – 158 .
- Bigras , F J . 1997 . Root cold tolerance of black spruce seedlings: Viability tests in relation to survival and regrowth . Tree Physiol , 17 : 311 – 318 .
- Birgas , F J and Calme , S . 1994 . Vitality tests for estimating root cold tolerance of black spruce seedlings . Can J Forest Res , 24 : 1039 – 1048 .
- Birgas , F J and D'Aoust , A L . 1992 . Hardening and dehardening of shoots and roots of containerized black spruce and white spruce seedlings under short and long days . Can J Forest Res , 22 : 388 – 396 .
- Bozarth , C S , Mullet , J E and Boyer , J S . 1987 . Cell wall proteins at low water potentials . Plant Physiol , 85 : 261 – 267 .
- Bronnum , P . 2005a . Assessment of seedlings storability of Quercus robur and Pinus sylvestris . Scand J Forest Res , 20 : 26 – 35 .
- Bronnum , P . 2005b . Preplanning indicators of survival and growth of desiccated Abies procera bareroot planting stock . Scand J Forest Res , 20 : 36 – 46 .
- Burr , K E . The target seedlings concepts: Bud dormancy and cold hardiness . The Target Seedling Symposium: Combined Meeting of the Western Forest Nursery Associations . Roseburg, Oregon. Edited by: Rose , R , Campbell , S J and Landis , T D . pp. 79 – 90 . US Department Agriculture Forest Service General Technical Report RM-200 .
- Burr , K E , Tinus , R W , Walner , S J and King , R M . 1990 . Comparison of three cold hardiness tests for conifers . Tree Physiol , 6 : 351 – 369 .
- Cabral , R and O'Reilly , C . 2005 . The physiological responses of oak seedlings to warm storage . Can J Forest Res , 35 : 2413 – 2422 .
- Camm , E L , Goetze , D C , Silim , S N and Lavender , D P . 1994 . Cold storage of conifer seedlings: An update from the British Columbia perspective . Forest Chron , 70 : 311 – 316 .
- Colombo , S J . 1990 . Bud dormancy status, frost hardiness, shoot moisture content and readiness of black spruce container seedlings for frozen storage . J Am Soc Hort Sci , 115 : 302 – 307 .
- Colombo , S J . 1994 . Timing of cold temperature exposure effects root and shot hardiness of Picea mariana container seedling . Scand J Forest Res , 9 : 52 – 59 .
- Colombo , S J , Zhao , S and Blumwald , E . 1995 . Frost hardiness gradients in shoots and roots of Picea mariana seedlings . Scand J Forest Res , 10 : 32 – 36 .
- Chiatante , D , Di Iorio , A , Sciandra , S , Scippa , G S and Mazzoleni , S . 2006 . Effects of drought and fine root development in Quercus pubescens Willd. and Fraxinus ornus seedlings . Env Exp Bot , 56 : 190 – 197 .
- Chiatante , D , Di Iorio , A , Scippa , G S and Sarnataro , M . 2002 . Improving vigor assessment of pine (Pinus nigra Arnold) . Plant Biosystem , 136 : 209 – 219 .
- Chiatante , D , Di Iorio , A and Scippa , G S . 2005 . Root response of Quercus ilex L. seedlings to drought and fire . Plant Biosyst , 139 : 198 – 208 .
- Coursolle , C , Bigras , F and Margolis , H A . 2000 . Assessment of root freezing damage of two years old white spruce, black spruce, and jack pine seedlings . Scan J Forest Res , 15 : 343 – 353 .
- Dexter , S T , Tottingham , W E and Graber , L F . 1930 . Preliminary results in measuring the hardiness of plants . Plant Physiol , 5 : 215 – 223 .
- Dexter , S T , Tottingham , W E and Graber , L F . 1932 . Investigations of the hardiness of plants by measurement of electrical conductivity . Plant Physiol , 7 : 63 – 78 .
- Duryea , M L . 1985 . “ Evaluation seedling quality importance to reforestation ” . In Evaluation seedlings quality: Principles, procedures and predictive abilities of major tests , Edited by: Duryea , M L . 1 – 6 . Corvallis, OR : Forest Research Laboratory, Oregon State University .
- Epron , D and Dreyer , E . 1992 . Effects of severe dehydration on leaf photosynthesis in Quercus pertraea (Matt.) Liebl: Photosystem II efficiency, photochemical and nonphotochemical fluorescence quenching and electrolyte leakage . Tree Physiol , 10 : 273 – 284 .
- Epstein , E . 1961 . The essential role of calcium in selective cation transport by plant cells . Plant Physiol , 36 : 437 – 444 .
- Flint , H L , Boyce , B R and Beattie , D J . 1967 . Index of injury – A useful expression on freezing injury to plant tissues as determined by the electrolytic method . Can J Plant Sci , 47 : 229 – 230 .
- Fitter , A H . 1991 . “ Characteristics and functions of root systems ” . In Plant roots: The hidden half , Edited by: Waisel , Y , Eshel , A and Kafkafi , U . 3 – 25 . New York : Marcel Dekker .
- Folk , R S and Grossnickle , S C . 1997 . Determining field performance potential with the use of limiting environmental conditions . New Forest , 13 : 121 – 138 .
- Folk , R S , Grossnickle , S C , Axelrood , P and Trotter , D . 1999 . Seed lot, nursery, and bud dormancy effects on root electrolyte of Douglas-fir (Pseudotsuga menziesii) seedlings . Can J Forest Res , 29 : 1269 – 1281 .
- Garriou , D , Girard , S , Guehl , J-M and Généré , B . 2000 . Effect of desiccation during cold storage on planting stock quality and field performance in forest species . Ann Forest Sci , 57 : 101 – 111 .
- Généré , B and Garriou , D . 1999 . Stock quality and field performance of Douglas-fir seedlings under varying degrees of water stress . Ann Forest Sci , 56 : 501 – 510 .
- Généré , B , Garriou , D , Omarzad , O , Grivet , J P and Hagège , D . 2004 . Effect of strong cold storage induced desiccation on metabolic solutes, stock quality and regrowth, in seedlings of two oak species . Trees , 18 : 559 – 565 .
- Goldstein , A H , Mayfield , S P , Danon , A and Tibbot , B K . 1989 . Phosphate starvation inducible metabolism in Lycopersicon esculentum . Plant Physiol , 91 : 175 – 182 .
- Grossnickle , S C and Folk , R S . 1993 . Stock quality assessment forecasting survival on performance on a reforestation site . Tree Planters Notes , 44 : 113 – 121 .
- Harper , C P and O'Reilly , C . 2000 . Effect of warm storage and date of lifting on the quality of Douglas-fir seedlings . New Forest , 20 : 1 – 13 .
- Hendricks , S B and Taylorson , R B . 1976 . Variation in germination and amino acid leakage of seeds with temperature related membrane phase change . Plant Physiol , 58 : 7 – 11 .
- Hendricks , S B and Taylorson , R B . 1979 . Dependence of thermal responses of seeds on membrane transitions . Proc Natl Acad Sci USA , 76 : 778 – 781 .
- Hiatt , A J and Lowe , R H . 1967 . Loss of inorganic acids, amino acids, K and Cl from barley roots treated anaerobically and with metabolic inhibitors . Plant Physiol , 42 : 1731 – 1736 .
- Iraki , N M , Bressan , R A and Capita , N C . 1989 . Extracellular polysaccharides and proteins of tobacco cell cultures and changes in composition associated with growth-limiting adaptation to water and saline stress . Plant Physiol , 91 : 54 – 61 .
- Kocheva , K , Georgiev , G and Kochev , V . 2005 . A diffusion approach to the electrolyte leakage from plant tissues . Physiol Plant , 125 : 1 – 9 .
- Larcher , W . 1995 . Physiological plant ecology , 1 – 560 . Berlin, Heidelberg : Springer .
- Lavender , D P . 1984 . “ Plant physiology and the nursery environment: interactions affecting seedling growth ” . In Forest nursery manual: Production of bare root seedlings , Edited by: Duryea , M L and Landis , T D . 133 – 141 . Hague : Martinus Nijhoff/Dr. W. Junk .
- Levitt , J . 1980 . Responses of plants to environmental stresses, Vol. I. Chilling, freezing and high temperature stresses , London : Academic Press .
- Lindstrom , A and Mattsson , A . 1989 . Equipment for freezing rots and its use to test cold resistance of young and mature roots of Norway spruce seedlings . Scand J Forest Res , 4 : 59 – 66 .
- Lindstrom , A and Nyström , C . 1987 . Seasonal variation in root hardiness of container-grown Scots pine, Norway spruce and lodgepole pine seedlings . Can J Forest Res , 17 : 787 – 793 .
- Maki , D and Colombo , S . 2001 . Early detection of the effects of warm storage on conifers seedlings using physiological tests . Forest Ecol Manag , 154 : 237 – 249 .
- Mason , W L and McKay , H . 1990 . Evaluating the quality of Sitka spruce planting stock before and after cold storage . Comb Proc Int Plant Prop Soc , 39 : 234 – 242 .
- Mattsson , A . 1997 . Predicting field performance using seedling quality assessment . New Forest , 13 : 227 – 252 .
- McEvoy , C and McKay , H . 1997 . Root frost hardiness of amenity broadleaved seedlings . Arboricult J , 21 : 231 – 244 .
- McKay , H M . 1992 . Electrolyte leakage from fine roots of conifer seedlings: A rapid index of plant vitality following cold storage . Can J Forest Res , 22 : 1371 – 1377 .
- McKay , H M . 1993 . Tolerance of conifer fine roots to cold storage . Can J Forest Res , 23 : 337 – 342 .
- McKay , H M . 1994 . Frost hardiness and cold-storage tolerance of the root system of Picea sitchensis, Pseudotsuga menziesii, Larix kaempferi and Pinus sylvestris bare-root seedlings . Scand J Forest Res , 9 : 203 – 213 .
- McKay , H M . 1997 . A review of the effects of stresses between lifting and planting on nursery stock quality and performance . New Forest , 13 : 369 – 399 .
- McKay , H M . 1998a . Optimal planting times for freshly lifted bare-rooted conifers on the North York Moors . Forestry , 71 : 33 – 48 .
- McKay , H M . 1998b . Root electrolyte leakage and root growth potential as indicators of spruce and larch establishment . Silva Fenn , 32 : 241 – 252 .
- McKay , H M , Gardiner , B A , Mason , W L , Nelson , B G and Hollingsworth , M K . 1993 . The gravitational forces generated by dropping plants and the response of Sitka spruce seedlings to dropping . Can J Forest Res , 23 : 2443 – 2451 .
- McKay , H M , Jinks , R L and McEvoy , C . 1999 . The effect of desiccation and rough handling on the survival and early growth of ash, beech, birch and oak seedlings . Ann Forest Sci , 56 : 391 – 402 .
- McKay , H M and Mason , W L . 1991 . Physiological indicators of tolerance to cold storage in Sitka spruce and Douglas-fir seedlings . Can J Forest Res , 21 : 890 – 901 .
- McKay , H M and Milner , A D . 2000 . Species and seasonal variability in the sensitivity conifer roots to drying and rough handling . Forestry , 73 : 259 – 270 .
- McKay , H M and Morgan , J L . 2001 . The physiological basis for the establishment of are-root larch seedlings . Forest Ecol Manag , 142 : 1 – 18 .
- McKay , H M and White , I MS . 1996 . Fine root electrolyte leakage and moisture content: Indices of Sitka spruce and Douglas-fir seedling performance after desiccation . New Forest , 13 : 139 – 162 .
- McKay , H M and White , I MS . 1997 . Fine root electrolyte leakage and moisture content: Indices of Sitka spruce and Douglas-fir seedling performance after desiccation . New Forest , 13 : 139 – 162 .
- Mena-Pelite , A , Ortega-Lasuen , U , Gonzalez-Moro , M B , Lacuesta , M and Munoz-Rueda , A . 2001 . Storage duration and temperature effect on the functional integrity of container and bare-root Pinus radiata D. Don stock-types . Trees , 15 : 289 – 296 .
- Mena-Petite , A , Robredo , A , Alcalde , S , Dunabeitia , M K , Gonzalez-Moro , M B , Lacuesta , M and Munoz-Rueda , A . 2003 . Gas exchange and chlorophyll fluorescence responses of Pinus radiata D. Don seedlings during and after several storage regimes and their effects on post-planting survival . Trees , 17 : 133 – 143 .
- Mohammed , G H . 1997 . The status and future of stock quality testing . New Forest , 13 : 491 – 514 .
- Monaghan , S . 2002 . Propagation, cold storage and mycorrhizal associations of nursery grown Douglas fir [Doctoral thesis] , Ireland : University College Dublin .
- Mortazavi , M , O'Reilly , C and Keane , M . 2004 . Stress resistance levels change little during dormancy in ash, sessile oak and sycamore seedlings . New Forest , 28 : 89 – 108 .
- Murray , M B , Cape , J N and Flower , D . 1989 . Quantification of frost damage in plant tissues by rates of electrolyte leakage . New Phytol , 113 : 307 – 311 .
- O'Reilly , C , Harper , C and Keane , K . 1999 . Influence of physiological status at time of lifting on cold storage tolerance and field performance of Douglas-fir and Sitka spruce . Irish Forest , 56 : 2 – 17 .
- O'Reilly , C , Harper , C and Keane , M . 2002 . Influence of physiological condition at the time of lifting on the cold storage tolerance and field performance of ash and sycamore . Forestry , 75 : 1 – 12 .
- O'Reilly , C , Harper , C , McCarthy , N and Keane , M . 2001 . Seasonal changes in physiological status, cold storage tolerance and field performance of hybrid larch seedlings in Ireland . Forestry , 74 : 407 – 421 .
- O'Reilly , C , McCarthy , N , Keane , M and Harper , C P . 2000 . Proposed dates for lifting Sitka spruce planting stock for fresh or cold storage, based on physiological indicators . New Forest , 19 : 117 – 141 .
- O'Reilly , C , Mortazavi , M and Keane , N . 2003 . Effects of physiological status on the cold storage tolerance and field performance of ash, oak and sycamore in Ireland . Phyton Annales Rei Botanicae , 43 : 335 – 350 .
- Palta , J P , Levitt , J and Stadelmann , E I . 1977 . Freezing injury in onion bulb cells. I. Evaluation of the conductivity method analysis of ion and sugar efflux from injured cells . Plant Physiol , 60 : 393 – 397 .
- Perks , P M , Monaghan , S , O'Reilly , C , Osborne , A B and Mitchell , T D . 2001 . Chlorophyll fluorescence characteristics, performance and survival of freshly lifted and cold stored Douglas fir seedlings . Ann Forest Sci , 58 : 225 – 235 .
- Puttonen , P . 1989 . Criteria for using seedling performance potential tests . New Forest , 3 : 67 – 87 .
- Puttonen , P . 1997 . Looking for the “silver bullet” can one test do it all? . New Forest , 13 : 9 – 27 .
- Polomski , J and Kuhn , N . 2002 . “ Root research methods ” . In Plant roots: The hidden half , 3rd ed , Edited by: Waisel , Y , Eshel , A and Kafkafi , U . 295 – 321 . New York : Marcel Dekker .
- Poovaiah , B W and Reddy , A SN . 1987 . Calcium messenger system in plants . CRC Crit Rev Sci , 6 : 47 – 103 .
- Radoglou , K and Raftoyannis , Y . 2001 . Effects of desiccation and freezing on vitality and field performance of broadleaved tree species . Ann Forest Sci , 58 : 59 – 68 .
- Radoglou , K and Raftoyannis , Y . 2002 . The impact of storage, desiccation and planting date on seedling quality and survival of woody plant species . Forestry , 75 : 179 – 190 .
- Radoglou , K , Raftoyannis , Y and Halivopoulos , G . 2003 . The effect of planting date and seedlings quality on field performance of Castanea sativa Mill and Quercus frainetto Ten . Forestry , 76 : 569 – 578 .
- Ritchie , G A . 1984 . “ Assessing seedling quality ” . In Forest nursery manual: Production of bare root seedlings , Edited by: Duryea , M L and Landis , T D . 243 – 259 . Corvallis, OR : Forest Research Laboratory, Oregon State University .
- Ritchie , G A and Landis , T D . 2006 . Seedling quality tests: Root electrolyte leakage. Forest Nursery Notes, USDA . Winter , 2006 : 6 – 10 .
- Ryyppö , A and Repo , T . 2007 . A limitation in electrolyte leakage method for cold hardiness measurement of roots Submitted manuscript
- Ryyppö , A , Repo , T and Vapaavuori , E . 1998 . Development of frost hardiness in roots and shoots of Scots pine seedlings at non-freezing temperatures . Can J Forest Res , 28 : 557 – 565 .
- Sakai , A and Larcher , W . 1987 . Frost survival of plants , 321 Berlin : Springer Verlag .
- Sampson , P H , Templeton , C WG and Colombo , S J . 1997 . An overview of Ontario stock quality assessment program . New Forest , 13 : 469 – 487 .
- Sarvas , M . 2003 . Change of cold hardiness in bare rooted Norway spruce planting stock during autumn and its effects on survival . J Forest Sci , 49 : 133 – 139 .
- Sarvas , M . 2004 . Change in cold hardiness of silver larch in bare rooted seedlings during autumn and spring . J Forest Sci , 50 : 237 – 242 .
- Schaberg , P G , Hennon , P E , DrAmore , D V , Hawley , G J and Borer , C H . 2005 . Seasonal differences in freezing tolerance of yellow-cedar and western hemlock trees at a site affected by yellow-cedar decline . Can J Forest Res , 35 : 2065 – 2070 .
- Schmohl , N and Horst , W . 2002 . Effect of aluminium on the activity of apoplastic acid phosphatase and exudation of macromolecules by roots and suspension-culture cells of Zea mays L . J Plant Physiol , 159 : 1213 – 1218 .
- Shalata , A and Neumann , P M . 2001 . Exogenous ascorbic acid (vitamin C) increases resistance to salt stress and reduces lipid peroxidation . J Exp Bot , 52 : 2207 – 2211 .
- Stattin , E and Lindstrom , A . 1999 . Influence of soil temperature on root freezing tolerance of Scots pine (Pinus sylvestris L.) seedlings . Plant Soil , 217 : 173 – 181 .
- Stattin , E , Hellqvist , C and Lindström , A . 2000 . Storability and root freezing tolerance of Norway spruce (Picea abies) seedlings . Can J Forest Res , 30 : 964 – 970 .
- Steponkus , P L . 1984 . Role of the plasmamembrance in freezing injury and cold acclimaion . Ann Rev Pl Physiol , 35 : 543 – 584 .
- Sutinen , M-L , Ritari , A , Holoppa , T and Kujala , K . 1998 . Seasonal changes in soil temperature and on the frost hardiness of Scots pine (Pinus sylvestris) roots under subarctic conditions . Can J Forest Res , 28 : 946 – 950 .
- Sutton , R F . 1979 . Planting stock quality and grading . Forest Ecol Manag , 2 : 123 – 132 .
- Tanaka , Y , Brotherton , P , Hostetter , S , Chapman , D , Dyce , S , Belanger , J , Johnson , B and Duke , S . 1997 . The operational planting stock quality testing program at Weyerhaeuser . New Forest , 13 : 423 – 437 .
- Vancura , V and Hovadik , A . 1965 . Roots exudates of plants. II. Composition of root exudates of some vegetables . Plant Soil , 22 : 21 – 32 .
- Van den Driessche , R . 1969 . Measurement of frost hardiness in two-year-old Douglas fir seedlings . Can J Plant Sci , 49 : 159 – 172 .
- Van Egeraat , A WSM . 1975 . Exudation of ninhydrin-positive compounds by pea seedlings: A study of the sites of exudation and of the composition of the exudates . Plant Soil , 42 : 37 – 47 .
- Vogt , K and Bloomfield , J . 1991 . “ Tree root turnover and senecence ” . In Plant roots: The hidden half , Edited by: Waisel , Y , Eshel , A and Kafkafi , U . 287 – 306 . New York : Marcel Dekker .
- Waisel , Y . 1962 . The effects of calcium on the uptake of monovalent ions by excised barley roots . Physiol Plant , 15 : 709 – 724 .
- Waisel , Y , Eshel , A and Kafkafi , U . 2002 . Plant roots: The hidden half , 3rd ed , New York : Marcel Dekker .
- Waisel , Y and Samocha , Y . How to identify a dying root? . Proceedings of Roots, Mycorrhizas and their External Mycelia in Carbon dynamics in Forest Soil, COST 38 . September 2006 , Rovaniemi, Finland. Poster No. 38
- Wilner , J . 1955 . Results of laboratory tests for winter hardiness of woody plant by electrolyte methods . Proc Am Hort Sci , 66 : 93 – 99 .
- Wilner , J . 1959 . Note on an electrolytic procedure for differentiating between frost injury of roots and shoots in woody plants . Can J Plant Sci , 39 : 512 – 513 .
- Wilner , J . 1960 . Relative and absolute electrical conductance tests for frost hardiness of apple varieties . Can J Plant Sci , 40 : 630 – 637 .
- Wilner , J . 1961 . Relationship between certain methods and procedures of testing for winter injury of outdoor exposed shoots and roots of apple trees . Can J Plant Sci , 41 : 309 – 315 .
- Wilson , B C and Jacobs , D F . Electrolyte leakage from stem tissue as an indicator of hardwood seedling physiological status and hardiness . Proceedings of the 14th Central Hardwoods Forest Conference . 2006 .
- Wood , A and Paleg , L G . 1974 . Alteration of liposomal memrane fluidity by gibberellic acid . Aust J Plant Physiol , 1 : 31 – 40 .
- Zhoa , S , Colombo , S J and Brumwald , E . 1995 . The induction of freezing tolerance in jack pine seedling: The role of root plasma membrane H+ ATPase and redox activities . Physiol Plant , 93 : 55 – 60 .