Abstract
Fungi produce various secondary metabolites that have beneficial and harmful effects on other organisms. Those bioactive metabolites have been explored as potential medicinal and antimicrobial resources. However, the activities of the culture filtrate (CF) and metabolites of white-rot fungus (Schizophyllum commune) have been underexplored. In this study, we assayed the antimicrobial activities of CF obtained from white-rot fungus against various plant pathogens and evaluated its efficacy for controlling anthracnose and gray mold in pepper plants. The CF inhibited the mycelial growth of various fungal plant pathogens, but not of bacterial pathogens. Diluted concentrations of CF significantly suppressed the severity of anthracnose and gray mold in pepper fruits. Furthermore, the incidence of anthracnose in field conditions was reduced by treatment with a 12.5% dilution of CF. The active compound responsible for the antifungal and disease control activity was identified and verified as schizostatin. Our results indicate that the CF of white-rot fungus can be used as an eco-friendly natural product against fungal plant pathogens. Moreover, the compound, schizostatin could be used as a biochemical resource or precursor for development as a pesticide. To the best of our knowledge, this is the first report on the control of plant diseases using CF and active compound from white-rot fungus. We discussed the controversial antagonistic activity of schizostatin and believe that the CF of white-rot fungus or its active compound, schizostatin, could be used as a biochemical pesticide against fungal diseases such as anthracnose and gray mold in many vegetables.
1. Introduction
Chili pepper (Capsicum annum L.) is one of the most important vegetable crops cultivated worldwide [Citation1]. It is commonly used for seasoning in many Asian countries including Korea. Diseases are serious threats in the cultivation of pepper plants and production of pepper. Therefore, many chemicals are used for the control of the diseases [Citation2]. Pathogens in the genera Alternaria, Botrytis, Cochliobolus, Fusarium, Geotrichum, Penicillium, Sclerotina, and Phytophthora are major concerns for commercial production of pepper. Among the diseases, anthracnose and gray mold caused by Colletotrichum gloeosporioides and Botrytis cinerea, respectively are the most economically important; they are responsible for both pre- and postharvest crop losses [Citation1–4], and consequently affect both qualitative and quantitative production.
Because of the threat of anthracnose in pepper cultivation, around 17 fungicides have been registered in South Korea for commercial application to pepper plants for the control of anthracnose in the field [Citation3]. However, those chemical fungicides are highly toxic to animals and humans. In addition, the heavy and widespread application of those fungicides has not only resulted in the development of pathogen resistant to the chemicals, but also become a major source of environmental contamination and ecosystem damage [Citation5]. Thus, it is necessary to develop alternative control strategies or eco-friendly chemicals to control plant diseases in an effective and sustainable way.
Biological control of plant diseases through the application of microorganisms is a potent alternative to harmful chemicals. Recent studies have shown that higher fungi (Ascomycota and Basidiomycota) could be natural sources for the production of fungicidal, herbicidal, and insecticidal compounds [Citation6]. Furthermore, Ascomycetes has been the source of many fungal-derived pharmaceuticals [Citation7]. Basidiomycota have also been rich sources of metabolites that inhibit the growth of bacteria, fungi, viruses, nematodes, and insects; more recently, many species in Basidiomycetes have been reported as potential sources for plant disease control [Citation8–10]. The Agaricomycetes class of Basidiomycota includes many species that offer biocontrol of fungal plant diseases. For example, antifungal compounds such as grifoline from Albatrellus dispansus, phellinsin A from Phellinus sp., and strobilurins from Strobilurus tenacellus have gained economic importance as alternatives to chemical fungicides.
S. commune is a widely distributed fungus that grows abundantly during the rainy season and is frequently found on decaying organic matter, especially the dead wood of trees [Citation11]. The fungus belongs to the genus Schizophyllum of the Schizophyllaceae family in the Agaricales order of the Agaricomycetes class. Although the fruiting body (mushroom) of the species is listed as inedible in European and North American guidebooks, it has been widely consumed as food and medicine in Africa and Asia [Citation12,Citation13]. The mushroom has been shown to have various biological functions, such as antitumoral, antioxidant, antimicrobial, anticancerous, and antineurasthenia effects [Citation14–16].
In spite of the various bioactive substances identified from the mycelial culture and mushrooms of S. commune, the use of antimicrobial properties of the metabolites as natural biochemical pesticides has been underexplored. In this study, to search eco-friendly alternatives for chemical pesticides, we evaluated the potential of extracts from a culture of the white-rot fungus S. commune to control plant diseases. We analyzed the antagonistic activity of cell-free culture filtrate (CF) from S. commune against fungal plant pathogens including C. gloeosporioides and B. cinerea. In addition, based on the selected treatment concentration and time, we tested the CF’s control efficacy against anthracnose in field conditions and as a postharvest protection for fruits. Furthermore, we identified and assayed the antimicrobial activity of the underlying bioactive compound. The CF of S. commune suppressed the mycelial growth of fungal pathogens and reduced the incidence of anthracnose in the field. The pure compound, identified as schizostatin, showed antifungal activity against plant fungal pathogens and reduced the symptoms of anthracnose and gray mild in the treated fruits. To the best of our knowledge, this is the first report showing the plant disease control efficacy of S. commune, which could be developed as a biochemical pesticide in further studies.
2. Materials and methods
2.1. Preparation of CF from white-rot fungus culture
White-rot fungus (Schizophyllum commune; Basidiomycota, Agaricomycetes, Agaricales, Schizophyllaceae) was obtained from National Academy of Agricultural Science (NAAS), Rural Development Administration, Korea (NAAS 04688) and cultured at 27 °C on potato dextrose agar (PDA) plates. The CF was obtained using the protocol by Chen and Huang [Citation8]. Briefly, from freshly subcultured plates, actively growing mycelium plug was used to inoculate 400 mL of potato dextrose broth (PDB) in a 1 L Erlenmeyer flask. The flasks were incubated at 27 °C for 4 weeks without shaking. The culture broth was harvested by filtration through Whatman No. 4 filter paper and centrifugation at 4000 g for 15 min to remove cell debris, followed by filtration of the supernatant through a 0.22 µm filter (Sartorius Stedim Biotech, Goettingen, Germany). The CF was stored at −20 °C until further use.
2.2. Fungal and bacterial plant pathogens
The fungal pathogens, B. cinerea KACC 40574 (causal pathogen of gray mold in pepper plants), C. gloesporioides KACC 40003, Colletotrichum dematium KACC 40013 and Colletotrichum coccodes (KACC40802) (causal pathogen of anthracnose), Alternaria solani KACC 44355 (causal pathogen of Alternaria leaf spot), Rhizopus stolonifer KACC 45160 (causal pathogen of Rhizopus fruit rot), and Diaporthe sp. KACC 40303 (causal pathogen of black dot fruit rot) were obtained from the Korean Agricultural Culture Collection (KACC), Wanju, Korea. The fungal isolates were maintained on PDA plates and stored at 4 °C. Mycelial plugs from the stored plates were subcultured on fresh PDA plates and incubated at 25 °C for further use.
The bacterial pathogens Ralstonia solanacearum (causal pathogen of bacterial wilt) and Pectobacterium carotovorum subsp. carotovorum (causal pathogen of soft rot) were revived from glycerol stock kept at −80 °C and revived on Luria-Bertani (LB) agar plates at 28 °C as necessary.
2.3. Inhibition assay of the CF against the mycelial growth of fungal pathogens
To analyze the activity of different concentrations of CF against the growth of fungal pathogens, a gradual twofold dilution was mixed with autoclaved, molten PDA at 45 °C to final concentrations of 50, 25, 12.5, 6, 3, and 1%. Distilled water (DW) was used as a control. One mycelial disk (4-mm-diameter) was cut from the edge of a 3- to 5-day-old colony of each fungus growing on PDA and put on the center of each plate with or without CF. The plates were maintained at 25 °C in darkness (or 16 h light/8 h dark) and measurements were recorded after 10 days of growth. The inhibition rate was determined according to the formula: [(average colony diameter of control − average colony diameter of treatment)/average colony diameter of control] × 100. The inhibitory concentration (LC50) was determined as the percentage of CF at which there was 50% inhibition of growth compared with the control. This experiment was repeated once under the same conditions. All treatments were performed independently three times in triplicate [Citation17].
2.4. Antagonism assay of the CF against bacterial pathogens
The antagonistic activity of the CF (or purified compound) against the bacterial pathogens, R. solanacearum and P. carotovorum was tested by the dual inoculation technique. Test plates were prepared with LB agar medium mixed with a cell suspension (1 × 106 CFU/mL) of R. solanacearum and P. c. subsp. carotovorum, and each concentration of CF (20 µl) was inoculated on paper disks (8 mm) laid on the LB test plates. Antagonistic activity was evaluated by measuring the widths of the inhibition zones after 2 days of incubation at 28 °C. The experiment was replicated two times with three plates per replicate.
2.5. Assay of CF on the incidence of anthracnose and gray mold using detached pepper fruits
The fully grown green-pepper fruits (c.v. Manita) with similar size were purchased from local markets and surface sterilized with 2% NaOCl for 5 min followed by three washings with sterile distilled water. To determine the protective effect of the CF, fruits were dipped in each concentration of CF for 30 min and dried at room temperature for 2 h. The disinfested pepper fruits (10 per replication) were pricked with a needle and a mycelial plug (5 mm) from an actively growing culture plate was placed on the pricked site, with the mycelium side touching the wound. The inoculated fruits were placed on a water-saturated Kimwipe (Kimberly-Clark, Irving, TX) in a plastic box and incubated at 25 °C in darkness (or 16 h light/8 h dark). After 7 days of incubation, the lesions on each fruit were assessed and expressed as the average disease area per fruit (%).Water treated fruits were used as negative control and this experiment was repeated three times.
2.6. Effect of the CF on anthracnose in pepper seedlings
Pepper seeds (c.v. Manita) were sown in a plastic tray (55 cm × 35 cm × 15 cm) containing commercial soil (Baroker; Seoul Bio Co., Ltd., Eumseong, Korea) and grown for 3–4 weeks. Seedlings at the two-leaf stage were transplanted into plastic pots (5 cm × 15 cm × 10 cm) containing the same commercial soil, raised in the plant growth room under 16 h illuminations at 28 ± 2 °C, and subsequently used for 3–4 weeks until 5–6 true leaves formed. To determine the optimal concentration for field application, we assessed the effects of 25% and 12.5% concentrations of CF on seedlings, because those concentrations showed high control efficacy in the detached fruit assay. In addition, to determine its preventive effects, the pepper seedlings were sprayed with CF until it ran off, and then conidial suspensions of C. gloeosporioides in 0.04% Silwet L-77 were sprayed onto the seedlings after 1 or 24 h. The treated plants were covered with transparent polyethylene bags for 24 h to maintain high humidity. The plants were recorded for disease symptoms at 7 and 14 days post inoculation (dpi). The disease severity was recorded using the disease score in a scale of 0 to 5 where 0 = no disease; 1 = 1–10% leaf area with lesions; 2 = 11–25% leaf area with lesions; 3 = 26–50% leaf area with lesions and limited chlorosis; 4 = more than 50% leaf area with lesions and extensive necrosis; 5 = defoliation. Disease incidence (%) was calculated based on the following formula: [Σ(Number of leaves in each score × numerical value of each score)/Total number of leaves counted × 5] × 100. Water was treated as a negative control. The plants were kept for observation of disease development for up to 30 dpi. There were three replicates (10 plants per replicate) for each treatment.
2.7. Assay of the CF to control anthracnose in the field
The field trial was conducted in the fields at the Iksan campus of Chonbuk National University, Korea, in 2018. Pepper seeds were surface-sterilized using 5% sodium hypochlorite for 10 min and then rinsed five times with sterile DW. Pepper seeds were germinated in sterilized soil containing a low level of nutrient soil-less mixture (Punong Co., Ltd., Gyeongju, Korea) and transferred to the rain-shielding fields after 3 weeks of growth. The plants were grown on in 20-cm-high ridges, which were covered with black and white polyethylene plastic film before transplanting. The treatment plots were replicated four times in a completely randomized design. The final concentration of CF and the optimal number of sprays required for disease control under field conditions were determined based on a pilot experiment using young seedlings. One week after transplantation, the plants were sprayed with 12.5% of the CF until it ran off, and 24-h later, the plants were challenge inoculated with the conidial suspension of C. gloeosporioides in 0.04% Silwet L-77. Two additional sprays of CF were applied at weekly intervals after the challenge inoculation. Plants sprayed with water at the time of CF application were served as negative control. A total of 20 plants were recorded for disease symptom development at 7 and 14 dpi and the first 5 true leaves were considered for disease scoring and the percentage of disease incidence was calculated as described above. The percentage of infected fruits was also recorded at 30 dpi and the percentage of diseased area of the fruits was calculated from randomly selected 20 fruits.
2.8. Purification and identification of the compound
From freshly subcultured PDA plates, an actively growing mycelial plug of S. commune was used to inoculate 400 mL of PDB in a 1 L Erlenmeyer flask. The flasks were incubated at 27 °C for 3 weeks without shaking. A total of 10 L of culture broth was harvested by filtration through Whatman No. 4 filter paper and centrifuged at 4000 g for 15 min to remove cell debris, followed by filtration of the supernatant through a 0.22 µm filter. The cell-free CF was extracted with acetone at 25 °C and the acetone was removed using a rotary evaporator (CCA-1111-CE; Eyela, Shanghi, China). The resulting extract was partitioned with chloroform. The chloroform extract was chromatographed on a silica gel column and eluted with a gradient of chloroform:methanol (25:1, 5:1, 0:100, v/v). Then, the fractions eluted from the 5:1 and 0:100 (v/v) ratio were combined and concentrated under reduced pressure. The resulting concentrated fraction was subjected to medium pressure liquid chromatography (MPLC, RP-C18) and eluted with 50% aqueous methanol to yield the active compound which was then identified by comparing the spectroscopic data with the previous literature [Citation18,Citation19].
2.9. In vitro antifungal assay of the pure compound
The active compound, schizostatin was dissolved in methanol to make a stock of 10 mg/mL. To assess the minimum inhibitory concentration (MIC), it was mixed with molten PDA to obtain final concentrations of 10, 25, 50, 100, and 150 µg/mL. A mycelial disk (4-mm-diameter) cut from the edge of a 10 day-old culture of each fungus growing on PDA was placed on the center of the plates and incubated at 25 °C for 7 days. The radius of the mycelial growth was measured and the inhibition rate was determined by calculating the percent decrease over the control. The IC50 was determined as the minimum concentration of compound required to inhibit mycelial growth by 50% compared to the control. The negative control was PDA containing 1% methanol.
2.10. Effect of the purified compound on detached pepper fruits
For fruit inoculation, green-pepper fruits were prepared as described above. To determine the protective effect of schizostatin, fruits were brushed with different concentrations of the compounds (10, 25, 50, 100, and 150 µg/mL) and kept at room temperature for 30 min. The treated fruits were then pricked with a needle and challenge inoculated by placing a mycelial plug (5 mm) from an actively growing culture plate, with the mycelium side facing the wound. The fruits were placed on a water-saturated Kimwipe (Kimberly-Clark, Irving, TX) in a plastic box and incubated at 25 °C in 16 h light/8 h dark. After 7 days of incubation, the lesions on each fruit were assessed, and results are expressed as average disease area per fruit (%). Fruits treated with water were used as the negative controls. This experiment was repeated twice.
2.11. Statistical analysis
Data were subjected to analysis of variance using SAS JMP software (SAS Institute, Cary, NC). Significant differences in the means of each treatment were determined using the least significant difference (LSD) test at P = 0.05. Data from each experiment were analyzed separately.
3. Results and discussion
3.1. In vitro antibiotic activity of CF against fungal plant diseases
The use of natural compounds for the development of eco-friendly pesticides has been gaining interest because of the hazardous influence of chemical pesticides on humans and the environment. Microorganisms such as fungi use antimicrobial compounds to survive in nature, and those compounds can be extracted and exploited as bioactive compounds [Citation20]. The white-rot fungus S. commune produces various metabolites, including antimicrobial substances [Citation14,Citation21].
In our search for eco-friendly alternatives to agrochemicals, we assessed the antimicrobial potential of CF obtained from S. commune against fungal and bacterial plant pathogens. The mycelial growth of fungal pathogens was significantly inhibited by incorporating various concentrations of CF into PDA. In general, as the concentration of the CF in the PDA increased, fungal growth was more suppressed (; ). The LC50 for C. gloeosporioides and B. cinerea were 20% and 15% CF, respectively. The mycelial growth of other Colletotrichum species, such as C. dematium and C. coccodes, which are also known to cause anthracnose in pepper plants, was also significantly reduced in comparison to control (; ). The results indicate that adding 1% of CF to PDA inhibited the growth of A. solani, B. cinerea, and C. gloeosporioides. Whether the amount of active ingredient in such a low concentration of CF is enough to exhibit antifungal activity is debatable, but previous reports suggested that very-low-concentration water extracts of S. commune inhibited the growth of wood-degrading fungi, with an MIC of 0.31 µg/µl for Lentinus sp. and 0.61 µg/µl for Microporus affinis and Microporus xanthopus [Citation21]. The extract of S. commune also showed antifungal activity against rubber-wood degrading fungi [Citation22]. The antifungal activity of S. commune was attributed to the presence of several interesting compounds, such as schizophyllan, glycerine, 5-heptyldihydro-2(3H)-furanone, 3,5-dihydroxy-6-methyl-2,3-dihydro-4H-pyran-4-one, and triacetin. CF from other higher fungi, such as Lentinula edodes, Clitocybe nuda, Ganoderma lucidum, and Tremella aurantialba, showed antifungal activity against species of Colletotrichum, Alternaria, and Phytophthora [Citation8]. In most previous studies using S. commune, the antimicrobial properties were extracted from fungal biomass [Citation16,Citation21]. Because we used CF for this study, various compounds in the extracts, including exopolysaccharides, β-glucans, and protein complexes, might have contributed to the antimicrobial activity [Citation23]. Intriguingly, the CF was not effective against R. stolonifer. The use of stolon in the growth of R. stolonifer might allow it to circumvent the antagonism. So far as we know, this is the first report of antifungal activity against plant pathogens from the CF of S. commune.
Figure 1. Effect of various concentrations of culture filtrate on in vitro mycelial growth inhibition of fungal pathogens. Each fungus, (A) Alternaria solani (B) Botrytis cinerea (C) Colletotrichum coccodes (D) C. dematium (E) C. gloeosporioides (F) Diaporthe sp., and (G) Rhizopus stolonifer was inoculated in the center of PDA amended with various concentrations of culture filtrate. The picture was taken 7 days after incubation.
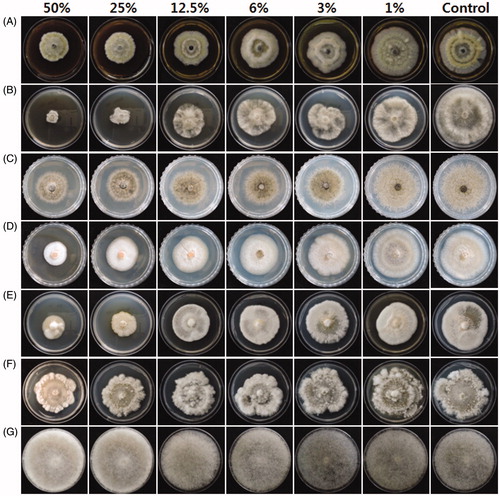
Table 1. Effect of culture filtrate on the in vitro mycelial growth of fungal pathogens after 7 days of incubation.
3.2. Antagonistic activity of CF against bacterial plant pathogens
The CF of S. commune in this study did not show antibacterial activity against the bacterial pathogens, R. solanacearum or P. carotovorum subsp. carotovorum. Previous studies reported that metabolites such as schizophyllan produced by S. commune displayed antifungal activity [Citation14] and that oxidized schizophyllan, scleraldehyde possessed antimicrobial activity against a broad range of bacterial species [Citation24]. In addition, the aqueous and methanol extract of S. commune inhibited the growth of Bacillus subtilis, Bacillus cereus, Staphylococcus aureus, and Agrobacterium tumefaciens [Citation25,Citation26]. However, in another study, extracts of S. commune showed no or poor antimicrobial activity against all of the tested microorganisms, including Escherichia coli, B. cereus, and S. aureus [Citation23], which corresponds to the results of this study. David et al. [Citation27] suggested that Gram-negative bacteria were more sensitive to oils extracted from S. commune than Gram-positive bacteria. Tripathi and Tiwary [Citation28] reported that Gram-positive bacteria such as B. subtilis and S. aureus were more sensitive to CF and methanol extracts of S. commune. This inconsistency of results might result from differences in the metabolites produced by various fermentation conditions of S. commune.
The production of mycelia and metabolites are significantly influenced by fermentation technology and nutrient supplementation in the media [Citation21,Citation29]. In addition, several factors during fermentation, such as pH, nutrients, age of culture, culture space, substrate concentration, and conservation methods, are responsible for the quality and quantity of antimicrobial compounds [Citation30]. The inconsistent results between studies indicate that different kinds and amounts of metabolites are produced, depending on the cultivation/fermentation conditions and strains used.
3.3. Control efficacy for anthracnose and gray mold in the detached pepper fruits
To determine the optimum concentration for the control of anthracnose and gray mold, we treated the detached pepper fruits with different dilutions of CF and assessed the control efficacy (). Disease incidence decreased significantly when the fruits were treated with a high concentration (50% and 25%) of CF. The control effect was 95.5% for anthracnose and 96.0% for gray mold by treatment with a 25% CF dilution. The disease incidence gradually increased with the dilution-fold, with control efficacy drastically decreased by treatment with 6.25% CF.
Figure 2. Effect of various concentrations of culture filtrates on the incidence of anthracnose and gray mold in detached pepper fruits. Mycelial plugs of Colletotrichum gloeosporioides (Cg) and Botrytis cinerea (Bc) were inoculated on hot-pepper fruits pretreated with different concentrations of culture filtrates. Distilled water (Con) was used as the negative control. (A) Data for disease area (%) are presented as mean ± standard deviation. Different letters above the bars indicate significant differences at P = 0.05. (B) The picture was taken 7 days after inoculation.
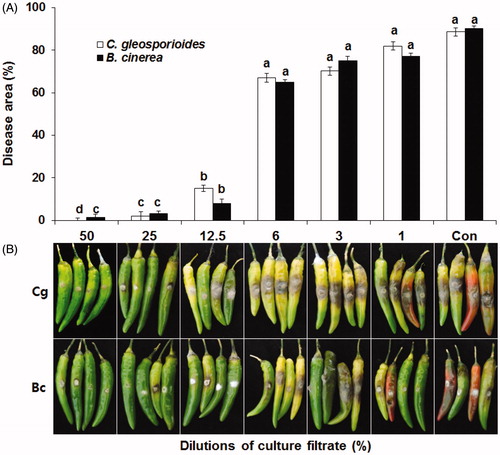
However, CF applied after the challenge inoculation with the pathogen produced no significant reduction in disease incidence compared to the control, indicating that the CF provided preventive benefits but no therapeutic effect. Therefore, CF has to be applied before pathogen infection. In the context of biocontrol efficacy and mass production by high dilution, we selected two concentrations of CF, 25.0% and 12.5%, for further assays.
3.4. Control efficacy of CF against anthracnose in pepper plants (pot assay)
Based on the dilution factors obtained from the results of the pepper fruit assay, we scaled up and assayed the CF’s preventive effects by applying 1 and 24 h before infection. When pepper seedlings were challenge-inoculated 1 h after CF spray, disease incidences were reduced to 20.2% and 22.1%, respectively with the treatment of 25% and 12.5% CF compared to 59.5% in the control. When the seedlings were inoculated 24 h after CF treatment, disease incidences were reduced to 22.0% and 25.5%, respectively, with 25% and 12.5% CF application in comparison to 57.5% in the control. Overall, the effects of both concentrations were effective in reducing the disease developments for 10 days. Therefore, we determined that a 12.5% dilution would be effective before the onset of infection, and we used that dilution for the rest of our assays.
3.5. Control effect of CF in the field
Treatment of pepper plants with CF significantly reduced the symptoms of anthracnose compared to the pathogen-only control. The disease incidence was 20.0% by treatment with 12.5% CF compared to 65.0% in the pathogen-only treatment at 7 dpi (). The disease spread continuously in the control (73.5%), but the disease incidence at 14 dpi was suppressed to 22.4% by the treatment of CF. Symptom development indicated that CF treatment inhibited the spread of disease to neighboring healthy plants more than 7 days. In case of fruits, disease incidence was 98.0% in the pathogen only treatment, whereas 7.7% in CF treated plants. In addition, the diseased area in the fruits was significantly suppressed to 5.7% by treatment of CF compared to 74.8% in the control (). Yohalem et al. [Citation31] reported that aqueous extracts of substrate used for Agaricus bisporus mushroom production effectively controlled apple scab disease caused by the fungus Venturia inaequalis in a field assay, though with lower efficacy than the chemical captan. Viecelli et al. [Citation32] reported that aqueous extracts of the fungus Pycnoporus sanguineus reduced angular leaf spot disease in beans by 49.0% in a field assay. However, that extract did not inhibit the mycelial growth, sporulation, or conidial germination of the pathogen Pseudocercospora griseola. The treated plants showed increased peroxidase and polyphenol oxidase activity, along with higher chlorophyll and protein content, which suggests induced systemic resistance (ISR) in the plants.
Table 2. Control effects of anthracnose incidence in the leaves and fruits by treatment of culture filtrate from Schizophyllum commune.
Proteins, tamavidin, and lectins of fungal origin have been reported to be effective antifungal, antibacterial, nematicidal, and insecticidal agents in plants [Citation33–36]. However, in spite of those studies, very few studies have reported field assays of fungal extracts. In this study, the CF of S. commune restricted development of disease in field conditions at concentration of 12.5%. The results indicate the CF of S. commune could be a potent alternative to chemical fungicides for use in organic cultivation. However, further studies are necessary to investigate ISR, microbiome maintenance, and restructuring.
3.6. Identification of the active compound and its antifungal activity
Many compounds have been extracted from the mycelial culture or mushroom of S. commune and assayed for their beneficial effects. Sizofiran, an antitumour polysaccharide extracted from the culture broth of S. commune, served as an effective immune‐therapeutic agent against cervical carcinoma [Citation37]. In addition, several different polysaccharides, such as hetero‐β‐glucans and their protein complexes, have been developed for beneficial effects from the mushroom and mycelia of S. commune, particularly in China and Japan [Citation23]. Phenolic compounds with antioxidant activity, phenyl benzoate and 4-(phenyl methoxy) phenol, and antibacterial compound, pyrrolo (1, 2-a) piperazine-3, 6-dione were also identified from the culture extract [Citation28]. More recently, S. commune reduced the adult emergence of tobacco cutworm, indicating the genotoxic and cytotoxic effects of the fungus and suggesting the potential of S. commune as an insecticidal agent [Citation15]. As discussed earlier, the small changes of fermentation conditions such as age of culture, nutrient, temperature, and space, influence on the production of secondary metabolites. Therefore, the difference in active compound might be due to the culture conditions provided.
In this study, the CF was extracted with acetone and further purified with MPLC. The mass spectrometry indicated that the active compound is schizostatin which was previously identified as a novel squalene synthase inhibitor [Citation19]. When schizostatin was mixed in PDA media, a minimum of 50 µg/mL was needed to inhibit the growth of B. cinerea whereas 10 µg/mL showed an inhibitory effect against C. gloeosporioides (). The compound inhibited the growth of C. gloeosporioides and B. cinerea by 92.4% and 87.3%, respectively at a concentration of 150 µg/mL. The LC50 was determined to be around 40.0 µg/mL for C. gloeosporioides and 95.0 µg/mL for B. cinerea. The metabolites produced by S. commune displayed antifungal activity [Citation14,Citation21] and scleraldehyde showed antibacterial activity [Citation24]. However, in this study, we found that schizostatin did not exhibit antibacterial activity against the bacterial plant pathogens, such as R. solanacearum and P. carotovorum even at high concentration of 400 µg/mL. This result corresponds to previous reports in which schizostatin showed no in vitro antimicrobial activity at a concentration of 1000 μg/mL against S. aureus, B. subtilis, E. coli, Mycobacterium smegmatis, Proteus vulgaris, Proteus mirabilis, Candida albicans, or Mycoplasma mycoides [Citation19]. Interestingly, schizostatin has been indicated a light stimulated autoinhibitor of growth in S. commune responsible for the asymmetric pattern of dikaryotic growth [Citation38]. The periodic growth inhibition in S. commune could be either by schizostatin itself or through intracellular accumulation of farnesol, which is a close relative to schizostatin and had antimicrobial property [Citation39,Citation40].
Table 3. Effect of schizostatin on in vitro mycelial growth of fungal plant pathogens.
3.7. Control efficacy of schizostatin against anthracnose in detached pepper fruits
To investigate the in vivo control activity of the purified schizostatin, we treated pepper fruits with the compound and then challenge-inoculated them with C. gloeosporioides or B. cinerea. For C. gloeosporioides infection, significant disease reduction was observed from treatment with 10 µg/mL, with 97.8% and 100.0% control effects with 100 µg/mL and 150 µg/mL treatment, respectively (). The effective control of gray mold required a higher concentration than the control of anthracnose. The control effect for gray mold was 83.2% and 94.6% by treatment with 100 µg/mL and 150 µg/mL, respectively. Thus, schizostatin was the key compound contributing to the overall biocontrol activity of CF from S. commune. Although the content of schizostatin in the diluted CF would be lower than that in the solution of the pure compound, the suppression of disease incidence was more efficient when CF was used. The higher inhibition by CF could be due to the presence of compounds other than schizostatin, as discussed above. Overall, the results of this study indicate that schizostatin is a potent agent for controlling anthracnose and gray mold in peppers, both in the field and postharvest stages.
Figure 3. Effect of various concentrations of schizostatin on the incidence of anthracnose and gray mold in the detached pepper fruits. Mycelial plugs of Colletotrichum gloeosporioides and Botrytis cinerea were inoculated onto hot-pepper fruits pretreated with different concentrations of purified compound. Distilled water (Con) was used as negative control. Data are presented as mean ± standard deviation. Different letters above the bars indicate significant differences at P = 0.05.
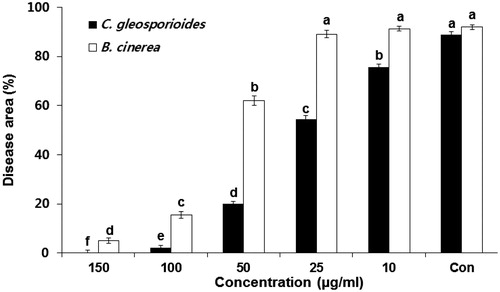
Disclosure statement
No potential conflict of interest was reported by the authors.
Additional information
Funding
References
- Oo MM, Oh SK. Chilli anthracnose (Colletotrichum spp.) disease and its management approach. Kor J Agric Sci. 2016;43:153–162.
- Sun C, Mao SL, Zhang ZH, et al. Resistances to anthracnose (Colletotrichum acutatum) of Capsicum mature green and ripe fruit are controlled by a major dominant cluster of QTLs on chromosome P5. Sci Hortic. 2015;181:81–88.
- Hong JK, Yang HJ, Jung H, et al. Application of volatile antifungal plant essential oils for controlling pepper fruit anthracnose by Colletotrichum gloeosporioides. Plant Pathol J. 2015;31:269–277.
- Veloso J, Diaz J. Induced resistance to Botrytis cinerea in Capsicum annuum by a Fusarium crude elicitor fraction, free of proteins. Plant Biol J. 2013;15:1040–1044.
- Heydari A, Pessarakli M. A review on biological control of fungal plant pathogens using microbial antagonists. J Biol Sci. 2010;10:273–290.
- Barseghyan GS, Barazani A, Wasser SP. Medicinal mushrooms with antiphytopathogenic and insecticidal properties. In: Petre P, editor. Mushroom biotechnology: developments and applications. Verlag: Academic Press; 2015. p. 137–154.
- Stodulkova E, Císarova I, Kolarík M, et al. Biologically active metabolites produced by the Basidiomycete Quambalaria cyanescens. PLOS ONE. 2015;10:e0118913.
- Chen JT, Huang JW. Antimicrobial activity of edible mushroom culture filtrates on plant pathogens. Plant Pathol Bull. 2010;19:261–270.
- Jo WS, Hossain MA, Park SC. Toxicological profiles of poisonous, edible, and medicinal mushrooms. Mycobiology. 2014;42:215–220.
- Sivanandhan S, Khusro A, Paulraj MG, et al. Biocontrol properties of Basidiomycetes: an overview. J Fungi (Basel). 2017;3:2.
- Hibbett DS, Binder M, Bischoff JF, et al. A higher-level phylogenetic classification of the fungi. Mycol Res. 2007;111:509–547.
- Ohm RA, de Jong JF, Lugones LG, et al. Genome sequence of the model mushroom Schizophyllum commune. Nat Biotechnol. 2010;28:957–963.
- Zhang GQ, Chen QJ, Sun J, et al. Purification and characterization of a novel acid phosphatase from the split gill mushroom Schizophyllum commune. J Basic Microbiol. 2013;53:868–875.
- Hao LM, Xing XH, Li Z, et al. Optimization of effect factors for mycelia growth and exopolysaccharide production by Schizophyllum commune. Appl Biochem Biotechnol. 2010;160:621–631.
- Kaur M, Chadha P, Kaur S, et al. Schizophyllum commune induced genotoxic and cytotoxic effects in Spodoptera litura. Sci Rep. 2018;8:4693.
- Kumari M, Survase SA, Singhal RS. Production of schizophyllan using Schizophyllum commune NRCM. Bioresour Technol. 2008;99:1036–1043.
- Fang XL, Li ZZ, Wang YH, et al. In vitro and in vivo antimicrobial activity of Xenorhabdus bovienii YL002 against Phytophthora capsici and Botrytis cinerea. J Appl Microbiol. 2011;111:145–154.
- Kogen H, Tago K, Kaneko S, et al. Schizostatin, a novel squalene synthase inhibitor produced by the mushroom, Schizophyllum commune. II. Structure elucidation and total synthesis. J Antibiot. 1996;49:624–630.
- Tanimoto T, Onodera K, Hosoya T, et al. Schizostatin, a novel squalene synthase inhibitor produced by the mushroom, Schizophyllum commune. I. Taxonomy, fermentation, isolation, physico-chemical properties and biological activities. J Antibiot. 1996;49:617–623.
- Alves M, Ferreira IFR, Dias J, et al. A review on antimicrobial activity of mushroom (Basidiomycetes) extracts and isolated compounds. Planta Med. 2012;78:1707–1718.
- Teoh YP, Don MM, Ujang S. Nutrient improvement using statistical optimization for growth of Schizophyllum commune and its antifungal activity against wood degrading fungi of rubberwood. Biotechnol Progress. 2012;28:232–241.
- Teoh YP, Mashitah MD, Ujang S. Production of biomass by Schizophyllum commune and its antifungal activity towards rubberwood-degrading fungi. Sains Malaysiana. 2017;46:123–128.
- Fagade OE, Oyelade AA. A comparative study of the antibacterial activities of some wood‐decay fungi to synthetic antibiotic discs. Electron J Environ Agric Food Chem. 2009;8:184–188.
- Jayakumar GC, Kanth SV, Chandrasekaran B, et al. Preparation and antimicrobial activity of scleraldehyde from Schizophyllum commune. Carbohydr Res. 2010;345:2213–2219.
- Emsen B, Kocabas A, Çınar S, et al. In vitro cytotoxicity, antibacterial and antioxidant properties of various extracts from Schizophyllum Commune fr. Fresen Environ Bul. 2017;26:1144–1153.
- Karthiga K, Sivakumar T. Antibacterial activity of methanolic extract of Basidiomycetes. Asian J Chem. 2010;22:1637–1638.
- David OM, Fagbohun Ed, Oluyege AO, Adegbuyi A. Antimicrobial activity and physicochemical properties of oils from tropical macrofungi. J Yeast Fungal Res. 2012;3:1–6.
- Tripathi AM, Tiwary BN. Biochemical constituents of a wild strain of Schizophyllum commune isolated from Achanakmar-Amarkantak Biosphere Reserve (ABR), India. World J Microbiol Biotechnol. 2013;29:1431–1442.
- Guo X, Zou X, Sun M. Optimization of a chemically defined medium for mycelia growth and polysaccharide production by medicinal mushroom Phellinus igniarius. World J Microbiol Biotechnol. 2009;25:2187–2193.
- Hernandez D, Cardell E, Zarate V. Antimicrobial activity of lactic acid bacteria isolated from Tenerife cheese: initial characterization of plantaricin TF711, a bacteriocin-like substance produced by Lactobacillus plantarum TF711. J Appl Microbiol. 2005;99:77–84.
- Yohalem DS, Nordheim EV, Andrews JH. The effect of water extracts of spent mushroom compost on apple scab in the field. Phytpopathology. 1996;86:914–922.
- Viecelli CA, Stangarlin JR, Kuhn OJ, et al. Induction of resistance in beans against Pseudocercospora griseola by culture filtrates of Pycnoporus sanguineus. Trop Plant Pathol. 2009;4:87–96.
- Pohleven J, Brzin J, Vrabec L, et al. Basidiomycete Clitocybe nebularis is rich in lectins with insecticidal activities. Appl Microbiol Biotechnol. 2011;91:1141–1148.
- Pohleven J, Renko M, Magister S, et al. Bivalent carbohydrate binding is required for biological activity of Clitocybe nebularis lectin (CNL), N,N¢-diacetyllactosediamine (GalNAcß1-4GlcNAc, LacdiNAc)-specific lectin from basidiomycete C. nebularis. J Biol Chem. 2012;287:10602–10612.
- Smid I, Gruden K, Buh Gasparic M, et al. Inhibition of the growth of Colorado potato beetle larvae by macrocypins, protease inhibitors from the parasol mushroom. J Agric Food Chem. 2013;61:12499–12509.
- Takakura Y, Oka N, Suzuki J, et al. Intercellular production of tamavidin 1, a biotin-binding protein from tamogitake mushroom, confers resistance to the blast fungus Magnaporthe oryzae in transgenic rice. Mol Biotechnol. 2012;51:9–17.
- Shittu OB, Alofe FV, Onawunmi GO, et al. Mycelial growth and antibacterial metabolite production by wild mushrooms. Afr J Biomed Res. 2005;8:157–162.
- Klein KK, Landry J, Friesen T, et al. Kinetics of asymmetric mycelial growth and control by dikaryosis and light in Schizophyllum commune. Mycologia. 1997;89:916–923.
- Nickerson KW, Atkin AL, Hornby JM. Quorum sensing in dimorphic fungi: farnesol and beyond. Appl Environ Microbiol. 2006;72:3805–3813.
- Singh A, Del Poeta M, Lipid signaling in pathogenic fungi. Cell Microbiol. 2011;13:177–185.