Abstract
Androgen-independent prostate cancer accounts for mortality in the world. In this study, various extracts of a medical fungus dubbed Ganoderma formosanum were screened for inhibition of DU145 cells, an androgen-independent prostate cancer cell line. Results demonstrated that both hexane (GF-EH) and butanol (GF-EB) fraction of G. formosanum ethanol extract inhibited DU145 cell viability in a dose-dependent manner. GF-EH induced cell-cycle arrest in G1 phase of DU145 cells via downregulation of cyclin E2 protein expression. In addition, GF-EB triggered extrinsic apoptosis of DU145 cells by activating caspase 3 gene expression resulting in programed cell death. Above all, both GF-EH and GF-EB show lower toxicity to normal human fibroblast cell line compared to DU145 cell, implying that they possess specific drug action on cancer cells. This study provides a molecular basis of G. formosanum extract as a potential ingredient for treatment of androgen-independent prostate cancer.
1. Introduction
Prostate cancer is the second leading cause of cancer death among men in the United States [Citation1]. In general, prostate cancer develops slowly and may be symptomless for several years, thus threatens long-term health [Citation2]. Androgen deprivation therapy (ADT) is one of the major treatments for patients to suppress the growth of prostate cancer cells, but long-term antiandrogen therapy may cause hormone refractory [Citation3]. Therefore, alternative treatments are critical for the suppression of androgen-independent prostate cancer progression.
Ganoderma spp., generally recognized as Lingzhi in Asia, is a nutraceutical with various pharmacological effects, such as immunomodulation, anti-inflammation, and antitumor [Citation4]. Moreover, several clinical trials of Ganoderma drugs are still on going for the treatment of lung and breast cancer [Citation4].
It is worth noting that various Ganoderma ingredients have been reported to possess the potential of suppressing growth of prostate cancer cells [Citation5]. For instance, ganoderic acid DM (GA-DM), a triterpenoid isolated from G. lucidum, functions as 5-alpha-reducatase to inhibit the transformation of testosterone to dihydrotestosterone, leading to suppression of androgen-dependent prostate cancer cell (PC-3) proliferation [Citation6]. In addition, ganoderol B extracted from G. lucidum have been demonstrated to bind to androgen receptor, resulting in inhibition of androgen-induced prostate cancer cells (LNCaP) [Citation7]. Moreover, GLF4 extracted from G. lucidum shows other mechanisms of Ganoderma extracts to inhibit prostate cancer cells, such as apoptosis and cell cycle arrest [Citation8]. These studies suggest that Ganoderma spp. is a potential medical mushroom to screen antiprostate cancer drugs. However, the studies of inhibitory activity of Ganoderma extracts on androgen-independent prostate cancer remain unclear.
Among more than 80 types of Ganoderma, an endemic species in Taiwan dubbed G. formosanum has recently attracted attention for its antimelanin formation potential [Citation9]. In our previous studies, GFE-EA, a subfraction of ethanolic extract of G. formosanum mycelia has been demonstrated that it inhibits melanin synthesis during zebrafish embryos development [Citation10]. However, other medical potential of G. formosanum mycelia extract remains unknown.
The aim of this study is to screen novel ingredients from submerged fermentation of G. formosanum mycelia on down-regulation of androgen-independent prostate cancer cell growth via in vitro cell drug screening model, and the mechanism was investigated through protein expression and cell cycle analysis. This study provides new insight into G. formosanum as a nutraceutical for treatment of prostate cancer.
2. Materials and methods
2.1. Fungal strain and submerged fermentation
Ganoderma formosanum ATCC76537 obtained from the American Type Culture Collection (Manassass, VA) was cultured on potato dextrose agar (Acumedia, Baltimore, MD). Then 8 cm2 agars with mycelium was incubated in 250 mL flask containing 100 mL medium (45 g/L of glucose, 3.75 g/L of yeast extract, KH2PO4 0.88 g/L, MgSO4.7H2O 0.5 g/L, Vit B1 0.05 g/L, pH is adjusted to 5) for preculture at 25 °C and 120 rpm for 7 days. After 7 days of preculture, 10% (v/v) of preculture broth was inoculated to 6.8 L bioreactor (Winpact, Waltham, MA) containing 5 L medium (49.2 g/L of glucose, 4.9 g/L of yeast extract, KH2PO4 0.88 g/L, MgSO4.7H2O 0.5 g/L, Vit B1 0.05 g/L, pH is adjusted to 5.3), and cultured at 25 °C, 120 rpm agitation and aeration at 1 vvm for 7 days. The compositions of medium are referred to our pervious study [Citation11].
2.2. Cell culture
In this study, DU145 (BCRC60348), an androgen-independent prostate cancer cell line, was purchased from Bioresource Collection and Research Center (BCRC, Hsinchu, Taiwan). It was cultured in 90% MEM/EBSS medium (Hyclone, Logan, UT) with 10% FBS, 50 U/mL penicillin and 50 μg/mL streptomycin at 37 °C in an incubator containing 5% CO2.
2.3. Preparation of G. formosanum extracts
Ganoderma formosanum mycelium was collected and dried for 48 h via lyophilizer after fermentation (T10, HCS, New Taipei City, Taiwan). The dried mycelium was extracted with reverse osmosis water at 60 °C for 1 h. After 1-h extraction, the extract was collected and centrifuged at 6500×g for 20 min at 4 °C using Centrifuge 5430 R (Eppendrof, Hamburg, Germany). The water extract mixed with three volumes 95% ethanol to precipitate polysaccharides and filtrated with No.1 filter paper (ADVANTEC, Dublin, CA) to separate water extract and polysaccharides. The extracted mycelium subsequently extracted with 95% ethanol at 60 °C for 1 h. Both crude extracts were concentrated until totally dried under reduced pressure to obtain crude extracts with rotary evaporator (N-1200A, EYELA, Tokyo, Japan). After that, the ethanol extract was dissolved into deionized water, and subsequently partitioned with hexane, ethyl acetate, and butanol (Sigma-Aldrich, St Louis, MO) to obtain different fractions. All the extracts were prepared as stock concentrations of 20 mg/ml in dimethylsulfoxide (DMSO) and stored at –20 °C. The yield of extract was calculated as: (amount of dried crude extracts)/(amount of dried mycelium) × 100%
2.4. Cell proliferation assay
Cell proliferation assay was determined with WST-1 assay. The prostate cancer cell lines were seeded at 96 well plate (1000 cells/well). After 24 h post-seeding, the medium was replaced with new medium contained various concentration of extracts dissolving in 1% DMSO and incubated at 37 °C for 24 or 48 h. After incubation, the medium was removed and supplemented with medium containing 5% WST-1 reagent (Roche, Mannheim, Germany) and subsequently incubated at 37 °C in the dark for 30 min. Cell viability was determined by detecting the absorbance at 450 nm with spectrometry (Multiskan GO, Thermo Scientific, Waltham, MA).
2.4.1. RNA extraction and quantitative real time-PCR
After treatment with G. formosanum extracts, cells were harvested by centrifugation (1000×g, 5 min). The cell pellet was washed twice with cold PBS and resuspended in 1 ml cold PBS. Total RNA of collected cells (106 cells) were extracted with novel total RNA mini kit (NovelGene, Taipei, Taiwan). mRNA was reversed transcribed by using RevertAid H Minus First Strand cDNA Synthesis Kit (Thermo Scientific, Waltham, MA). The cDNA obtained was kept at −80 °C for long-term storage. The primer used in this study is listed in Supplementary Table S1. Quantitative real-time PCR was conducted with KAPA SYBR FAST ABI Prism qPCR kit (Kapabiosysrems, Wilmington, MA) on StepOne Plus (Applied Biosystems, Waltham, MA). The real-time PCR conditions were 10 min at 95 °C followed by 40 cycles of 15 s at 95 °C and 60 s at 60 °C. Data were normalized by the level of beta-actin in each sample and calculated by 2-ΔΔCt manner to compare the relative gene expression between different groups.
2.5. Cell cycle analysis by flow cytometry
The cell cycle phase distributions were determined by cytoFLEX (Bechman Coulter Inc., Indianapolis, IN). Cells treated with G. formosanum extracts were collected and washed twice with cold PBS. In addition, the cells were fixed with 1 ml PBS and 9 ml methanol for 1 h at 4 °C. After that, the cells were washed twice with cold PBS to remove the methanol and then incubated with 0.5 ml FxCycle PI/RNase Staining solution (Thermo Scientific, Waltham, MA) at room temperature for 30 min. Finally, these cells were analyzed through cytoFLEX flow cytometry.
2.6. Protein extraction and expression analysis
After treatment with extracts for 48 h, culture dishes with cells were placed on ice and washed the treated cells with cold phosphate buffer saline (PBS), and protein was subsequently extracted with lysis buffer (G-Biosciences/Geno Technology, Inc., St. Louis, MO). The harvested cells were homogenized with electric homogenizer, and the lysate was subsequently centrifuged at 14,000 rpm for 20 min at 4 °C by centrifuge (Eppendorf, Hauppauge, NY). After centrifugation, the supernatant was transferred to new microcentrifuge tube, and the protein concentration was determined through Bradford Protein Assay (Bio-Rad Laboratories Taiwan Ltd., Hercules, CA).
Protein expression levels of cyclin were analyzed by western blotting. The purified protein was electrophoresed by SDS-PAGE (10% polyacrylamide separating gel with 4% polyacrylamide stacking gel), and the gel was electro-transferred onto polyvinylidene difluoride (PVDF) membrane. The free binding site in the membranes was blocked with 5% fat-free milk in PBST for 1 h. Further, the membrane was incubated with cyclin antibody (Proteintech, Rosemont, IL) at 4 °C for overnight. Finally, the membrane was then incubated with goat antimouse IgG or goat antirabbit IgG secondary antibody at room temperature for 1.5 h. The antibody was detected with ECL™ Western Blotting Detection Reagent (GE Healthcare Life Sciences, Logan, UT).
2.7. Statistical analysis
All data in this study were performed at least triplicate and expressed as mean ± SD. Statistical analysis was conducted using Duncan’s test with SAS software. A p-value less than 0.05 was recognized as statistical significance.
3. Results and discussion
3.1. Preparation of mycelia
Ganoderma extract has been demonstrated superior antiproliferation on prostate cancer cells among a total 201 Basidiomycetes extracts [Citation12]. Moreover, ethanol extract of G. lucidum fruiting bodies is reported to inhibit DU145 prostate cancer cells (IC50 = 132 ppm), but it is worth noting that the high cost of Ganoderma fruiting bodies is the issue of concern [Citation13]. Therefore, submerged fermentation is employed to produce G. formosanum mycelia for the extraction of bioactive ingredients in this study (). This technique not only provides stable culture condition to control mycelia quality, but also reduces the cost of traditional Ganoderma cultivation [Citation4].
Figure 1. Production of G. formosanum mycelia with submerged fermentation. (A) G. formosanum mycelia grown on PDA; (B) Flask; and (C) Bioreactor.
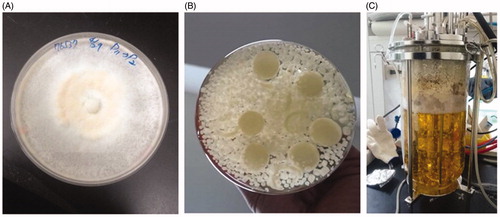
For production of G. formosaum for extraction, mycelia were seeded in PDA agar (), and subsequently transfer into flask for further submerged fermentation (). According to our previous studies [Citation11], optimized medium for increasing biomass of G. formosanum was used for preculture condition, and the culture broth in the flask was inoculated into bioreactor for production of G. formosanum mycelia for 7 days preculture (). Taken as a whole, the progress of two stages takes 14 days to produce mycelia for bioactive ingredients extraction.
3.2. Antiproliferation effects of G. formosanum extracts on DU145 cells
First of all, antiproliferation effect of aqueous (GF-A) and ethanolic extract (GF-E) of G. formosanum on DU145 prostate cancer cells were compared in WST-1 assay. As shown in , antiproliferation activity of GF-E on DU145 cells is significantly higher (p < 0.05) than groups treated with GF-A after 48 h in a dose-dependent manner (50–200 ppm). After 48 h treatment of GF-E, 20% DU145 cells decreased when compared to vehicle group without drug treatment.
Figure 2. Cell viability analysis of DU145 cells after treated with G. formosanum extracts. (A) DU145 cells treated with GF-A and GF-E for 48 h. (B) DU145 cells treated with various GF-E fractions for 48 h. (C) Human fibroblast cells treated with G. formosanum extracts for 48 h. Statistical differences were analyzed by Duncan’s new multiple range test. Values are presented as mean ± SE. Three independent experiments with different superscripts (A, B, C, D, E) are significantly different (p < 0.05).
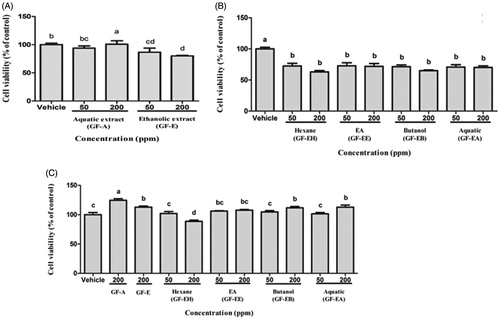
In order to purify more effective extracts, various fractions of GF-E were further purified by liquid-liquid partition extraction. In comparison of hexane (GF-EH), ethyl acetate (GF-EE), butanol (GF-EB), and aquatic (GF-EA) fraction of GF-E, both GF-EH and GF-EB demonstrated stronger cell toxicity on DU145 cells than other fractions (). Nearly 40% of DU145 cells are inhibited after treated with GF-EH or GF-EB for 48 h, and IC50 of these two groups are 266.9 ± 28.8 and 254.3 ± 31.7 ppm, respectively.
Cell viability assay indicated that crude ethanolic extract of G. formosanum mycelia shows higher antiproliferation activity on DU145 cells than that of aquatic extract (). Similar results have been found in previous study that ethanolic G. tsugae extract exerts antiproliferation effect on colorectal cancer cells, but water extract does not possess any inhibitory activity [Citation12]. In addition, GF-EH and GF-EB possessed more effective anticancer cell growth potential compared to other fractions of GF-E (), and these results are firstly reported that small compounds of G. formosanum demonstrate anticancer property instead of polysaccharide [Citation14]. There are ways for prostate cancer therapy including surveillance, radiation therapy, chemotherapy, surgery, androgen-deprivation therapy, and palliative care. Some of them may cause side effects such as nausea, burning urination, urinary incontinence, and impotence [Citation15]. As for chemotherapy, Zhu et al. reported curcumin-induced antiproliferation of prostate cancer cells (22RV1, PC‐3, and DU145) [Citation16]. The IC50 was around 13 μmol/L (around 4.8 ppm) toward DU145 cells. Our samples showed weaker antiproliferation effect than those from curcumin, and it might be owing to the impurity of crude extract. For application in human cancer therapy, further research is needed to validate the antiproliferation efficacy and biosafety by studying the constituents of G. lucidum in the future.
The water extract of Ganoderma lucidum has extremely bitter taste where Japanese scientists discovered a group of triterpenoids (also known as Ganoderma acid) are unique metabolites of Ganoderma contributing the bitter taste. Hsu and Cheng [Citation4] summarized various kinds of triterpenoids with different chemical structure. The triterpenoid structure of Ganoderma belongs to lanostane triterpenes group. Due to the differences in strains, cultivation methods and conditions like harvest time and maturity, there are huge structural and content various in triterpenoids production. In addition, four types of immunoregulatory proteins, Lingzhi-8 (Lz-8), Fip-gts, GMI, and Fip-gat, have been isolated and purified from Ganoderma [Citation17]. Above all, GE-EB (200 ppm) does not lead to normal human fibroblast cells death, and GF-EH (200 ppm) shows lower cytotoxicity to normal cells (15% inhibition rate) than that of DU145 cells (). These results implied that GF-E fractions exert more specific cytotoxicity to prostate cancer cells. In addition, previous study indicated G. lucidum whiskey extract upregulates apoptotic related gene expression of cancer cell but not noncancerous cell line (HEK293), suggesting Ganoderm extracts might possess cancer specific cytotoxicity [Citation13].
3.3. Effects of G. formosanum extracts on apoptosis of DU145 cells
To investigate the mechanism of anticancer action of G. formosanum extracts, molecular biotech tools from DNA, RNA, and protein were employed to analyze the drug action of GF-EH and GF-EB. As we know, DNA fragmentation is a feature of apoptosis [Citation18]. DNA fragmentation is a sensitive and cost-effective approach to measure apoptosis. As shown in , total DNA of DU145 treated with GF-EH and GF-EB for 48 h is separated through electrophoresis, and DNA fragmentation was visualized in GF-EB group but not GF-EH group.
Figure 3. DNA and RAN analysis of DU145 cells after treated with G. formosanum extracts. (A) DNA fragmentation analysis of DU145 cells treated with GF-EH (200 ppm) and (B) cells treated with GF-EB (200 ppm) (white arrow indicates the DNA fragmentation). M stands for Marker, and lanes 1 and 2 stands for G. formosanum extracts; (C) Relative caspase 3 gene expression of DU145 treated with or without 200 ppm G. formosanum extracts for 48 h. Significant difference (p < 0.05) between various groups is presented as (B).
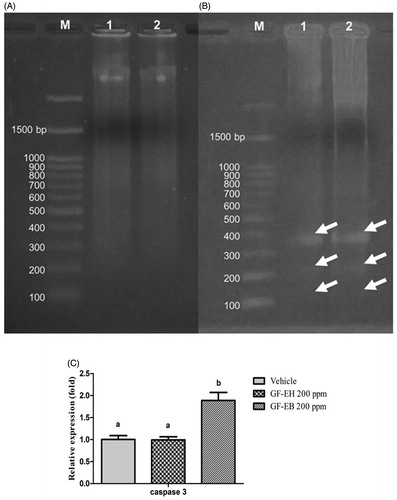
Furthermore, relative gene expression of caspase 3 of DU145 cells treated with GF-EB and GF-EH was compared with control group to reveal the molecular mechanism of G. formosanum extracts (). Results of real-time PCR indicated that a 1.8-fold relative gene expression of caspase 3 of DU145 cells is significantly increased after treatment of GF-EB; however, cells treated with GF-EH does not result in any change of caspase 3 gene expression when compared to control group.
Electrophoresis analysis demonstrates the DNA of DU145 cells treated with GF-EB was fragmented (), suggesting it might be resulting in apoptosis, also known as programed cell death, to inhibit cancer cell proliferation. In contrast, GF-EH group does not show any DNA ladder during the electrophoresis ().
Caspase 3 is required for activation of apoptosis, leading to cleavage of chromosomal DNA [Citation18]. Therefore, relative gene expression of caspase 3 of DU145 cells treated with G. formosanum extracts was measured and compared to vehicle group. As illustrated in , cells treated with GF-EB demonstrates a 1.8-fold increase of caspase 3 gene expression when compared to vehicle group, and this result is consistent with the aforementioned DNA fragmentation of cells treated with GF-EB, suggesting that GF-EB suppresses prostate cancer cells growth by activating caspase 3 gene expression to trigger programed cell death. Furthermore, cancer cell was reported to downregulate apoptosis progress to survive, so GF-EB can reverse this mechanism [Citation19]. As a matter of fact, previous study also indicated that various Ganoderma extracts kill cancer cells through apoptotic pathway [Citation20,Citation21].
It is worth noting that GF-EH dose not suppress prostate cancer cell growth via apoptotic pathway. Based on previous studies, Ganoderma extracts were found to inhibit various types of cancer cells by influencing normal cell cycle progression, such as breast, bladder, and cervical cancer [Citation5]. Therefore, the cell cycle distribution of DU145 cells treated with G. formosanum extracts was investigated to reveal the anticancer mechanism.
3.4. Cell cycle is regulated by G. formosanum extracts
Cell proliferation is mediated through cell cycle progression, and many anticancer drugs are reported to suppress cancer cell growth via cell cycle arrest [Citation22,Citation23]. To investigate the mechanism of GF-EH and GF-EB, cell cycle progression of DU145 cells treated with G. formosanum extracts are measured by western blotting and flow cytometry.
After treating DU145 cells with G. formosanum extracts for 48 h, western blotting analysis indicated that GF-EH reduces the protein level of cyclin A2, B2, and E2 in a dose-dependent manner but does not influence the level of cyclin D (). On the other hand, apart from cyclin A2, cyclin protein expression of DU145 cells treated with GF-EB is similar to vehicle group ().
Figure 4. Effect of G. formosanum extracts on cyclin protein expression in DU145 cells. DU145 cells were treated or not with G. formosanum extracts 200 ppm for 48 h. The expression levels of cyclins are presented with β-actin as control.
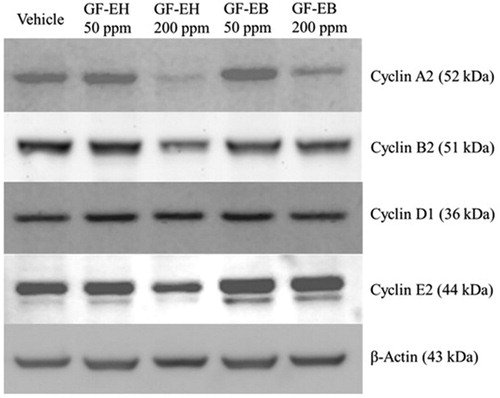
Cell cycle is composed of four stages including G1, S, G2, M, and different cyclin-CDK complexes are responsible for initiating each stage [Citation24]. For instance, Cyclin E2 is associated with CDK2 to trigger cell transition from G1 to S phase, suggesting it may be rate-limiting for G1 phase [Citation25]. Western blotting result indicates that the protein level of cyclin E2 is decreased of DU145 cells treated with GF-EH but not that with GF-EB (). Cyclin A2 and B2 are highly expressed in S-G2 and G2-M phase, the former is required for DNA replication, and the latter is critical for G2/M transition [Citation26,Citation27]. Furthermore, both of Cyclin A2 and B2 protein level are also reduced by GF-EH (), demonstrating that GF-EH is a cell cycle inhibitor to suppress DU145 cells proliferation.
To confirm aforementioned antiproliferation mechanism of G. formosanum extracts, flow cytometry was employed to measure the profile of cell cycle stage of DU145 cells treated with G. formosanum extracts for 48 h. As illustrated in , the distribution of G0G1, S, G2M stage of control group is 63.99, 15.54, and 19.26%, respectively. However, cell cycle progression of GF-EH group is quite different from control group, the distribution of G0G1, S, G2M stage are 85.07, 6.26, and 7.63%, demonstrating cell cycle of DU145 cells treated with GF-EH arrest in G0G1 phase (). In contrast to GF-EH, the distribution of G0G1, S, G2M stage of GF-EB group is 61.3, 17.88, and 17.1%, respectively, suggesting that it is similar to control group ().
Figure 5. Effect of G. formosanum extracts on cell cycle distribution of DU145 cells. (A) Vehicle group; (B) cells treated with GF-EH (200 ppm); and (C) cells treated with GF-EB (200 ppm) for 48 h.
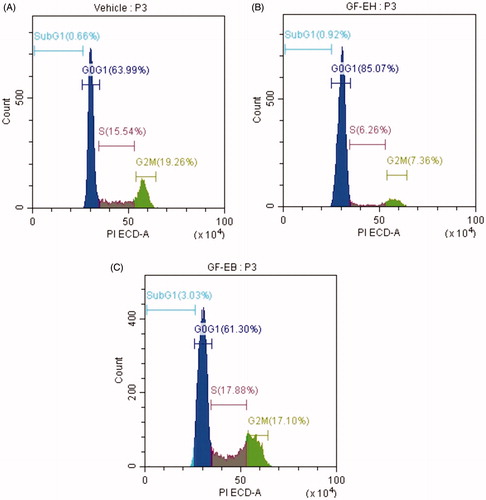
To confirm aforementioned mechanism, DU145 cells treated with GF-EH were sorted via flow cytometry, and the results are consistent with protein expression profile of GF-EH groups. Owing to downregulation of cyclin E2, cell cycle of GF-EH group arrests in G1 phase when compared to vehicle group (). On the other hand, GF-EB only downregulates cyclin A2 (), so the distribution of cell cycle demonstrates slight increase in S phase compared to control group (17.88 versus 15.54%) (); however, the distribution of cell cycle of GF-EH is similar to pathway instead of cell cycle arrest, implying it is not a cell cycle inhibitor. Interestingly, G. lucidum whiskey extract was reported to alter gene expression of two types of androgen-independent prostate cancer cell line (PC3 and DU145), especially cell cycle and apoptosis-related genes, which is consistent with our data [Citation13].
In conclusion, this study firstly demonstrates that G. formosanum mycelial extracts possess antiproliferation activity on androgen-independent prostate cancer cell line (DU145). Furthermore, molecular evidences indicate that GF-EH and GF-EB cause cancer cell death through different pathways, which might be helpful to develop prostate cancer therapy with combination effects. More importantly, lower toxicity was observed in normal human cells treated with both GF-EH and GF-EB (). However, the active compound(s) of GF-EH and GF-EB remain unclear, hence the industrial submerged cultivation of G. formosanum for production of these two extracts require further purification and identification of the key compounds in the future. Once the active ingredient(s) of G. formosanum is confirmed, the drug action and potential of GF-EB and GE-EH for antiprostate cancer will be clearer.
In summary, this is the first report to discuss the inhibitory effect of Ganoderma formosanum mycelium extracts on androgen-independent prostate cancer. Results shows that both hexane (GF-EH) and butanol (GF-EB) fractions of G. formosanum ethanol extract inhibited DU145 cell viability in a dose-dependent manner. GF-EH induced cell-cycle arrest in G1 phase of DU145 cells via down-regulation of cyclin E2 protein expression. In addition, GF-EB triggered extrinsic apoptosis of DU145 cells by activating caspase 3 gene expression resulting in programed cell death. It is worth noting that GF-EH and GF-EB exhibited no adverse effect toward normal human fibroblast cell line. This study provides a molecular basis of G. formosanum extract as a potential solution for androgen-independent prostate cancer therapy. Purification and identification of bioactive constituents of Ganoderma will be the next challenge.
Supplemental Material
Download Zip (29.5 KB)Acknowledgments
The authors are very grateful to Dr. Shella P. Santoso from Widya Mandala Catholic University Surabaya for the article proof reading and English editing.
Disclosure statement
No potential conflict of interest was reported by the author(s).
Additional information
Funding
References
- Siegel RL, Miller KD, Fedewa SA et al. Colorectal cancer statistics. CA Cancer J Clin. 2017;67(3):177–193.
- Huang S-Y, Huang G-J, Wu H-C, et al. Ganoderma tsugae inhibits the SREBP-1/AR axis leading to suppression of cell growth and activation of apoptosis in prostate cancer cells. Molecules. 2018;23(10):2539.
- Qu L, Li S, Zhuo Y, et al. Anticancer effect of triterpenes from Ganoderma lucidum in human prostate cancer cells. Oncol Lett. 2017;14(6):7467–7472.
- Hsu K-D, Cheng K-C. From nutraceutical to clinical trial: frontiers in ganoderma development. Appl Microbiol Biotechnol. 2018;102(21):9037–9051.
- Wu G-S, Guo J-J, Bao J-L, et al. Anti-cancer properties of triterpenoids isolated from Ganoderma lucidum–a review. Expert Opin Invest Drugs. 2013;22(8):981–992.
- Johnson BM, Doonan BP, Radwan FF, et al. Ganoderic acid DM: an alternative agent for the treatment of advanced prostate cancer. Open Prost Cancer J. 2010; 3:78–85.
- Liu J, Shimizu K, Konishi F, et al. The anti-androgen effect of ganoderol B isolated from the fruiting body of Ganoderma lucidum. Bioorg Med Chem. 2007;15(14):4966–4972.
- Zaidman B-Z, Wasser SP, Nevo E, et al. Androgen receptor-dependent and-independent mechanisms mediate Ganoderma lucidum activities in LNCaP prostate cancer cells. Int J Oncol. 2007;31(4):959–967.
- Hsu K-D, Chen H-J, Wang C-S, et al. Extract of Ganoderma formosanum mycelium as a highly potent tyrosinase inhibitor. Sci Rep. 2016;6:32854.
- Hsu K-D, Chan Y-H, Chen H-J, et al. Tyrosinase-based TLC autography for anti-melanogenic drug screening. Sci Rep. 2018;8(1):401.
- Hsu K-D, Wu S-P, Lin S-P, et al. Enhanced active extracellular polysaccharide production from Ganoderma formosanum using computational modeling. J Food Drug Anal. 2017;25(4):804–811.
- Zaidman B-Z, Wasser SP, Nevo E, et al. Coprinus comatus and Ganoderma lucidum interfere with androgen receptor function in LNCaP prostate cancer cells. Mol Biol Rep. 2008;35(2):107–117.
- Kao CH, Bishop KS, Xu Y, et al. Identification of potential anticancer activities of novel Ganoderma lucidum extracts using gene expression and pathway network analysis. Genomics Insights. 2016;9:GEI. S32477.
- Wang C-L, Lu C-Y, Hsueh Y-C, et al. Activation of antitumor immune responses by Ganoderma formosanum polysaccharides in tumor-bearing mice. Appl Microbiol Biotechnol. 2014;98(22):9389–9398.
- Litwin MS, Tan HJ. The diagnosis and treatment of prostate cancer: a review. JAMA. 2017;317(24):2532–2542.
- Zhu M, Zheng Z, Huang J, et al. Modulation of miR-34a in curcumin-induced antiproliferation of prostate cancer cells. J Cell Biochem. 2019;120(9):15616–15624.
- Cao Y, Xu X, Liu S, et al. Ganoderma: a cancer immunotherapy review. Front Pharmacol. 2018;9:1217.
- Zhang JH, Xu M. DNA fragmentation in apoptosis. Cell Res. 2000;10(3):205–211.
- Ouyang L, Shi Z, Zhao S, et al. Programmed cell death pathways in cancer: a review of apoptosis, autophagy and programmed necrosis. Cell Prolif. 2012;45(6):487–498.
- Hsin I-L, Ou C-C, Wu M-F, et al. GMI, an immunomodulatory protein from Ganoderma microsporum, potentiates cisplatin-induced apoptosis via autophagy in lung cancer cells. Mol Pharmaceutics. 2015;12(5):1534–1543.
- Dan X, Liu W, Wong JH, et al. A ribonuclease isolated from wild Ganoderma lucidum suppressed autophagy and triggered apoptosis in colorectal cancer cells. Front Pharmacol. 2016;7:217.
- Takahashi A, Kimura F, Yamanaka A, et al. Metformin impairs growth of endometrial cancer cells via cell cycle arrest and concomitant autophagy and apoptosis. Cancer Cell Int. 2014;14(1):53.
- Smith ML, Murphy K, Doucette CD, et al. The dietary flavonoid fisetin causes cell cycle arrest, caspase-dependent apoptosis, and enhanced cytotoxicity of chemotherapeutic drugs in triple-negative breast cancer cells. J Cell Biochem. 2016;117(8):1913–1925.
- Sherr CJ, Bartek J. Cell cycle–targeted cancer therapies. Annu Rev Cancer Biol. 2017;1(1):41–57.
- Gudas JM, Payton M, Thukral S, et al. Cyclin E2, a novel G(1) cyclin that binds Cdk2 and is aberrantly expressed in human cancers. Mol Cell Biol. 1999;19(1):612–622.
- Bolognese F, Wasner M, Dohna CL, et al. The cyclin B2 promoter depends on NF-Y, a trimer whose CCAAT-binding activity is cell-cycle regulated. Oncogene. 1999;18(10):1845–1853.
- Gong D, Ferrell JE. The roles of cyclin A2, B1, and B2 in early and late mitotic events. Mol Biol Cell. 2010;21(18):3149–3161.