Abstract
Use of nanoparticles (NPs) in several commercial products has led to emergence of novel contaminants of air, soil and water bodies. The NPs may exhibit greater ecotoxicity due to nano-scale dependent properties over their bulk counterparts. The present investigation explores the effect of in vitro supplementation of TiO2, silica and silver NPs on radial growth and ultrastructural changes in the hyphae and spores of two mushroom genera, Ganoderma lucidum and Volvariella volvaceae. A concentration dependent decrease in radial growth on NP amended potato dextrose agar medium was recorded. However, in comparison to control, there was decrease in radial diameter on supplementation with TiO2 NPs while an increase was recorded for silica and silver NPs amendments as compared to their bulk salts at same concentrations after 48 h of incubation. Optical microscopy studies showed decrease in the number of spores while increase in spore diameter and thinning of hyphal diameter on NPs supplementation. Scanning electron microscopy analysis of fungal growth showed presence of deflated and oblong spores in two fruiting strains of Ganoderma while Volvariella exhibited decreased sporulation. Further, hyphal thinning and branching was recorded in response to NP amendments in both the test mushrooms. Enhancement of protein content was observed on NP compared to bulk supplementation for all cultures, concentrations and hours of incubation except for TiO2 NPs. Likewise, bulk and NP supplementations (at 100 mg L−1) resulted in enhanced laccase activity with occurrence of laccase specific protein bands on SDS-PAGE analysis.
1. Introduction
Nanoparticles (NPs), 0-D nanomaterials (NMs), have potential applications in electronics and commercial products, including imaging and medical apparatus [Citation1], fabrics and cosmetics [Citation2,Citation3]. Most NPs that are currently in use are of transition metals, silicon, carbon (CNTs), and metal oxides (zinc dioxide and titanium dioxide) [Citation4]. Among these, the major commercial applications include the use of photocatalytic titania NPs for fabricating self-cleaning window panes and other construction materials, silver NPs (AgNPs) in home appliances, paints, textiles and personal care products [Citation1,Citation5] and silica NPs for development of novel construction materials, in pharmaceutics and in energy and electronics [Citation6]. This has culminated to release of these NPs in the open, dynamic systems like air, soil and water ecosystems. The environmental exposure on release of these novel materials may result in myriad of interactions in air, soil, or aquatic ecosystems leading to cascade of detrimental impacts. For instance, TiO2 is chemically stable in dark but absorbs in UV region of EM spectrum inducing some chemical reactions due to photocatalytic properties [Citation7]. Similarly, nanoscale silicon exhibits unique size-dependent optical properties (multi-colour emission, non-photobleaching characters), are amenable to surface functionalization [Citation6] and exhibit interaction with biological molecules in comparison to semi-conductor nanoparticles [Citation7]. It is, therefore, desirable that the environmental risks posed by NPs due to their aberrant mobility and reactivity and thus nano-ecotoxicity aspects must be determined using effective quantitative analytical methods [Citation8].
Microorganisms are one of the most sensitive group of unicellular to multicellular organisms that can be utilized as the indicators for assessing the ecotoxicity of the xenobiotics. Among these, group fungi are comprised of multicellular filamentous microbes and include basidiomycetes encompassing several mushroom species. The mushrooms are key scavengers which play unique and prime role in geochemical recycling of the elements [Citation9] and therefore, can be a potential candidate to assess the ecotoxicity impacts of the nanomaterials. Alteration in the physiology and metabolism showcased as morphological changes in these fungi in presence of transition/heavy metal/non-metal/metal oxide salts and their nanoparticles can be useful to interpret extent of toxicity of engineered NPs. The exposure to heavy metal in ionic form or their NPs may alter the total protein content and enzyme profile besides initiating expression of novel stress-signalling, -combating or -neutralizing proteins on exposure [Citation10,Citation11].
Here, we have tested the response of two mushroom fungi, Ganoderma lucidum (Order Polyporales) and Volvariella volvaceae (Order Agaricales) belonging to two different orders and families. G. lucidum, Lingzhi or Reishi mushroom (of pharmaceutical significance in oriental medicine), voraciously grow on wood logs [Citation12] while the genera Volvariella is an edible paddy straw mushroom which possesses both nutritional and medicinal properties. Due to their preference for a lignocellulose substrate, both genera exhibit greater lignocellulase activities and decompose decayed wood and paddy straw substrates, respectively. The lignocellulolytic activity involves “laccase (EC 1.10.3.2)” enzyme which permits depolymerization of highly polydispersed polyphenolic lignin to small compounds [Citation13]. The versatility of the laccase enzyme complex mandates its environmental application including agri-waste management and decontamination of cyclic organic xenobiotics. Therefore, these two fungi have a brighter potential for success in bioremediation due to their bioprospecting properties. The aim of this study was to investigate the growth rate, ultrastructural changes in the hyphae and spore besides the biochemistry particularly protein content of G. lucidum and V. volvaceae in response to bulk salt and nanoparticle stress of titanium, silver and silicon elements. However, a broader objective was to observe the tweaking of expression of the laccase enzyme or other related proteins with application of bulk and nano-scale formulations of the three test elements.
2. Materials and methods
2.1. Chemicals
The prepared media, potato dextrose agar was purchased from Hi-Media Laboratory Chemicals Pvt. Ltd, Mumbai, India. The commercial titania (TiO2), silica (SiO2) and silver (Ag) nanoparticles were purchased from Sisco Research Laboratory Pvt. Ltd., Mumbai, India.
2.2. Microbial cultures
The microbial cultures, G. lucidum (two each of fruiting [GL-I and GL-II] and non-fruiting strains [GL-III and GL-IV]) and V. volvaceae were procured from the Mushroom Research Complex, Department of Microbiology, Punjab Agricultural University, Ludhiana, Punjab, India. These were maintained on potato dextrose agar (PDA) medium at 30 °C ± 1 °C.
2.3. Culturing conditions and stereomicroscopy
The PDA media were supplemented with bulk salts of Titanium, silver and sodium besides the aqueous nanoparticle sols of titanium dioxide, silver and silicon dioxide at five different working concentrations of 0, 25, 50, 75 and 100 mg L−1. The plated medium was inoculated with a 5 mm mycelium disk punched from fresh culture and incubated at 30 °C ± 1 °C for four days. The colony growth or the radial diameter was measured using Macroviewer (Leica MZ 60, Wetzlar, Germany) equipped with CCD camera (Leica DFC290) at four different time intervals, i.e., 24, 48, 72 and 96 h of incubation.
2.4. Optical research microscopy
The mycelial growth scrapped from agar media after 96 h of incubation was stained with lactophenol cotton blue stain. The fungal growth was placed on a clean glass slide. A drop of the lactophenol cotton blue stain was placed on the slide. Using a sterilized needle, the fungal growth was teased and the cover slip was placed cautiously avoiding insertion of any air bubble. The prepared slides were observed for the number and shape of spores and spore and hyphal diameter at 200X and 400X magnifications using optical research microscope (Leica DM5000B).
2.5. Scanning electron microscopy
The fungal biomass/hyphae were processed for SEM studies by chemical fixation using 2.5% cacodylate buffered glutaraldehyde as primary fixative at 4 °C for 24 h [Citation14]. The hyphal biomass was then washed with 0.1 M cacodylate buffer thrice for 15 min each at 4 °C to wash off the primary fixative. Secondary fixation was performed with 1% osmium tetraoxide for 2 h at 4 °C. The excess secondary fixative was washed with 0.1 M cacodylate buffer three times for 15 min each at 4 °C. Later, the hyphal biomass was dehydrated using graded ethanol series (30%–100%) for 10 min each followed by drying in Critical Point dryer (Polaron 3000E, East Sussex, United Kingdom). The dried samples were placed on aluminum stub using double sided carbon tape and sputter coated with gold in Ion Sputter coater (Hitachi E-1010; Hitachi, Tokyo, Japan). The sputtered samples were viewed under SEM (Hitachi s-3400N; Hitachi, Tokyo, Japan) at 15 kV accelerating voltage in secondary electron (SE) analysis mode.
2.6. SEM-Energy dispersive spectroscopy
The SEM-EDS was performed to ascertain the presence of Ti, Si and Ag elements on the surface of the hyphae by using EDS module (Thermo Noran System Six; Thermo Fisher Scientific, Walthum, MA, USA) attached with SEM. The hyphal biomass was processed as discussed above. The EDS analysis was performed in whole surface spectra and point and shoot spectra modes. The latter involved taking 10–15 regions/points of interest on the surface to obtain % atom and % weight occurrence of elements on the sample surface.
2.7. Total soluble protein content of the test mushroom cultures
The effect of the test culture(s)-nanoparticle interaction on the total soluble protein content was determined using standard Lowry’s method [Citation15]. The mycelia growth of hyphal cultures (G. lucidum four strains and V. volvaceae) was scrapped from the agar surface and then crushed in liquid nitrogen using sterilized pestle mortar. The extract obtained was mixed with phosphate buffer, precipitated with chilled acetone and was used for estimation of the protein content.
2.8. Sodium dodecyl sulfate polyacrylamide gel electrophoresis (SDS-PAGE)
The SDS-PAGE of the crude proteins was performed in polyacrylamide gel to fractionate proteins according to their molecular weight by using mass standard. This will be a useful indicator to help in determining the similarity between strains of fungus [Citation16].
2.9. Laccase enzyme activity
Laccase estimation was carried out as per the method of [Citation17] with some modifications [Citation18]. One mL of enzyme extract was added to 3 mL of buffered guaiacol in spectrophotometer cuvette, mixed and tube was placed in UV-Vis spectrophotometer (SL-159, ELICO Ltd., Hyderabad, India) immediately. Change in absorbance was recorded for every 15 s upto 120 s at 495 nm. The constant change in absorbance was plotted for laccase activity. An increase in OD by 0.01 in 60 s was taken as one unit. Enzyme activity was expressed as unit/mL culture filtrate or units/mL enzyme extract.
2.10. Statistical analysis
The data was subjected to analysis of variance (ANOVA) using generalized linear model procedure of SAS (Version 9.2; SAS Inst., Cary, NC, USA). The two mushroom genera, concentration of the chemical/NP formulation used and hours of incubation were analyzed as per the split plot design [Citation19]. The treatment differences were determined using Fisher’s protected LSD method at p ≤ 0.05.
3. Results
3.1. Effect of titanium, silicon dioxide and silver NPs on radial growth
The stereomicroscopy studies on radial hyphal growth of the mycelium of the four strains of G. lucidum and one strain of V. volvaceae grown on PDA medium supplemented with bulk salts and NPs of Ti, Si and Ag at five different concentrations (0, 25, 50, 75 and 100 mg L−1) () were conducted. The different concentrations of the bulk/nano-formulations supplemented in PDA media had caused statistically non-significant increase/decrease in the radial growth of the five test cultures except for silver nitrate and sodium silicate (bulk salt sources). The hours of incubation affected the radial growth of the test cultures for all the three types of bulk salts and NP amendments used. Several interactions also affected the radial growth of test fungi with respect to bulk and nanoformulation amendments. The bulk salt supplementation of Ti element exhibited a dual interaction for culture × concentration, Ti (bulk) and Si NPs for culture × hours besides all amendments exhibited concentration × hours interactions. Only Ti (bulk) source exhibited triple interaction for culture × concentration × hours.
Table 1. Analysis of variance for concentrations, hours of incubation and different sources for the radial growth of test mushroom cultures.
A variable response was recorded for the fruiting and non-fruiting strains of G. lucidum on Ti bulk salt supplementation in PDA media (, Supplementary Figure 1). A numerical decrease in radial growth diameter was observed for GL-I at 24, 48 and 96 h of incubation for 75, 25 and 100 mg L−1 working concentrations. However, the other fruiting strain, GL-II exhibited numerical decrease till 4th day of incubation while a numerical increase in diameter was observed post 48 h of incubation for 50, 75 and 100 mg L−1 amendments. The non-fruiting strains (GL-III and GL-IV) behaved quite differently among each other. A numerical decrease was recorded till 3rd and 4th day of incubation at 25, 75 and 100 mg L−1 respectively for GL-III while a numerical increase till 96 h of incubation was recorded in 100 mg L−1 treatment for GL-IV. A statistically higher radial diameter was observed for 25 and 50, 75 and 100 mg L−1 concentrations at 72 and 24 h of incubation, respectively, for V. volvaceae. Highest increase in radial diameter was observed for TiO2 NPs at 100 mg L−1 for GL-I, 75 mg L−1 for GL-III and Volvariella for 25 mg L−1 working concentrations post 4th day of incubation (Supplementary Table 1). Similarly, GL-I and Volvariella exhibited increased radial growth at 100 mg L−1 concentration while GL-III at 25 mg L−1 at 96 h of incubation while the radial growth of GL-II and GL-IV was inhibited by AgNP amendment. Silver nitrate bulk salt supplementation resulted in decrease in the radial hyphal growth of all the five test fungal cultures (Supplementary Table 2). The silica NP supplementation decreased the radial growth of GL-II, GL-IV and Volvariella in a concentration dependent manner post 4 days of incubation. However, the sodium silicate supplementation decreased the radial hyphal growth for all the five test cultures (Supplementary Table 3).
Table 2. Effect of supplementation of five different concentrations of TiO2 bulk on radial growth of Ganoderma lucidum and Volvariella volvaceae at every 24 h of incubation till day four.
3.2. Effect of NPs on spore count and spore/hyphae diameter
The optical research microscopy studies have revealed the variable effect of supplementation of NPs/bulk salts of Ti, Si and Ag on spore number, spore and hyphal diameter of G. lucidum and V. volvaceae cultures (). The highest number of spores were reported in GL-II on supplementation of SiNPs (97.0) followed by AgNPs (85.0). However, the highest spore diameter was observed in GL-II culture grown on TiO2 NP supplemented PDA media (21.77 μm) while maximum hyphal diameter was recorded for V. volvaceae+TiO2 NPs treatment (10.45 μm) (, Supplementary Figure 2). The supplementation of both bulk and nanoparticle formulations of the three elements resulted in formation of no spores in GL-III while negligible sporulation was observed in GL-IV.
Table 3. Effect of supplementation of bulk salts and nanoparticles of titanium dioxide, silica and silver on spore number, spore and hyphal diameter of Ganoderma lucidum and Volvariella volvaceae.
A numerical increase in the hyphal diameter was recorded for all the cultures on supplementation of NPs of TiO2, Si and Ag except GL-IV for Ag NP amendment in contrast to their respective bulk salts. However, gradual thinning of the hyphae was recorded with respect to the respective control cultures (, Supplementary Table 4). Along with hyphal thinning another morphological variation was observed i.e., extensive branching of hyphae which increased on NPs supplementation. Therefore, at higher concentration, the networking of mycelia was enormous which indicates a physiological alteration in the fungal cells in the presence of NPs.
Figure 1. Scanning Electron micrographs showing the effect of supplementation of bulk salts of titanium, silver and silicon elements on spore and hyphae of Ganoderma lucidum and Volvariella volvaceae. (i), (ii) and (iii) Ganoderma (GL-II) for bulk salts of Ti, Si and Ag; (iv), (v) and (vi) Ganoderma (GL-IV) for bulk salts of Ti, Si and Ag; and (vii), (viii), (ix), (x), (xi) and (xii) Volvariella for bulk salts of Ti, Si and Ag respectively (Magnification 1.00 k).
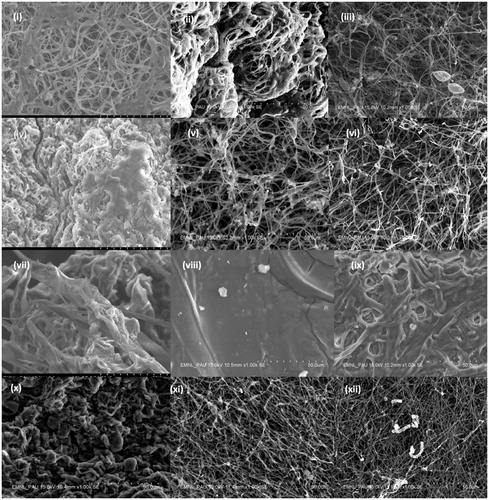
3.3. SEM-Energy dispersive X-ray spectroscopy (SEM-EDS)
The SEM-EDS studies of the surface of the hyphal mass showed difference in the % weight and % atom composition of various elements. The characteristic peaks appeared for the supplemented test elements, Si (1.740 keV for Kα x-ray lines), Ag (2.984 and 3.348 keV for Lα and Lβ X-ray lines) and Ti (4.510 keV for Kα x-ray lines). The higher % atom occurrence of Si, and Ag and Ti was recorded on NPs supplemented potato dextrose broth compared to their bulk counterparts indicating specific adsorption of NPs on hyphae ().
Table 4. Occurrence of Ti, Ag and Si elements on the surface of hyphal biomass in the test mushroom cultures as obtained through SEM-EDS spectroscopy.
3.4. Total soluble protein content of the test mushroom cultures
The total soluble protein content recorded after 96 h of incubation of the test mushroom cultures in the presence of bulk salts as well as NPs was observed to vary significantly (). All the test cultures varied significantly on amendment of the bulk and nanoformulations of the three test elements except silver nitrate bulk salt treatment. A significant variation in protein content with respect to working concentrations was recorded for test cultures for all the three element amendments (both bulk and NP) (Supplementary Figure 3). The dual interaction of culture × concentration was statistically significant for Ti bulk and silver nitrate salt amendments. The highest protein content was recorded for Ganoderma GL-III in the presence of Ti bulk salt at 100 mg L−1 and GL-II in TiO2 NPs at 100 mg L−1 treatment (Supplementary Table 5).
Table 5. Analysis of variance for concentrations of bulk and nanoformulations of three elements and total protein content of Ganoderma lucidum and Volvariella volvaceae.
Similarly, irrespective of the test cultures, sodium silicate supplementation resulted in decrease in the total hyphal protein content in a concentration dependent manner except at 100 mg L−1 working concentration for GL-II culture. However, the silica NPs supplementation resulted in drastic increase in the total protein content with highest average content at 50 mg L−1 concentration. The maximum average protein content irrespective of the concentration of the salt/NP supplementation was exhibited by GL-III (2199.68 µg g−1) and GL-II (2020.58 µg g−1) for Ti bulk salt treatment. V. volvaceae and G. lucidum GL-I exhibited highest average protein contents for AgNO3 salt and silver NPs respectively (Supplementary Table 5).
3.5. Sodium dodecyl sulfate polyacrylamide gel electrophoresis of the crude protein extract
The crude protein samples were fractionated and resolved as protein bands by SDS-PAGE to observe any variation in the banding pattern of proteins of different test strains of G. lucidum and of V. volvaceae. Two to seven bands were observed in the samples with slight variation in the banding pattern among the G. lucidum and V. volvaceae. The proteins with average molecular weight of 66.0 kDa, 45.0 kDa, 35.0 kDa, 40 kDa and 28.0 kDa were observed in the SDS-PAGE gel (Supplementary Figure 4).
3.6. Laccase enzyme activity
Irrespective of the test cultures, supplementation of all three elements, Ti, Si and Ag resulted in highest mean average increase in the laccase activity at 100 mg L−1 concentration for both bulk and NP formulations (). Similarly, irrespective of element concentrations used, the highest average laccase activity was recorded for GL-II for Ti (bulk), Si and Ag (both bulk and nano-formulation) and Volvariella for TiO2 NPs. The highest laccase activity was observed for Ganoderma lucidum (GL-III) (0.384 U mg−1) followed by V. volvaceae in presence of 100 mg L−1 TiO2 bulk salt (0.368 U mg−1). An increasing trend down the concentration was observed for laccase activity for both bulk salt and nanoparticles of the three elements, i.e., laccase activity increased with the increasing concentration of the test salt/nanoparticle formulation used though at lower concentration of 25 and/or 50 mg L−1 a decrease in activity can be observed w.r.t control treatment.
Table 6. Effect of supplementation of bulk salts and nanoparticles on laccase enzyme activity (IU mg−1) of Ganoderma lucidum and Volvariella volvaceae at 96 h of incubation.
4. Discussion
This is the first report on qualitative morphological and physiological variations of two test mushroom cultures; G. lucidum and V. volvaceae on exposure to two physical states i.e., as bulk and nano-scale formulation of three elements viz., titanium, silicon and silver. Our study accentuates that bulk salts and their nanoformulations behaved differently regarding the radial hyphal growth of the test cultures. The objective of testing the effect of nanoparticles of titanium dioxide, silver and silica is their rampant use in several commercialized cosmetics and pharmaceuticals products. Therefore, the release of these nanoparticles in open system as soil ecosystem may culminate to negative effects on soil microbiota particularly the detrimental effects on soil beneficial microbes which are required to be discerned. As given in the results section, the supplementation of bulk silicon and silver sources resulted in the decrease in the radial growth of all the test fungi in a concentration dependent manner. Only bulk Ti supplementation resulted in significant increase in radial growth for GL-IV at 100 mg L−1 and for V. volvaceae at 25 and 50, 75 and 100 mg L−1 concentrations at 72 and 24 h of incubation, respectively. A similar numerical increase in radial diameter of G. lucidum fruiting and non-fruiting strains has been reported by Goyal et al. [Citation20] in response to 10 mg L−1 supplementation of bulk selenium salt.
The spore number was decreased by NP supplementation of the Ti and Ag bulk formulations for four Ganoderma strains and GL-I and Vovariella, respectively. The hyphal diameter increased for bulk supplementation of Ti, Ag and Si elements w.r.t their NP formulations. In consensus to our results, Goyal et al. [Citation20] have recorded concentration dependent gradual decrease in hyphal diameter in response to Se bulk supplementation. The spore diameter decreased for all the test elements among five cultures except a numerical increase for GL-I and GL-II on Ti bulk application. However, a significant decrease in spore diameter has been reported for 20 and 25 mg L−1 Se application [Citation20].
In general, the protein content of the test mushroom cultures was decreased by application of Si and Ag bulk formulations while their respective NPs have increased the protein content. The trend was observed to reverse for the Ti element with higher protein content in bulk over the respective NP amendments. Apart from the total protein content, the extracellular laccase enzyme activity was also observed to be affected by the bulk and NP supplementations of the three elements. The laccase activity was observed to be increased by supplementation of Ti and Ag bulk formulations in the test mushroom cultures while a numerical increase in nano Si over the bulk Si supplementation was recorded. The application of heavy metal in ionic forms derived from their soluble salts has been reported to enhance laccase formation/activity [Citation10,Citation21,Citation22]. Contrary to our results, Galhaup and Haltrich [Citation23] have reported Ag, Cd, Hg and Zn metals to be ineffective in enhancing laccase formation in Trametes pubescens. Copper, for instance, has been reported to enhance the laccase production. The solid-state fermentation experiments by Tychanowicz et al. [Citation24] exhibited improvement in the laccase production by Pleurotus pulmonarius on copper supplementation. Similarly, P. ostreatus laccase activity was observed to be increased by the addition of 1–5 mM Cd [Citation25]. The positive impact of the NPs for enhancement in the laccase activity has been documented [Citation26]. They showed both synthesis and stabilization or capping of gold nanoparticles by laccase enzyme. Therefore, a significant increase in the laccase activity was reported due to formation of the laccase-Au nanoparticle nanobioconjugate in presence of visible light [Citation26]. The heavy metal/NP amendment stress may also result in formation of novel proteins. Few protein bands appeared in the SDS-PAGE analysis of our study may be related to the formation of peroxidase enzyme complexes which are the key enzymes involved in combating the heavy metal and other abiotic stress management in eukaryotes [Citation22].
Nanomaterials particularly metal/metal oxide/non-metal oxide nanoparticles are increasingly being used as active ingredients of several cosmetics and other products. After use, the NPs may contaminate the soil and water resources which may be detrimental to the native soil bacteria and fungi. As mushrooms are the fungal genera having important role in decomposition and nutrient cycling, therefore, the concentrations of M/MO/NMO NPs detrimental to this indicator group must be deduced. The three NP supplementations resulted in numerical decrease in radial diameter of fungal colony in presence of TiO2 and Ag NPs at 100 mg L−1 while the radial growth increased by supplementation of silica NPs at 25 and 100 mg L−1 concentrations. The NP amendment also resulted in formation of new proteins which appeared as novel bands on SDS-PAGE of the crude protein extract of the G. lucidum and V. volvaceae strains.
Supplemental Material
Download MS Word (28.1 KB)Supplemental Material
Download MS Word (12.1 KB)Supplemental Material
Download Zip (155 MB)Acknowledgements
The authors acknowledge that this research work has been supported by the projects– ICAR-153 (PC2307), and UHK VT2019-2021.
Disclosure statement
No potential conflict of interest was reported by the author(s).
Additional information
Funding
References
- Khan I, Saeed K, Khan I. Nanoparticles: properties, applications and toxicities. Arabian J Chem. 2019;12(7):908–931.
- Tratnyek PG, Johnson RL. Nanotechnologies for environmental cleanup. Nanotoday. 2006;1(2):44–48.
- Inshakova E, Inshakov O. World market for nanomaterials: structure and trends. MATEC Web Conf ICMTMTE. 2017;129:02013.
- Jeevanandam J, Barhoum A, Chan YS, et al. Review on nanoparticles and nanostructured materials: history, sources, toxicity and regulations. Beilstein J Nanotechnol. 2018;9:1050–1074.
- Maynard AD, Aitken RJ, Butz T, et al. Safe handling of nanotechnology. Nature. 2006;444(7117):267–269.
- Huan C, Shu-Qing S. Silicon nanoparticles: preparation, properties, and applications. Physical Biol. 2015;46(39):no–14.
- Hashimoto K, Irie H, Fujishima A. TiO2 photocatalysis: a historical overview and future prospects. AAPPS Bull. 2007;17(6):12–28.
- Fujioka K, Hiruoka M, Sato K, et al. Luminescent passive-oxidized silicon quantum dots as biological staining labels and their cytotoxicity effects at high concentration. Nanotechnol. 2008;19(41):1–7.
- Wiesner MR, Lowry GV, Alvarez P, et al. Assessing the risks of manufactured nanomaterials. Environ Sci Technol. 2006;40(14):4336–4345.
- Baldrian P. Wood-inhabiting ligninolytic basidiomycetes in soils: ecology and constraints for applicability in bioremediation. Fungal Ecol. 2008;1(1):4–12.
- Gianfreda L, Xu F, Bollag JM. Laccases: a useful group of oxidoreductive enzymes. Bioremediation J. 1999;3(1):1–26.
- Baldrian P. Effect of heavy metals on saprotrophic soil fungi. In: Sherameti I, Varma A, editors. Soil heavy metals. Berlin (Heidelberg): Springer-Verlag 2010. p. 263–279.
- Amdekar S. Ganoderma lucidum (Reishi): source of pharmacologically active compounds. Curr Sci. 2016;111(6):976–978.
- Bozzola JJ, Russell LD. Electron microscopy: principles and techniques for biologists. Boston; Jones & Bartlett; 1999.
- Lowry OH, Rosebrough NJ, Farr AL, et al. Protein measurement with the Folin phenol reagent. J Biol Chem. 1951;193(1):265–275.
- Laemmli UK, Molbert E, Showe M, et al. Form-determining function of the genes required for the assembly of the head of bacteriophage T4. J Mol Biol. 1970;49(1):99–113.
- Turner EM. Phenoloxidase activity in relation to substrate and developmental stage in mushroom Agaricus biosporus. Trans Br Mycol Sociol. 1974;63(3):541–547.
- Singh RP, Garcha HS, Khanna PK. Laccase production by Pleurotus spp. Indian J Microbiol. 1988;28:38–41.
- Dhaliwal MS, Jindal SK, Dhaliwal LK, et al. Growth and yield of tomato influenced by condition of culture, mulch and planting date. Intl J Veg Sci. 2017;23(1):4–17.
- Goyal A, Kalia A, Sodhi HS. Selenium stress in Ganoderma lucidum: a scanning electron microscopy appraisal. Afr J Microbiol Res. 2015;9(12):855–862.
- Baldrian P. Fungal laccases – occurrence and properties. FEMS Microbiol Rev. 2006;30(2):215–242.
- Baldrian P. Interactions of heavy metals with white-rot fungi. Enzyme Microb Technol. 2003;32(1):78–91.
- Galhaup C, Haltrich D. Enhanced formation of laccase activity by the white-rot fungus Trametes pubescens in the presence of copper. Appl Microbiol Biotechnol. 2001;56(1–2):225–232.
- Tychanowicz GK, de Souza DF, Souza CGM, et al. Copper improves the production of laccase by the white-rot fungus Pleurotus pulmonarius in solid state fermentation. Braz Arch Biol Technol. 2006;49(5):699–704.
- Baldrian P, Gabriel J. Copper and cadmium increase laccase activity in Pleurotus ostreatus. FEMS Microbiol Lett. 2002;206(1):69–74.
- Guo S, Li H, Yang J, et al. Visible-light-induced effects of Au nanoparticle on laccase catalytic activity. ACS Appl Mater Interfaces. 2015;7(37):20937–20944.