Abstract
The strains 17E-042, 17E-039, and NC13-171 belong to Ascomycota and were isolated from soil collected from Sancheong-gun and Yeongam-gun, Korea. The strain 17E-042 produced white mycelial colonies that developed a sienna color with a round margin on potato dextrose agar (PDA), and the reverse side developed a light sienna color. Morphologically, this strain was similar to the strains of Arthrinium phragmites and A. hydei, but the shorter conidial size of the newly identified strain (17E-042) was distinct. The strain 17E-039 produced macroconidia that were pale yellow to orange-brown, elongated-ellipsoid to oblong, round at both ends, primarily straight but sometimes slightly curved, 0-septate, thin-walled, and filled with numerous droplets, having diameters of 20.4–34.3 × 8.0–12.0 μm. And the strain NC13-171 formed hyaline to light brown chlamydospores, solitary or in a chain. Multigene phylogenetic analyses were conducted using sequence data obtained from internal transcribed spacer (ITS) regions, 28S rDNA large subunit (LSU), β-tubulin (TUB2), translation elongation factor 1-alpha (TEF1-α), and RNA polymerase II large subunit (RPB2) genes. The results of molecular phylogeny, the detailed descriptions and illustrations of each species strongly support our proposal that these strains from soil in Korea be designated as Arthrinium minutisporum sp. nov. and two new records of Pezicula neosporulosa and Acrocalymma pterocarpi.
1. Introduction
The genus Arthrinium (sexual morph Apiospora) is widespread and ecologically diverse; some species were associated with plants as endophytes or saprobes, and some were pathogenic to plants, such as ornamentals [Citation1,Citation2]. Currently, there were more than 80 recognized species in Arthrinium [Citation3]. Notably, Arthrinium species show a distinct preference for growing on two graminaceous families, Poaceae and Cyperaceae. Bambusa (Poaceae) and Cwerex (Cyperaceae) were two of the most common host genera for Arthrinium species [Citation4]. The asexual morph of Arthrinium species can be easily recognized by its dark, aseptate, lenticular conidia with a hyaline rim or germ slit [Citation5]. Because of their relatively conserved morphology, the identification of Arthrinium to the species level is difficult when only the asexual morph is available. The Arthrinium species can produce hyphomyceteous fruiting bodies in culture or coelomycetous structures on host substrates [Citation6]. A detailed reevaluation of Arthrinium based on multigenic data added eight new species and presented genetic data from several other taxa strains [Citation7]. Therefore, molecular data and phylogenetic analysis have been used to classify Arthrinium species, allowing closely related taxa to be differentiated [Citation6,Citation7].
Genus Pezicula Tul. and C. Tul. (Leotiomycetes, Helotiales, Dermateaceae) was introduced as a widespread Discomycetes with the type strain Peziza carpinea and the members of Pezicula were characterized by their brightly colored apothecia, which occur on the bark of woody plants [Citation8]. Verkley monographed Pezicula and resolved the taxonomy of the common species P. cinnamomea, which includes P. livida and P. eucrita [Citation9]. He also introduced a new species, P. sporulosa which was morphologically distinct from the two other species [Citation9]. Subsequently, two new species and nine new combinations have been added to the genus [Citation10]. The phylogeny of these genera using sequence data from internal transcribed spacer (ITS) sequences, which provided the foundation for later studies on this generic complex [Citation11]. In 1912, Cryptosporiopsis scutellata was introduced as the asexual morph; the sexual morph is Pezicula ocellata. Since then, many species have been described under Cryptosporiopsis and Pezicula [Citation12–14].
The genus Acrocalymma belongs to the Dothideomycetes class, which was introduced as a pathogen that causes reddening of the internal root and crown tissue of Lucerne (Medicago sativa) in Australia [Citation15,Citation16]. The genus Rhizopycnis is nestled among Acrocalymma species and is reduced to synonymy based on its morphology and phylogeny of DNA. Acrocalymmaceae was introduced into the Dothideomycetes class as a new family to accommodate members of this group. The family Acrocalymmaceae was also named after the genus Acrocalymma [Citation17]. Acrocalymma represents an undefined lineage of Dothideomycetes that has Massarina-like sexual morphs in culture, establishing asexual or sexual propagation [Citation18]. At present, seven species have been identified in different countries from diverse sources and habitats under the family of Acrocalymmaceae: A. aquatica, A. cycadis, A. fici, A. medicaginis, A. pterocarpi, A. vagum, and A. walker [Citation19]. Among these seven species, two were identified based on their sexual morph: A. pterocarpi and A. walker from Thailand and Australia, respectively [Citation17,Citation19]. A. medicaginis was described as a sexual morph of Massarina walkeri [Citation18]. Although some species were identified in different countries, there has not been a sexual or asexual morph recorded under this genus in Korea until now.
The aim of the overall study from which this report has emerged is to investigate the diversity of native fungal species in Korea based on cultural and morphological characteristics along with their molecular phylogenetic relationships. These previously undescribed fungal species of Ascomycetes were identified during this study.
2. Material and methods
2.1. Soil sample collection and fungal isolation
In 2017, the fungal candidates were obtained from soil samples collected from Sancheong-gun (35°20′15.0″N, 127°47′32.4″E), and Yeongam-gun (34°46′14.9″N, 126°39′21.1″E) in Korea. The soil samples were collected by using pre-autoclaved sterile spatula and shovel to randomly select composite soil samples and then removed the waste materials and debris. The collected soil was thoroughly mixed, transferred immediately into sterile plastic bags, and then transported to the laboratory. A serial dilution was performed, and 0.1 mL of sample plated on potato dextrose agar (PDA; Difco, Detroit, MI, USA) plates [Citation20]. To avoid bacterial contamination, ampicillin 100 µg/mL was added to the media before pouring into Petri dishes. The plates were incubated at 25 °C for 3 or 4 days. Single colonies were transferred to fresh PDA plates and incubated at 25 °C to obtain pure cultures. The fungal candidates were selected from among multiple candidates based on different cultural and morphological characteristics for further molecular analyses. Each pure fungal candidate was stored in 20% glycerol at −80 °C until further use.
2.2. Morphological characterizations
Cultural characteristics and morphological observations were performed with different media depending on the genus and species. The strains 17E-042 and 17E-039 were transferred onto potato dextrose agar (PDA), oatmeal agar (OA), or malt extract agar (MEA) and incubated at 25 °C temperature for 7 days [Citation7,Citation10]. Similar cultures of the strain 17E-039 were also incubated for 14–21 days under near-ultraviolet light [Citation10]. The strain NC13-171 was sub-cultured on PDA, OA, and MEA at 25 °C for 14 days [Citation17,Citation19]. Colony characteristics were recorded, and the mycological characteristics were observed by light microscopy (BX-50; Olympus, Tokyo, Japan).
2.3. Genomic DNA extraction, PCR amplification, and DNA sequencing
For molecular identification, the genomic DNA of each fungal strain was extracted using a HiGene Genomic DNA prep kit (BIOFACT, Daejeon, Korea), according to the manufacturer’s instructions. The ITS regions of rDNA genes were amplified using the primers ITS1 and ITS4 [Citation21]. For 17E-042, a portion of the translation elongation factor 1-alpha (TEF1-α) gene was amplified and sequenced using primers EF1-728F [Citation22] and EF-2 [Citation23]. A portion of the β-tubulin (TUB2) gene was also amplified using primers T1 [Citation24] and Bt2b [Citation25]. In 17E-039, primers fRPB2-5F2 and fRPB2-7cR [Citation26] were used to amplify RNA polymerase II large subunit (RPB2) along with ITS regions [Citation27] and the partial 28S rDNA large subunit (LSU) gene using primer pairs LROR and LR5 [Citation28]. In NC13-171, a portion of the nuclear 28S rDNA was also amplified using the primer pairs LROR and LR5 [Citation28] along with the ITS regions. PCR amplicons were sequenced using the same primers as were used for the amplification reactions, purified with EXOSAP-IT (Thermo Fisher Scientific, Waltham, MA, USA), and sequenced by Solgent (Daejeon, Korea). SeqMan Lasergene software (DNAStar Inc., Madison, WI, USA.) was used to align the sequence data obtained in this study.
2.4. Phylogenetic analysis
The sequences generated in this study were supplemented with additional sequences obtained from the National Center for Biotechnology Information (NCBI) (). The sequences were selected from NCBI using BLAST searches to identify members closest to the strains isolated in this study. The evolutionary distance matrices for the neighbor-joining (NJ) algorithm were calculated using Kimura’s two-parameter model [Citation29]. Phylogenetic relationships were also inferred by tree topology using maximum-likelihood (ML), and maximum-parsimony (MP) methods with MEGA7.0 software with bootstrap values based on 1000 replications [Citation30].
Table 1. List of species used in this study and their GenBank accession numbers for phylogenetic analysis.
3. Results
3.1. Taxonomical analysis of Arthrinium minutisporum 17E-042
3.1.1. Taxonomy
The collected strain 17E-042 exhibited distinct morphology compared with other allied species. Therefore, it is described as a new species.
Arthrinium minutisporum K. Das, S.Y. Lee and H.Y. Jung, sp. nov. ()
Figure 1. Cultural and morphological characteristics of 17E-042. (A) Colonies were grown on potato dextrose agar; (B) Malt extract agar; (C) Oatmeal agar at 25 °C for 7 days, reverse and obverse side view, respectively. (D) Colonies on oatmeal agar; (E–G) Conidiogenous cells give rise to conidia; (H–J) Sterile cells; (K–L) Conidia. Scale bars: E–L = 10 μm.
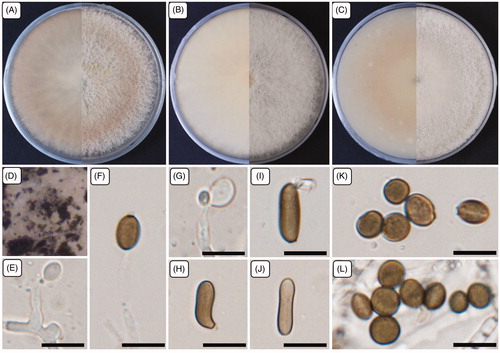
MycoBank: MB835039
Etymology: The specific epithet “minutisporum” refers to the small size of the conidia.
Typus: Sancheong-gun, Korea (35°20′15.0″N, 127°47′32.4″E), isolated from mountain soil. The stock culture (ZEVCFG0000000088 = KCTC 56425) was deposited in the National Institute of Biological Resources (NIBR) and Korean Collection for Type Cultures (KCTC) as a metabolically inactive culture.
Specimen examined: Sancheong-gun, Korea, isolated from mountain soil, deposited in NIBR Oct. 2017, H.Y. Jung, (holotype ZEVCFG0000000088, dried and living culture, ex-holotype living culture KCTC 56425).
Ecology and distribution: Several members of this genus have been recorded from different hosts as endophytes and as pathogens to plants, such as barley, wheat, lichens, and humans. However, there are so many members of this genus were also associated with the hosts and plant species in many countries such as Hordeum vulgare (Iran); Phragmites australis (Harderbos); leaf of bamboo, Camellia sinensis, Brassica capestris, Phragmites australis, air in karst cave (China); gut of a grasshopper, Jatropha podagrica (India); Carex despalata (Japan); plant species of Restionaceae family (South Africa); Bambusa sp. (Bangladesh); air and maritime sand (Spain); culms of Bambusa tuldoides, Arundinaria hindsii (Hong Kong); and also from leaf and culms of bamboo (The Netherlands, Thailand). Whereas, the present studied strain 17E-042 was collected from mountain soil in Korea. The soil contained plant debris, was yellowish brown, and had a low moisture capacity.
Cultural characteristics: Mycelia were superficial and immersed in media, with branched, septate, smooth hyphae. The colonies were flat, spreading with profuse aerial mycelium when cultured at 25 °C for 7 days. The colonies were initially white and then developed a sienna color with a round margin on PDA, and the reverse side displayed a light sienna color. On MEA, the colonies were white with a light brown color on the reverse side. On OA, colonies produced a light sienna color in the center. At higher magnification on OA, colonies were dirty white with patches of olivaceous-gray. The growth extent of colonies is similar in all media tested: 79.0–84.0, 80.0–84.0, and 80.0–83.0 mm on PDA, OA, and MEA, respectively. Colonies also develop a surrounding light-yellow pigment with age on PDA.
Morphological characteristics: The micromorphological structures were studied by culturing on PDA. Mycelia were produced profusely and were hyaline, branched, smooth, septate, and 1.8–4.0 µm wide. Conidiophores were reduced to conidiogenous cells. The conidiogenous cells were erect, ellipsoid to ovoid, hyaline, pale brown to umber in color, and smooth. Diameter (length/width) ranges were 5.0–7.5 × 3.6–6.0 µm with an average diameter of 6.0 × 5.0 µm. The strain also forms sterile cells that were solitary or sometimes aggregated with the conidia, light brown to brown in color, finely roughened, sometimes curved or shrunken in the middle or at the edge of cells, and ellipsoid to subcylindrical. Diameter ranges (n = 10) were 10.2–14.2 × 3.8–4.8 µm with an average diameter of 12.1 × 4.3 µm. The strain produced numerous conidia that were brown, smooth to finely roughened, ellipsoidal to ovoid, thick-walled, solitary to aggregated, with irregular dot-like structures inside the conidia. Diameter ranges (n = 100) were 5.7–8.2 × 4.6–7.0 µm with an average diameter of 6.7 × 6.1 µm.
Note: The strain 17E-042 produced white colonies that developed a sienna color with a round margin on PDA; the reverse side displayed a light sienna color. However, Arthrinium phragmites displayed a dirty white color with pale luteous patches, and the reverse side was luteous. The colonies of A. hydei also produced pale luteous colonies on PDA (). The strain 17E-042 produced smaller conidiogenous cells with diameters of 5.0–7.5 × 3.6–6.0 µm. However, A. phragmites produced conidiogenous cells with diameters of 12.0–15.0 × 3.0–5.0 µm, A. hydei also produced conidiogenous cells with the diameters of 5.0–8.0 × 4.0–5.0 µm, and A. aureum was described with the integrated, polyblastic, and denticulate conidiogenous cells. The conidia of 17E-042 were also smaller (5.7–8.2 × 4.6–7.0 µm) than those of A. phragmites (9.0–10.0(–12.0) × (5.0–)6.0(–7.0) µm), A. aureum (10.0 − 30.0 × 10.0 − 15.0) µm and A. hydei (17.0(15.0–) − 19.0(22.0–) × 11.0(10.0–) − 12.0(14.0–)) µm (). Sterile cells of A. phragmites were solitary loci on hyphae, brown, finely roughened, ellipsoid to clavate, with diameters of 13.0–15.0(–17.0) × (5.0–)6.0 µm. However, the strain 17E-042 produced sterile solitary cells that were aggregated with the conidia, light brown to brown, sometimes curved or shrunken in the middle or at the edge of cells, ellipsoid to subcylindrical, with diameters (n = 10) of 10.2–14.2 × 3.8–4.8 µm (). Therefore, the cultural and morphological characteristics supported that the strain 17E-042 is distinct from previously known species of Arthrinium.
Table 2. Morphological comparison, habitat, and origin of Arthrinium minutisporum sp. nov. with the closest species of Arthrinium.
3.1.2. Phylogenetic analysis of the strain 17E-042
The BLAST search results of ITS regions sequences from the NCBI database reflected similarities between 17E-042 and Arthrinium hydei G1863 (96%), A. phaeospermum N.SBA34 (95%), and A. aureum CBS 244.83T (96%). The TEF1-α gene revealed similarities with A. camelliae-sinensis LC8181 (90%), A. hydei CBS 114990T (89%), A. aureum CBS 244.83T (88%), and A. phaeospermum CBS 114315 (85%). The TUB2 gene reflected similarities with A. rasikravindrii LC7129 (90%), A. phaeospermum MAFF 410785 (89%), A. hydei LC7103 (88%), and A. aureum CBS 244.83T (87%). A combination of ITS regions, TEF1-α, and TUB2 genes was used to perform the phylogenetic analysis. Three phylogenetic trees were constructed using NJ, ML, and MP statistical method from concatenated sequences and the results were same. The ML and MP method results were also indicated in NJ tree with open and filled circles. Filled circles indicated that the corresponding nodes were recovered in trees generated with both the ML and MP algorithms. The tree positions generated with either the ML or MP algorithm were indicated with open circles (). The boundaries within the Arthrinium were defined by a combined alignment of ITS regions, TEF1-α, and TUB2 sequences to clarify the species that exhibited the highest sequence similarities and these boundaries contained 29 strains. The phylogenetic analysis was also conducted based on a combination of the sequences with MP (tree length = 1161, consistency index = 0.55, retention index = 0.71, and composite index = 0.43) to determine the exact taxonomic position of the strain. The phylogenetic tree showed that the phylogenetic position of the strain 17E-042 is distinct from the other identified species of Arthrinium (). Consequently, the strain 17E-042 is proposed as a new species in the fungal flora under the genus of Arthrinium.
Figure 2. Neighbor-joining phylogenetic tree of 17E-042 based on combined sequences data (ITS+TUB2+TEF1) showing the relationship between Arthrinium minutisporum sp. nov., and the closest Arthrinium spp. The tree was rooted using Seiridium phylicae CPC 19965 as an outgroup. The numbers above the branches represent the bootstrap values (ML/MP/NJ) obtained for 1000 replicates (values smaller than 50% were not shown). The isolated strain of this study is indicated in bold. Bar, 0.02 substitutions per nucleotide position.
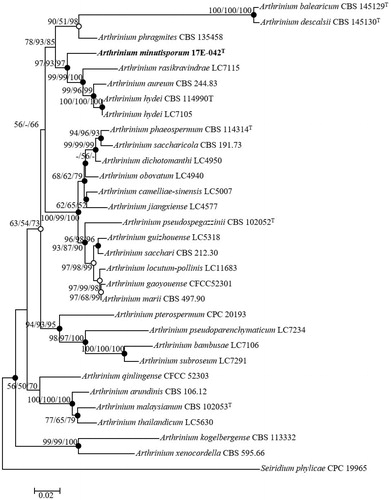
3.2. Taxonomical analysis of Pezicula neosporulosa 17E-039
Pezicula neosporulosa Yuan Z and Verkley GJM, Mycoscience 56: 205–213 (2015) ()
Figure 3. Cultural and morphological characteristics of 17E-039. (A) Colonies were grown on potato dextrose agar; (B) Malt extract agar; (C) Oatmeal agar at 25 °C for 14 days, reverse and obverse side view, respectively. (D–F) Conidiomata forming on oatmeal agar; (G) Macroconidiogenous cells; (H) Microconidiogenous cells; (I–K) Macroconidia; (L, M) Microconidia. Arrows indicate microconidiogenous cells. Scale bars: D–F = 500 μm; G–M = 10 μm.
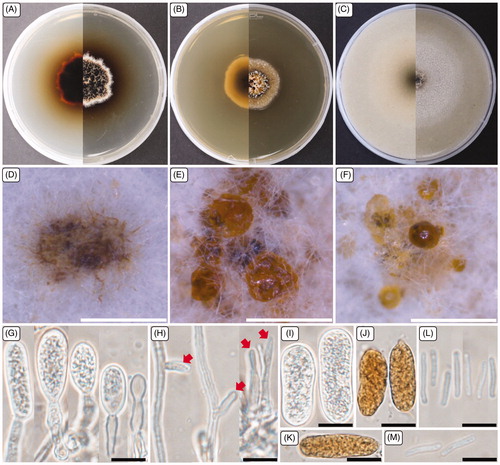
3.2.1. Taxonomy
The strain 17E-039 was isolated from mountain soil in Sancheong-gun, Korea (35°20′15.0″N, 127°47′32.4″E) exhibited the cultural and morphological characteristics () that were similar to the identified Pezicula neosporulosa, which has not been reported in Korea previously.
Table 3. Morphological characteristics of the strain 17E-039 with reference to Pezicula neosporulosa.
Specimen examined: Sancheong-gun, Korea (35°20′15.0″N, 127°47′32.4″E), isolated from mountain soil, deposited in NIBR July, 2017, H.Y. Jung, (NIBRFG0000501934, dried and living culture, National Institute of Biological Resources).
Ecology and distribution: The members of the genus Pezicula reported previously from temperate regions, where they occur as endophytes or as saprobes on recently dead branches, twigs, and herbaceous plants such as wood bark in Northern Thailand. They were also the causal agent of bull’s-eye rot on Japanese pears, even responsible for stem canker disease on apples and pears. Moreover, there are so many members of this genus were also associated with the plant species in many countries such as Acer spicatum, Gaultheria shallon (Canada), Eucalyptus sp. (USA), Carpinus betulus (Netherlands, Germany), Abies balsamea (Norway), Fagus sylvatica (France), and also from Juniperus communis (Switzerland). Whereas, the strain 17E-039 was isolated from mountain soil in Korea. The soil contained plant debris, was dark brown to yellowish brown, and had a low moisture capacity.
Cultural characteristics: The colonies on PDA were flat, white to brown, with aerial mycelium, and dark brown pigment developed on the agar media; the colonies extended to 29.0–32.0 mm in diameter when cultured at 25 °C for 14 days. On the reverse side, colonies were brown to black, with a dark yellow margin at the edge. Colonies on MEA were flat, white to pale brown, with submerged mycelium, pale yellow to dark yellow; the colonies extended to 28.0–30.0 mm diameter. On the reverse side, colonies were white at the edge and brown in the center. Colonies grow faster on OA than on other media, reaching 44.0–69.0 mm diameter under identical incubation conditions. Colonies were flat and white, and on the reverse side, colonies were pale brown to brown in the center.
Morphological characteristics: The asexual morph was studied in vitro on OA media to describe the morphology. Conidiomata were produced profusely on the agar surface, globose to sub-globose, initially closed with white to gray hyphae. The structures then became disc-like with a diameter range of 0.5–1.2 mm; these structures produced water droplets, with white to pale yellow conidial slime that contained micro- and macroconidia. Macroconidiogenous cells were hyaline, flask-shaped to cylindrical, simple, or branched. The macroconidiogenous cells proliferate percurrently, achieving a diameter (length/width) range of 7.2–23.1 × 4.5–9.1 µm, with an average diameter of 16.4 × 7.3 µm. Microconidiogenous cells were hyaline, phialidic, with a minute periclinal thickening, having a diameter range of 7.5–14.7 × 1.5–2.5 µm with an average diameter of 10.2 × 2.1 µm. Macroconidia were pale yellow to orange-brown, elongated-ellipsoid to oblong, round at both ends, primarily straight but sometimes slightly curved, 0-septate, thin-walled, and filled with numerous droplets, having diameters (n = 50) of 20.4–34.3 × 8.0–12.0 µm with an average diameter of 26.5 × 10.0 µm. Microconidia were cylindrical, straight, sometimes slightly bent but typically wider in the middle than near the rounded top, base truncate, hyaline, thin-walled, having diameters (n = 30) of 7.6–15.0 × 1.2–1.7 µm with an average diameter of 12.0 × 1.4 µm.
3.2.2. Phylogenetic analysis of strain 17E-039
Nucleotide sequences data encompassing 536 bp of the ITS regions, 830 bp of 28S rDNA, and 912 bp of RPB2 were obtained to investigate the evolutionary relationships between the strain 17E-039 and previously described species of Pezicula neosporulosa. ITS sequences from BLAST search results revealed the maximum similarity (99.81%) with the different strains of P. neosporulosa (CBS 101.96T, CBS 724.95, CBS 636.96, CBS 102.96, CBS 100416). The 28S rDNA displayed the maximum similarity (100%) with the strains of P. neosporulosa (CBS 101.96T, CBS 635.96, CBS 100416) and also showed high similarity (99.88%) with the strains of P. neosporulosa (CBS 636.96, CBS 660.95, CBS 724.95, CBS 102.96). The highest similarities with RPB2 displayed with P. eucrita CBS 259.97 (98.53%), P. sporulosa CBS 225.96 (98.53%), P. sporulosa CBS 224.96T (98.42%), and P. cinnamomea CBS 626.96 (97.97%). A phylogenetic analysis was conducted based on a combination of the ITS regions, partial sequences of the 28S rDNA, and RPB2 genes by using the NJ method (). The taxonomic position of the strain 17E-039 was same in each three phylogenetic tree methods. Filled circles indicated that the corresponding nodes were also recovered in trees generated with both the MP and MP algorithms. The tree positions generated with either the ML or MP algorithm were indicated with open circles (). Phylogenetic analyses using the NJ method revealed that the strain 17E-039 clustered with the previously identified strain P. neosporulosa CBS 101.96T (). Consequently, the strain 17E-039 is identified as Pezicula neosporulosa, a previously undescribed fungal species in Korea.
Figure 4. Neighbor-joining phylogenetic tree of 17E-039 based on combined sequences data (ITS + LSU+RPB2) showing the relationships between P. neosporulosa and the closest Pezicula spp. The tree was rooted using Rhizodermea veluwensis CBS 110605T as an outgroup. The numbers above the branches represent the bootstrap values (ML/MP/NJ) obtained for 1000 replicates (values smaller than 60% were not shown). The isolated strain of this study is indicated in bold. Bar, 0.01 substitutions per nucleotide position.
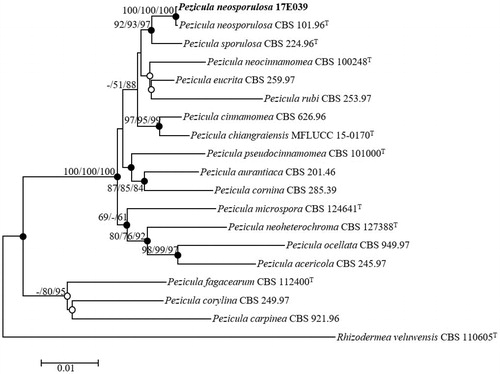
3.3. Taxonomical analysis of Acrocalymma pterocarpi NC13-171
Acrocalymma pterocarpi Jayasiri SC, Jones EBG and Hyde KD, Mycosphere 10 (1): 20 (2019) ()
Figure 5. Cultural and morphological characteristics of NC13-171. (A) Colony on potato dextrose agar; (B) Malt extract agar; (C) Oatmeal agar at 25 °C in 14 days, reverse and obverse side view, respectively. (D) Structures formed in culture; (E–G) Chlamydospores. Scale bars: D = 500 μm; E–G = 10 μm.
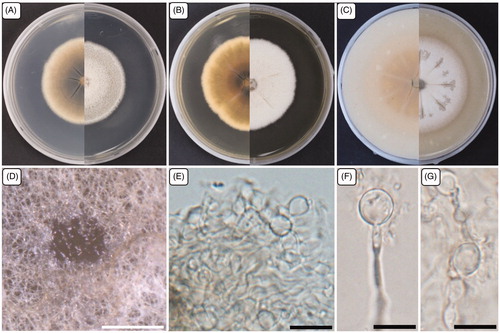
3.3.1. Taxonomy
The strain NC13-171 was isolated from field soil in Yeongam-gun (34°46′14.9″N, 126°39′21.1″E) exhibited similar cultural and morphological characteristics to the identified Acrocalymma pterocarpi, which has not been reported in Korea previously.
Specimen examined: Yeongam-gun, Korea (34°46′14.9″N, 126°39′21.1″E), isolated from field soil, deposited in NIBR May, 2017, H.Y. Jung, (ZEVCLC0000000002, dried and living culture, in the National Institute of Biological Resources).
Ecology and distribution: The member of the genus Acrocalymma associated with a root and crown rot disease of lucerne (Medicago sativa), found from freshwater in Thailand, leaves of Cycas calcicola in Australia, on Medicago sativa from Australia, and also from Pterocarpus indicus seed pod in Thailand. Whereas, the present studied strain NC13-171 was collected from field soil in Korea. The soil contained plant debris, was dark brown to blackish, and had a medium moisture capacity.
Cultural and morphological characteristics of the strain NC13-171
The mycelial colonies were white to pale gray, circular, and dense, with a smooth edge. On the reverse side, colonies were light brown to dark brown in the center on PDA. The colonies were circular, dense, white, rough in the middle with a smooth edge, and slightly undulate. On the reverse side, colonies were slightly yellow on MEA and OA. Fungal growth was circular with diameters of 42.0–51.0, 48.0–52.0, and 55.0–58.0 mm on PDA, MEA, and OA, respectively, when incubated at 25 °C for 14 days. The strain NC13-171 developed structures on the MEA surface after 4–5 weeks of incubation at 25 °C. The strain NC13-171 also produced compact chlamydospores, solitary or in a chain, and hyaline to light brown. Previous studies reported that the only species with a teleomorph is Acrocalymma walker, while the other five species were coelomycetous [Citation18]. Recently, another strain identified as a teleomorph of A. pterocarpi was isolated from a decaying Pterocarpus indicus seed pod in Thailand. However, A. pterocarpi failed to produce anamorphic structures in culture, and only chlamydospores were observed [Citation19]. The strain NC13-171 was isolated from the soil and cultured on different media to assess the cultural and morphological characteristics. However, NC13-171 failed to develop teleomorphic structures in culture but produced anamorphic structures, such as chlamydospores, like those of A. pterocarpi. Therefore, the cultural and morphological characteristics supported that the strain NC13-171 was Acrocalymma pterocarpi, and is reported in Korea for the first time.
3.3.2. Phylogenetic analysis of strain NC13-171
Nucleotide sequences data encompassing 489 bp of the ITS regions and 837 bp of the 28S rDNA were obtained to determine the evolutionary relationships between the strain NC13-171 and related strains obtained from GenBank (). The BLAST results from the ITS regions sequence data showed 99.16% similarity with the recently identified type strain Acrocalymma pterocarpi (MFLUCC 17-0926T) from Thailand. The 28S rDNA sequences revealed the maximum similarity (99.76%) with the type strain A. pterocarpi (MFLUCC 17-0926T). The phylogenetic tree was constructed using NJ method based on the combination of ITS regions and the partial sequences of 28S rDNA. NC13-171 was clustered together with the previously identified A. pterocarpi (MFLUCC 17-0926T) with strong bootstrap values (99%). The taxonomic position of the strain NC13-171 was same in each three-NJ, ML, and MP statistical method from concatenated sequences. Filled circles indicated that the corresponding nodes were recovered in trees generated with both the ML and MP algorithms. Tree positions generated with open circles either the ML or MP methods in the MP algorithm (). The phylogenetic results strongly supported the identification of NC13-171 as Acrocalymma pterocarpi. To our best knowledge, this is the first report of Acrocalymma pterocarpi isolated from soil in Korea.
Figure 6. Neighbor-joining phylogenetic tree based on the concatenated ITS region and partial of 28S rDNA sequences showing the phylogenetic position of Acrocalymma pterocarpi NC13-171 among members of the genus Acrocalymma. The tree was rooted using Boeremia exigua CBS 431.74 as an outgroup. The numbers above the branches represent the bootstrap values (ML/MP/NJ) obtained for 1000 replicates (values smaller than 50% were not shown). The isolated strain of this study is indicated in bold. Bar, 0.01 substitutions per nucleotide position.
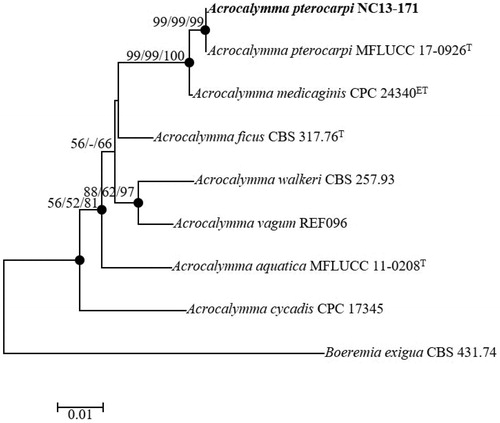
4. Discussion
The morphologically distinct strain 17E-042 and two unreported strains, 17E-039 and NC13-171, were obtained in 2017 from the soil at Sancheong-gun and Yeongam-gun in Korea, respectively. The strains exhibited morphological differences and similarities with the identified and closely related species; these findings were supported by the descriptions of closely related species in previously published reports.
Arthrinium has been reported as a plant pathogen. A. arundinis causes kernel blight in barley [Citation31], and A. sacchari causes damping-off of wheat [Citation32]. Arthrinium is also an endophyte in plant tissues [Citation33], lichens [Citation34], and soils [Citation5] and can also cause infections in humans [Citation35]. Many Arthrinium species produce bioactive compounds with pharmacological and medicinal applications, such as A. arundinis and A. saccharicola isolated from a brown alga Sargassum sp. [Citation36]. A. saccharicola, A. sacchari and A. phaeospermum isolated from Miscanthus sp. produce industrially important enzymes [Citation37]. A. marii occurs most frequently on Poaceae grasses Ampelodesmos mauritanicus and Phragmites australis, and A. phragmitis is commonly found on Phragmites australis and less commonly on Arundo donax [Citation7]. Recently, six new species of Arthrinium have been proposed: A. balearicum, A. descalsii, A. esporlense, A. ibericum, A. italicum, and A. piptatheri. Five of these species were found in the Mediterranean biogeographical region; the exception was A. ibericum, which was found in the Atlantic areas of Spain associated with host plants of the Poaceae family, such as Arundo donax and Piptatherum miliaceum [Citation3]. Moreover, Arthrinium species were also isolated from soil in different places such as A. rasikravindrii (karst cave); A. arundinis (karst cave and roadway); A. xenocordella (roadway) [Citation4]. Even, some species were also found from soil in different countries including A. xenocordella (Zimbabwe), A. phaeospermum (Japan), A. sacchari (Netherland), and A. xenocordella (Austria) [Citation7]. Whereas, the newly identified strain 17E-042 proposed Arthrinium minutisporum was found in soil during a survey of native fungal diversity in Korea.
The members of the genus Pezicula were reported in predominantly temperate regions, where they occur as endophytes or as saprobes on recently dead branches, twigs, and herbaceous plants [Citation38,Citation39]. Recently, Pezicula chiangraiensis was isolated from wood bark in Chiang Rai Province, Northern Thailand [Citation27]. Many species of Pezicula were weak pathogens that cause disease when their hosts were stressed [Citation9]. The asexual morph of P. corticola was identified as the causal agent of bull’s-eye rot on Japanese pears. P. cinnamomea was isolated from stem canker disease on apples and pears [Citation40]. Pezicula species also have pharmaceutical importance by antibiotics such as mellein, mycorrhizin, cryptosporiopsin, cryptocandin, and cryptocin have been isolated from endophytic Pezicula and Cryptosporiopsis species [Citation41]. Moreover, P. neosporulosa was isolated from diversified host plants such as Amelanchier lamarckii, Abies alba, Larix decidua, Pseudotsuga menziesii from Netherlands. The member of Pezicula (Pezicula ocellata) was found from the plant species of Salix sp. in Germany, P. melanigena (asexual morph Cryptosporiopsis melanigena) from Quercus petraea in Austria, and P. microspora from an endophyte called Berberis vulgaris in Italy [Citation42]. From the previous studies, the members of the Pezicula species were hardly found from soil, whereas, the newly identified undescribed species called Pezicula neosporulosa was found in soil during the investigation of native fungal diversity in Korea.
The genus Acrocalymma was established for A. medicaginis, a species associated with a root and crown rot disease of lucerne (Medicago sativa) [Citation15]. Stagonospora meliloti is also responsible for lucerne crown and root rot disease and is prevalent in southern New South Wales [Citation16]. Both pathogens (A. medicaginis and Stagonospora meliloti) cause reddening of the internal root and crown tissue of lucerne, and they can be distinguished by the symptoms they induce [Citation43]. A. aquatica was isolated from freshwater in Thailand [Citation44], and A. cycadis was isolated from the leaves of Cycas calcicola in Australia [Citation45]. A. walkeri is the only species with a sexual morph found on Medicago sativa from Australia [Citation17]. Recently, another sexual morph of A. pterocarpi was identified from a Pterocarpus indicus seed pod in Thailand [Citation19]. There are different members of the genus Acrocalymma were isolated from submerged wood in a freshwater stream, leaf litter of Cycas calcicola, Ficus sp., Medicago sativa, Amaranthusm sp., Citrullus lanatus, Cucumis melo, C. sativus, Cucurbita rootstock, and also from Vitis vinifera in different countries [Citation19]. To date, there are seven species identified including some of their sexual morph from different countries with the diversified host plants, but there has not been a sexual or asexual morph recorded under this genus in Korea until now. Although members of the genus Acrocalymma occur in many habitats worldwide, but the identified strain NC13-171 was isolated from soil in Korea.
Microorganisms are receiving more attention in recent years because of their harmful effects, their important role in our ecosystems, and their production of compounds that are used in pharmaceuticals. In this study, the identification of the Arthrinium species, Pezicula neosporulosa, and Acrocalymma pterocarpi was reported: cultural, morphological characteristics, and molecular phylogenetic relationships. Further investigation is necessary to reveal the pharmaceutical importance and potential pathogenicity to the range of host species present in the ecological and environmental conditions of Korea. To our best knowledge, this is the first report of Pezicula neosporulosa and Acrocalymma pterocarpi in Korea, and Arthrinium minutisporum is proposed as a novel species.
Acknowledgements
The authors are grateful to the Ministry of Environment (MOE) of the Republic of Korea for the research on survey data and discovery of indigenous fungal species supported by a grant from the National Institute of Biological Resources (NIBR).
Disclosure statement
The authors declare that they have no potential conflicts of interest.
References
- Agut M, Calvo MA. In vitro conidial germination in Arthrinium aureum and Arthrinium phaeospermum. Mycopathologia. 2004;157:363–367.
- Li BJ, Liu PQ, Jiang Y, et al. First report of culm rot caused by Arthrinium phaeospermum on Phyllostachys viridis in China. Plant Dis. 2016;100:1013–1013.
- Pintos Á, Alvarado P, Planas J, et al. Six new species of Arthrinium from Europe and notes about A. caricicola and other species found in Cwerex spp. hosts. MycoKeys. 2019;49:15–48.
- Wang M, Tan XM, Liu F, et al. Eight new Arthrinium species from China. MycoKeys. 2018;34:1–24.
- Singh SM, Yadav LS, Singh PN, et al. Arthrinium rasikravindrii sp. nov. from Svalbard, Norway. Mycotaxon. 2012;122:449–460.
- Dai DQ, Phookamsak R, Wijayawardene NN, et al. Bambusicolous fungi. Fungal Divers. 2017;82:1–105.
- Crous PW, Groenewald JZ. A phylogenetic re-evaluation of Arthrinium. IMA Fungus. 2013;4:133–154.
- Ooki Y, Fujita T, Harada Y. Pezicula cinnamomea from cherry tree: pathogenicity tests and photomorphogenesis in culture. Mycoscience. 2003;44:319–326.
- Verkley GJM. A monograph of the genus Pezicula and its anamorphs. Stud Mycol. 1999;44:1–180.
- Yuan ZL, Verkley GJM. Pezicula neosporulosa sp. nov. (Helotiales, Ascomycota), an endophytic fungus associated with Abies spp. in China and Europe. Mycoscience. 2015;56:205–213.
- Abeln ECA, de Pagter MA, Verkley GJM. Phylogeny of Pezicula, Dermea and Neofabraea inferred from partial sequences of the nuclear ribosomal RNA gene cluster. Mycologia. 2000;92:685–693.
- Verkley GJM, Zijlstra JD, Summerbell RC, et al. Phylogeny and taxonomy of root-inhabiting Cryptosporiopsis species, and C. rhizophila sp. nov., a fungus inhabiting roots of several Ericaceae. Mycol Res. 2003;107:689–698.
- Cheewangkoon R, Groenewald JZ, Verkley GJM, et al. Re-evaluation of Cryptosporiopsis eucalypti and Cryptosporiopsis-like species occurring on Eucalyptus leaves. Fungal Divers. 2010;44:89–105.
- Zhu L, Wang X, Huang F, et al. A destructive new disease of Citrus in China caused by Cryptosporiopsis citricarpa sp. nov. Plant Dis. 2012;96:804–812.
- Alcorn JL, Irwin JAG. Acrocalymma medicaginis gen. et sp. nov. causing root and crown rot of Medicago sativa in Australia. Trans Br Mycol Soc. 1987;88:163–167.
- Irwin JAG. Stagonospora root and crown rot of lucerne. Austral Plant Pathol. 1972;1:29–30.
- Trakunyingcharoen T, Lombard L, Groenewald JZ, et al. Mycoparasitic species of Sphaerellopsis, and allied lichenicolous and other genera. IMA Fungus. 2014;5:391–414.
- Shoemaker RA, Babcock CE, Irwin JAG. Massarina walkeri n. sp., the teleomorph of Acrocalymma medicaginis from Medicago sativa contrasted with Leptosphaeria pratensis, L. weimeri n. sp., and L. viridella. Can J Bot. 1991;69:569–573.
- Jayasiri SC, Hyde KD, Jones EBG, et al. Diversity, morphology and molecular phylogeny of Dothideomycetes on decaying wild seed pods and fruits. Mycosphere. 2019;10:1–186.
- Paul NC, Mun HY, Lee HW, et al. A new record of Penicillium raphiae isolated from agricultural soil of Ulleung island, Korea. Mycobiology. 2014;42:282–285.
- White TJ, Bruns T, Lee S, et al. Amplification and direct sequencing of fungal ribosomal RNA genes for phylogenetics. In: Innis MA, Gelfand DH, Sninsky JJ, et al., editors. PCR protocols: a guide to methods and applications. New York (NY): Academic Press; 1990. p. 315–322.
- Carbone I, Kohn LM. A method for designing primer sets for speciation studies in filamentous ascomycetes. Mycologia. 1999;91:553–556.
- O'Donnell K, Kistler HC, Cigelnik E, et al. Multiple evolutionary origins of the fungus causing Panama disease of banana: concordant evidence from nuclear and mitochondrial gene genealogies. Proc Natl Acad Sci USA. 1998;95:2044–2049.
- O'Donnell K, Cigelnik E. Two divergent intragenomic rDNA ITS2 types within a monophyletic lineage of the fungus Fusarium were nonorthologous. Mol Phylogenet Evol. 1997;7:103–116.
- Glass NL, Donaldson G. Development of primer sets designed for use with PCR to amplify conserved genes from filamentous ascomycetes. Appl Environ Microbiol. 1995;61:1323–1330.
- Liu Y, Whelen S, Hall BD. Phylogenetic relationships among ascomycetes: evidence from an RNA polymerase II subunit. Mol Biol Evol. 1999;16:1799–1808.
- Ekanayaka AH, Daranagama DA, Ariyawansa HA, et al. Pezicula chiangraiensis sp. nov. from Thailand. Mycotaxon. 2016;131:739–748.
- Vilgalys R, Hester M. Rapid genetic identification and mapping of enzymatically amplified ribosomal DNA from several Cryptococcus species. J Bacteriol. 1990;172:4238–4246.
- Kimura M. A simple method for estimating evolutionary rates of base substitutions through comparative studies of nucleotide sequences. J Mol Evol. 1980;16:111–120.
- Kumar S, Stecher G, Tamura K. MEGA7: molecular evolutionary genetics analysis version 7.0 for bigger datasets. Mol Biol Evol. 2016;33:1870–1874.
- Martínez-Cano C, Grey WE, Sands DC. First report of Arthrinium arundinis causing kernel blight on barley. Plant Dis. 1992;76:1077B.
- Mavragani DC, Abdellatif L, McConkey B, et al. First report of damping-off of durum wheat caused by Arthrinium sacchari in the semi-arid Saskatchewan fields. Plant Dis. 2007;91:469.
- Ramos HP, Braun GH, Pupo MT, et al. Antimicrobial activity from endophytic fungi Arthrinium state of Apiospora montagnei Sacc. and Papulaspora immerse. Braz Arch Biol Technol. 2010;53:629–632.
- He Y, Zhang Z. Diversity of organism in the Usnea longissima lichen. Afr J Microbiol Res. 2012;6:4797–4804.
- Zhao YM, Deng CR, Chen X. Arthrinium phaeospermum causing dermatomycosis, a new record of China. Acta Mycol Sin. 1990;9:232–235.
- Hong JH, Jang S, Heo YM, et al. Investigation of marine-derived fungal diversity and their exploitable biological activities. Mar Drugs. 2015;13:4137–4155.
- Shrestha P, Ibáñez AB, Bauer S, et al. Fungi isolated from Miscanthus and sugarcane: biomass conversion, fungal enzymes, and hydrolysis of plant cell wall polymers. Biotechnol Biofuels. 2015;8:38.
- Gené J, Guarro J, Figueras MJ. A new species of Cryptosporiopsis causing bud rot of Corylus avellana. Mycol Res. 1990;94:309–312.
- Kowalski T, Halmschlager E, Schrader K. Cryptosporiopsis melanigena sp. nov., a root-inhabiting fungus of Quercus robur and Q. petraea. Mycol Res. 1998;102:347–354.
- Nitta H, Sato T, Kobayashi T, et al. Bull’s-eye rot of Japanese pear caused by Cryptosporiopsis corticola (Edgerton) Nannfeldt. Jpn J Phytopathol. 2002;68:190.
- Zilla MK, Qadri M, Pathania AS, et al. Bioactive metabolites from an endophytic Cryptosporiopsis sp. inhabiting Clidemia hirta. Phytochemistry. 2013;95:291–297.
- Chen C, Verkley GJM, Sun G, et al. Redefining common endophytes and plant pathogens in Neofabraea, Pezicula, and related genera. Fungal Biol. 2016; 120:1291–1322.
- Irwin JAG, Mackie JM, Marney TS, et al. Incidence of Stagonospora meliloti and Acrocalymma medicaginis in lucerne crowns and roots in eastern Australia, their comparative aggressiveness to lucerne and inheritance of reaction to S. meliloti in lucerne. Austral Plant Pathol. 2004;33:61–67.
- Zhang H, Hyde KD, McKenzie EHC, et al. Sequence data reveals phylogenetic affinities of Acrocalymma aquatica sp. nov., Aquasubmersa mircensis gen. et sp. nov. and Clohesyomyces aquaticus (freshwater coelomycetes). Cryptogam Mycol. 2012;33:333–346.
- Crous PW, Shivas RG, Quaedvlieg W, et al. Fungal planet description sheets: 214–280. Persoonia. 2014;32:184–306.
- Calvo A, Guarro J. Arthrinium aureum sp. nov. from Spain. Trans Br Mycol Soc. 1980;75:156–157.