Abstract
Aspergillus species play a crucial role in terrestrial environments as degraders and are well known for producing various secondary metabolites. Recently, Aspergillus species have been discovered in marine environments, exhibiting adaptability to high salinity and producing diverse secondary metabolites with valuable properties. However, limited research has focused on their marine diversity, leading to inaccurate species identification. The current study addresses this gap by investigating diverse marine habitats in the Republic of Korea, including sediment, seawater, seaweed, and marine animals. From three coasts of the Korean Peninsula, 472 Aspergillus strains were isolated from the various marine habitats. A total of 41 species were accurately identified using multigenetic markers: internal transcribed spacer, calmodulin, and β-tubulin. The findings underscore the importance of accurate identification and provide a basis for elucidating the functional role of marine-derived Aspergillus species in marine ecosystems.
1. Introduction
Aspergillus is a genus of diverse fungal species that commonly grow on the surface of various substrates in terrestrial environments. The genus Aspergillus plays an important role in ecosystems as degraders of a wide range of natural organic substrates. Aspergillus species possess asexual spore-forming structure, aspergillum and many teleomorph genera have been designated to Aspergillus anamorph such as Chaetosartorya, Emericella, Eurotium, and Neosartorya [Citation1]. Even though they were recognized as distinct sexual genera, various studies have shown that they all belong to monophyletic Aspergillus [Citation2,Citation3]. The genus comprises six subgenera, 27 sections, and 446 species [Citation4]. Aspergillus contributes significantly to the industry by producing organic acids, antibiotics, and degrading polysaccharides such as cellulose [Citation5–9]. Aspergillus species have also been known as endophytes of plants [Citation10]. Some species are mycotoxin producers, food spoilers, and human pathogens [Citation11].
Recently, Aspergillus species have also been reported from marine environments. Marine-derived Aspergillus have been primarily isolated from intertidal zones, deep-sea sediment, sponges, and algae [Citation12–15]. There are 47 Aspergillus species reported from marine habitats [Citation16]. Marine-derived Aspergillus can grow in marine environments with more than 30% salinity [Citation17,Citation18]. They produce a large number of secondary metabolites with antimicrobial, cytotoxic, insecticidal, neuroprotective, and antioxidant effects [Citation19,Citation20]. Aspergillus species contribute significantly to the degradation of marine waste as they are efficient at cellulose degradation [Citation21]. Although research on marine-derived Aspergillus have been reported, few studies have been undertaken on the diversity of marine Aspergillus. Studies have primarily focused on discovering bioactive compounds or testing enzyme activity. Therefore, the identification of many Aspergillus species has been reported as inaccurate because the identification was dependent on morphological traits and/or the internal transcribed spacer (ITS) sequence. For the identification of Aspergillus, growth rate, the color of the colony, and size of conidial heads and conidia are crucial characteristics [Citation22]. However, both the morphological features and ITS are insufficient for species level identification of Aspergillus [Citation22].
Marine-derived Aspergillus species were isolated from mudflats and sea sand of the eastern and southern coasts [Citation14] and decaying spot of macroalgae (Agarum clathratum) in the Republic of Korea [Citation23]. Since then, we have isolated many strains of Aspergillus from various marine environments. In this study, we investigated the diversity of marine-derived Aspergillus species in the Republic of Korea as a part of a project organized by the Marine Fungal Resource Bank (MFRB). We used multigenetic markers, ITS, calmodulin (CaM), and the β-tubulin (BenA) for Aspergillus identification using a standardized method [Citation22].
2. Materials and methods
2.1. Sample collection and isolation
The marine-derived samples were collected around marine environments of the Republic of Korea during 2006–2022 (Table S1). Mudflat and sea sand samples were collected at 2–3 cm depth after removing the topsoil to avoid surface contamination. The deep-sea sediment samples were collected using a Smith-Mcintyre grab sampler. 5 g of each sediment was diluted tenfold or hundredfold with sterilized seawater (SSW). Each dilution with 100 μL was spread on dichloran rose bengal chloramphenicol agar (DRBC, Difco, Becton Dickinson, Sparks, MD, USA), glucose yeast extract agar (GYA; 1 g L−1 glucose, 0.1 g L−1 yeast extract, 0.5 g L−1 peptone, and 15 g L−1 agar, Oxoid, Hampshire, UK) media, or Sabouraud dextrose agar (SNA, Difco, Becton Dickinson, Sparks, MD, USA). Seawater was sampled from the surface or bottom with sterilized bottles or using Niskin bottles equipped in the conductivity, temperature, and depth (CTD) rosettes and filtered through a sterile polycarbonate track-etched membrane filter (d = 47 mm, ϕ = 0.2 μm, GVS Filter Technology, Sanford, USA) by using a vacuum pump as done in a previous study [Citation24]. The filters were placed and cultured on DRBC agar for 7–28 days. The crab, sandfish egg, seaweed, shellfish, sponge, and starfish were collected and transported in sterile bags on ice. To remove surface debris and soil, each sample was washed with SSW. Using sterilized scissors, samples were cut into 5 × 5 mm pieces. Each sample was transferred to DRBC, GYA, or SNA. Plates were incubated at 25 °C for 7–14 days. Each culture was transferred to a new PDA plate to obtain pure cultures. A map of where the samples were collected was conducted using R version 4.1.3 [Citation25]. Identified strains were visualized on the map by using the R ggmap package with Adobe Photoshop 2022 (Adobe Systems, USA) software [Citation26].
2.2. DNA extraction, PCR amplification, and sequencing
AccuPrep Genomic DNA extraction kit (Bioneer Co., Daejeon, Korea) was used to extract genomic DNA from the fungal mycelia, following the manufacturer’s protocol. The procedure was slightly modified using CTAB buffer instead of a TL buffer. PCR amplifications of ITS, BenA, and CaM markers were done using primer pairs of ITS1F/ITS4 [Citation27], Bt2a/Bt2b [Citation28], and CF1/CF4 or CMD5/CMD6 [Citation29,Citation30], respectively. PCR was conducted on a C1000 thermal cycler (Bio-Rad, Hercules, California, USA) with the AccuPower® PCR PreMix (Bioneer Co., Daejeon, Korea) in a final volume of 20 μl, containing 10 pmol of each primer and 10 ng of DNA. PCR amplification was done following the conditions mentioned in [Citation22]. The PCR products were purified using the Expin™ PCR SV Kit (GeneAll Biotechnology, Seoul, Korea) or an ExoSAP-IT Express PCR Product Cleanup (Thermo Fisher Scientific, Waltham, Massachusetts, USA) following the manufacturer’s guidelines. Both forward and reverse directions were sequenced. Sequencing was done at Macrogen (Seoul, Korea) using an ABI PRISM 3700 Genetic Analyzer (Life Technologies, Carlsbad, California, USA). Forward and reverse sequences were assembled using the De novo assemble function in the Geneious Prime software ver. 2022.0.2. (Biomatters Ltd., San Diego, California, USA) [Citation31]. Sequences were deposited in GenBank. GenBank accession numbers including reference sequences are provided in Table S2.
2.3. Phylogenetic analysis
Reference sequences were downloaded from GenBank based on NCBI blast search and following recent publications. Sequences were aligned using MAFFT v. 7 online server (http://mafft.cbrc.jp/alignment/server/index.html) [Citation32] and improved manually using the Geneious Prime (Biomatters Inc., USA) software. Aspergillus isolates were assigned to sections using the maximum likelihood (ML) tree generated based on ITS sequences. The phylogenetic analysis for entire species was conducted with combined sequences of ITS, BenA, and CaM. ML phylogenetic analyses were conducted using RAxML v. 8.2 [Citation33] with 1000 rapid bootstraps. The final tree was selected among suboptimal trees from each run by comparing likelihood scores with the GTRGAMMA nucleotide substitution model.
3. Results
Based on the ITS sequence data, 472 Aspergillus strains were recognized from various marine habitats along three seasides of the Republic of Korea (). They were further identified as 41 different Aspergillus species in 15 sections using phylogenetic analysis of combined ITS, BenA, and CaM markers. All Aspergillus strains were visualized with ML tree with 91 reference type strains (). The final alignments comprised 632 bases for ITS, 524 for BenA, and 779 for CaM. The Aspergillus and Nidulantes sections are the most dominant sections in the marine habitats in the Republic of Korea, where each of six species were found (, ).
Figure 1. Map showing the location of sampling sites and bar graph with the number of Aspergillus strains and species isolated from each seaside. The colored dots indicate the habitats: marine animal (red), seawater (green), seaweed (blue), sediment (purple).
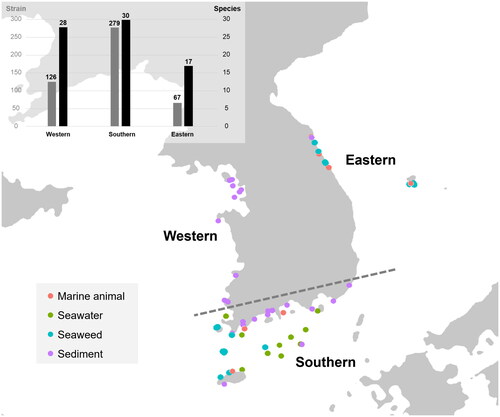
Figure2. Maximum likelihood phylogenetic tree of Aspergillus species based on the combined data set of ITS, BenA, and CaM sequences. Bootstrap values >70 are presented at the nodes. Thick lines on branches indicate 100 bootstrap support. The scale bar represents the number of nucleotide substitutions per site. “T” indicates the ex-type strains. Aspergillus species reported in this study are collapsed at a species level, and the number of SFC strains is indicated in parentheses.
Table 1. Discovered Aspergillus species with the habitat and seaside information.
Aspergillus species were reported from a variety of substrates. From marine sediment, the most diverse Aspergillus species were discovered from mudflats (37 species) followed by sea sand (14 species) and deep-sea sediment (2 species). Eight species were isolated from seawater at the surface and/or at bottom depth. Eighteen species were isolated from the seaweed and 12 species from marine animals including crabs, sandfish eggs, shellfish, sponges, and starfish (). The most diverse species were discovered from the southern seaside with 30 species, followed by 28 from the western and 17 species from the eastern seaside (). Of the 41 species, 12 species were isolated from all three seasides (). Detailed information for each strain is provided in Supplementary Table 1.
4. Discussion
Although marine-derived Aspergillus species have been discovered in various habitats, no research has been conducted on its overall marine diversity. In this study, we isolated 472 Aspergillus strains from diverse marine habitats, including sediments, seawater, seaweed, and marine animals around the seasides of the Republic of Korea. Overall, 41 species (in 15 sections) were identified based on the analyses of ITS, CaM, and BenA genetic markers (). We used the latest version of the name of Aspergillus species [Citation4,Citation34,Citation35–37]. Thirty one Aspergillus species were first discovered in new to marine environments compared with the previous list [Citation16]. Twelve species have been recorded for the first time from the marine environments in South Korea. Even though our research was limited to the marine environment of the Republic of Korea, we discovered a number of Aspergillus species from marine sites.
Based on our collection, the section Aspergillus (6 species) and Nidulantes (6) are the most diverse sections followed by Fumigati (5), Nigri (5), and Circumdati (3) in marine habitats. However, at the subgenus level, all subgenera contained marine-derived species. This suggests that there is no specific marine-adapted clade, and that the majority of the Aspergillus living on terrestrial environments can survive in marine environments with salt tolerance and osmotolerant mechanisms [Citation38–40]. Dominantly isolated species such as A. clavatus, A. creber, A. fumigatus, A. niger, A. sydowii, and A. terreus are also commonly found in indoor or terrestrial environments [Citation41,Citation42]. To date, salt-barriers have been known to play a role in dividing communities between species. However, fungi are strong colonizers on both terrestrial and marine environments, and transitions between the environments occur frequently [Citation43]. Further research is needed to elucidate the unique characteristics and ecological role of marine-derived Aspergillus in the marine environment.
The three seasides of the Republic of Korea have different characteristics and diversities because of differences in ocean currents and the depth of the surrounding oceans [Citation44,Citation45]. In some organisms, such as planktonic animals or protists, each seaside constitutes a significantly different community [Citation46,Citation47]. Additionally, habitat-dependent fungal species show differences in coastal distribution because of the different environments formed by each seaside [Citation48]. However, most Aspergillus species were found in two or all the three seasides, except for single strain isolated species. The excellent decomposition ability and tolerance to extreme environments may have enabled the isolation of Aspergillus in various habitats [Citation49]. Therefore, the unique species found in each seaside is also likely to be found on other seasides through further research.
The mudflat habitat was the most diverse environment, where 37 Aspergillus species were discovered. The intertidal mudflat habitats feature a high level of microbiological diversity [Citation50,Citation51]. In comparison, 14 species were identified from sea sand. These results are comparable to the findings of [Citation14], in which 13 species were reported from mudflat, and five species were reported from sea sand. In our study, A. fumigatus (36 strains), A. clavatus (34), A. terreus (27), A. niger (24), and A. sydowii (18) were the commonly found species from mudflats. Among them, A. fumigatus, A. niger, and A. sydowii have high cellulose-degrading capabilities [Citation52]. Of all marine-derived fungi, Aspergillus species produce diverse secondary metabolites [Citation19]. Some Aspergillus species have been studied extensively as opportunistic pathogens of marine organisms [Citation53,Citation54]. Aspergillus species are well known for producing marine-derived enzymes [Citation55]. Using these enzymes, they are able to inhabit intertidal zones by interacting with various marine organisms. Commonly isolated A. sydowii and A. versicolor were also detected in seawater and deep-sea sediment. According to their tolerance for osmotic pressure or salinity level [Citation12,Citation18,Citation56,Citation57], they possibly withstand other extreme or malnutrition environments. In this study, 18 Aspergillus species were isolated from seaweeds. They may play the role of saprotrophs by degrading seaweeds using enzymes such as cellulase [Citation58]. Some Aspergillus species are adapted to colonize seaweed as endosymbionts, which explains the continuous isolation of certain species from seaweed [Citation59–61]. Furthermore, several species have shown strong protease activity using caseinase and gelatinase in previous studies [Citation62]. These enzymes were examined in the Aspergillus species isolated from marine organisms such as crabs, sandfish egg masses, sponges, and shells [Citation62]. By decomposing these organisms, Aspergillus can contribute markedly to the nutrient circulation in the marine environment.
In conclusion, this study has focused on marine habitats in South Korea and reports several unrecorded Aspergillus species in marine environments. The findings suggest that Aspergillus diversity is high in marine environments, and more species are yet to be discovered. Although Aspergillus species have been reported from a variety of substrates, their role in marine environment is still unclear. To date, little research has been conducted to study the importance of Aspergillus in the marine environment and its taxonomy. The continuous discovery of Aspergillus species in marine environments will expand our understanding of their ecological importance.
Supplemental Material
Download MS Excel (32.9 KB)Supplemental Material
Download MS Excel (33.1 KB)Disclosure statement
No potential conflict of interest was reported by the author(s).
Data availability statement
All the data that support the findings of this study are available within the article.
Additional information
Funding
References
- Samson RA, Pitt JI. Integration of modern taxonomic methods for Penicillium and Aspergillus classification. FL: CRC Press; 2000.
- Kocsubé S, Perrone G, Magistà D, et al. Aspergillus is monophyletic: evidence from multiple gene phylogenies and extrolites profiles. Stud Mycol. 2016;85(1):199–213. doi: 10.1016/j.simyco.2016.11.006.
- Steenwyk JL, Shen X-X, Lind AL, et al. A robust phylogenomic time tree for biotechnologically and medically important fungi in the genera Aspergillus and Penicillium. MBio. 2019;10(4):e00925–19. doi: 10.1128/mBio.00925-19.
- Houbraken J, Kocsubé S, Visagie CM, et al. Classification of Aspergillus, Penicillium, Talaromyces and related genera (Eurotiales): an overview of families, genera, subgenera, sections, series and species. Stud Mycol. 2020;95:5–169. doi: 10.1016/j.simyco.2020.05.002.
- de Vries RP, Visser J. Aspergillus enzymes involved in degradation of plant cell wall polysaccharides. Microbiol Mol Biol Rev. 2001;65(4):497–522. doi: 10.1128/MMBR.65.4.497-522.2001.
- Hrmová M, Biely P, Vrs˘anská M, et al. Cellulose- and xylan-degrading enzymes of Aspergillus terreus and Aspergillus niger. Enzyme Microb Technol. 1989;11(9):610–616. doi: 10.1016/0141-0229(89)90090-2.
- Planchot V, Colonna P, Gallant DJ, et al. Extensive degradation of native starch granules by alpha-amylase from Aspergillus fumigatus. J Cereal Sci. 1995;21(2):163–171. doi: 10.1016/0733-5210(95)90032-2.
- Scheckermann C, Wagner F, Fischer L, et al. Galactosylation of antibiotics using the β-galactosidase from Aspergillus oryzae. Enzyme Microb Technol. 1997;20(8):629–634. doi: 10.1016/S0141-0229(96)00211-6.
- Yang L, Lübeck M, Lübeck PS, et al. Aspergillus as a versatile cell factory for organic acid production. Fungal Biol Rev. 2017;31(1):33–49. doi: 10.1016/j.fbr.2016.11.001.
- El-Hawary SS, Moawad AS, Bahr HS, et al. Natural product diversity from the endophytic fungi of the genus Aspergillus. RSC Adv. 2020;10(37):22058–22079. doi: 10.1039/d0ra04290k.
- Hedayati MT, Pasqualotto AC, Warn PA, et al. Aspergillus flavus: human pathogen, allergen and mycotoxin producer. Microbiology. 2007;153(Pt 6):1677–1692. doi: 10.1099/mic.0.2007/007641-0.
- Damare S, Raghukumar C, Raghukumar S, et al. Fungi in deep-sea sediments of the Central Indian basin. Deep Sea Res Part I. 2006;53(1):14–27. doi: 10.1016/j.dsr.2005.09.005.
- Kamat S, Kumari M, Taritla S, et al. Endophytic fungi of marine alga from Konkan Coast, India—a rich source of bioactive material. Front Mar Sci. 2020;7:31. doi: 10.3389/fmars.2020.00031.
- Lee S, Park MS, Lim YW, et al. Diversity of marine-derived Aspergillus from tidal mudflats and sea sand in Korea. Mycobiology. 2016;44(4):237–247. doi: 10.5941/MYCO.2016.44.4.237.
- Wiese J, Ohlendorf B, Blümel M, et al. Phylogenetic identification of fungi isolated from the marine sponge Tethya aurantium and identification of their secondary metabolites. Mar Drugs. 2011;9(4):561–585. doi: 10.3390/md9040561.
- Jones EBG, Suetrong S, Sakayaroj J, et al. Classification of marine Ascomycota, Basidiomycota, Blastocladiomycota and Chytridiomycota. Fungal Divers. 2015;73(1):1–72. doi: 10.1007/s13225-015-0339-4.
- Badawy AA, Alotaibi MO, Abdelaziz AM, et al. Enhancement of seawater stress tolerance in barley by the endophytic fungus Aspergillus ochraceus. Metabolites. 2021;11(7):428. doi: 10.3390/metabo11070428.
- Kis-Papo T, Oren A, Wasser SP, et al. Survival of filamentous fungi in hypersaline dead sea water. Microb Ecol. 2003;45(2):183–190. doi: 10.1007/s00248-002-3006-8.
- Lee YM, Kim MJ, Li H, et al. Marine-derived Aspergillus species as a source of bioactive secondary metabolites. Mar Biotechnol (NY). 2013;15(5):499–519. doi: 10.1007/s10126-013-9506-3.
- Orfali R, Aboseada MA, Abdel-Wahab NM, et al. Recent updates on the bioactive compounds of the marine-derived genus Aspergillus. RSC Adv. 2021;11(28):17116–17150. doi: 10.1039/d1ra01359a.
- Jones EG, Pang KL. Marine fungi: and fungal-like organisms. Berlin (Germany): Walter de Gruyter; 2012.
- Samson RA, Visagie CM, Houbraken J, et al. Phylogeny, identification and nomenclature of the genus Aspergillus. Stud Mycol. 2014;78(1):141–173. doi: 10.1016/j.simyco.2014.07.004.
- Lee S, Park MS, Lee H, et al. Fungal diversity and enzyme activity associated with the macroalgae, Agarum clathratum. Mycobiology. 2019;47(1):50–58. doi: 10.1080/12298093.2019.1580464.
- Lee W, Kim JS, Seo CW, et al. Diversity of Cladosporium (Cladosporiales, Cladosporiaceae) species in marine environments and report on five new species. MycoKeys. 2023a;98:87–111. doi: 10.3897/mycokeys.98.101918.
- R Core Team. R: a language and environment for statistical computing. R Foundation for Statistical Computing, Vienna, Austria; 2022. Available from: http://www.R-project.org/.
- Kahle DJ, Wickham H. GGMAP: spatial visualization with ggplot2. The R J. 2013;5(1):144–161. doi: 10.32614/RJ-2013-014.
- White TJ. Amplification and direct sequencing of fungal ribosomal RNA genes for phylogenetics. PCR Protocol Guide Method Appl. 1990;18(1):315–322.
- Glass NL, Donaldson GC. Development of primer sets designed for use with the PCR to amplify conserved genes from filamentous ascomycetes. Appl Environ Microbiol. 1995;61(4):1323–1330. doi: 10.1128/aem.61.4.1323-1330.1995.
- Hong S-B, Go S-J, Shin H-D, et al. Polyphasic taxonomy of Aspergillus fumigatus and related species. Mycologia. 2005;97(6):1316–1329. doi: 10.1080/15572536.2006.11832738.
- Peterson SW. Phylogenetic analysis of Aspergillus species using DNA sequences from four loci. Mycologia. 2008;100(2):205–226. doi: 10.1080/15572536.2008.11832477.
- Kearse M, Moir R, Wilson A, et al. Geneious basic: an integrated and extendable desktop software platform for the organization and analysis of sequence data. Bioinformatics. 2012;28(12):1647–1649. doi: 10.1093/bioinformatics/bts199.
- Katoh K, Standley DM. MAFFT multiple sequence alignment software version 7: improvements in performance and usability. Mol Biol Evol. 2013;30(4):772–780. doi: 10.1093/molbev/mst010.
- Stamatakis A. RAxML version 8: a tool for phylogenetic analysis and post-analysis of large phylogenies. Bioinformatics. 2014;30(9):1312–1313. doi: 10.1093/bioinformatics/btu033.
- Varga J, Frisvad JC, Kocsubé S, et al. New and revisited species in Aspergillus section Nigri. Stud Mycol. 2011;69(1):1–17. doi: 10.3114/sim.2011.69.01.
- Bian C, Kusuya Y, Sklenář F, et al. Reducing the number of accepted species in Aspergillus series Nigri. Stud Mycol. 2022;102(1):95–132. doi: 10.3114/sim.2022.102.03.
- Sklenář F, Jurjević Ž, Houbraken J, et al. Re-examination of species limits in Aspergillus section Flavipedes using advanced species delimitation methods and description of four new species. Stud Mycol. 2021;99(1):100120–100120. doi: 10.1016/j.simyco.2021.100120.
- Sklenář F, Glässnerová K, Jurjević Ž, et al. Taxonomy of Aspergillus series Versicolores: species reduction and lessons learned about intraspecific variability. Stud Mycol. 2022;102(1):53–93. doi: 10.3114/sim.2022.102.02.
- Araújo CA, Ferreira PC, Pupin B, et al. Osmotolerance as a determinant of microbial ecology: a study of phylogenetically diverse fungi. Fungal Biology. 2020;124(5):273–288. doi: 10.1016/j.funbio.2019.09.001.
- Cai L, Xu S, Lu T, et al. Salt-tolerant mechanism of marine Aspergillus niger cellulase cocktail and improvement of its activity. Chin J Chem Eng. 2020;28(4):1120–1128. doi: 10.1016/j.cjche.2019.11.012.
- Jalili B, Bagheri H, Azadi S, et al. Identification and salt tolerance evaluation of endophyte fungi isolates from halophyte plants. Int J Environ Sci Technol. 2020;17(7):3459–3466. doi: 10.1007/s13762-020-02626-y.
- Abdel-Azeem AM, Abdel-Azeem MA, Abdul-Hadi SY et al. Aspergillus: biodiversity, ecological significances, and industrial applications. In Mishra S, Singh S, Gupta A, et al., editors, Recent advancement in white biotechnology through fungi: volume 1: diversity and enzymes perspectives. Cham; Germany: Springer; 2019. p. 121–179.
- Espinosa SKC, et al. Phylogenetic identification, diversity, and richness of Aspergillus from homes in Havana, Cuba. Microorganisms. 2021;9(1):115.
- Jamy M, Biwer C, Vaulot D, et al. Global patterns and rates of habitat transitions across the eukaryotic tree of life. Nat Ecol Evol. 2022;6(10):1458–1470. doi: 10.1038/s41559-022-01838-4.
- Chang KI, Zhang CI, Park C, et al. Oceanography of the east sea (Japan Sea). Cham: Springer International Publishing; 2016. p. 17.
- Koh CH, Khim JS. The Korean tidal flat of the yellow sea: physical setting, ecosystem and management. Ocean Coastal Manage. 2014;102:398–414. doi: 10.1016/j.ocecoaman.2014.07.008.
- Baek SH. First report for appearance and distribution patterns of the epiphytic dinoflagellates in the Korean Peninsula. Korean J Environ Biol. 2012;30(4):355–361. doi: 10.11626/KJEB.2012.30.4.355.
- Seo MH, Choi SY, Park E-O, et al. Species diversity of planktonic copepods and distribution characteristics of its major species in coastal waters of Korea. Korean J Environ Biol. 2018;36(4):525–537. doi: 10.11626/KJEB.2018.36.4.525.
- Lee JW, Seo CW, Lee W, et al. Diversity and dynamics of marine arenicolous fungi in three seasides of the Korean peninsula. J Microbiol. 2023b;61(1):63–82. doi: 10.1007/s12275-023-00011-1.
- Jones EG. Marine fungi: some factors influencing biodiversity. Fungal Divers. 2000;4:53–73.
- Li P-D, Jeewon R, Aruna B, et al. Metabarcoding reveals differences in fungal communities between unflooded versus tidal flat soil in coastal saline ecosystem. Sci Total Environ. 2019;690:911–922. doi: 10.1016/j.scitotenv.2019.06.473.
- Lv X, Ma B, Yu J, et al. Bacterial community structure and function shift along a successional series of tidal flats in the yellow river delta. Sci Rep. 2016;6(1):36550. doi: 10.1038/srep36550.
- Moustafa AF, Sharkas MS. Fungi associated with cellulose decomposition in the tidal mud-flats of Kuwait. Mycopathologia. 1982;78(3):185–190. doi: 10.1007/BF00466074.
- Harvell D, Jordán-Dahlgren E, Merkel S, et al. Coral disease, environmental drivers, and the balance between coral and microbial associates. Oceanog. 2007;20(1):172–195. doi: 10.5670/oceanog.2007.91.
- Zuluaga-Montero A, Toledo-Hernández C, Rodríguez JA, et al. Spatial variation in fungal communities isolated from healthy and diseased sea fans Gorgonia ventalina and seawater. Aquat Biol. 2010;8(2):151–160. doi: 10.3354/ab00218.
- Bonugli-Santos RC, Dos Santos Vasconcelos MR, Passarini MRZ, et al. Marine-derived fungi: diversity of enzymes and biotechnological applications. Front Microbiol. 2015;6:269. doi: 10.3389/fmicb.2015.00269.
- Harpke M, Pietschmann S, Ueberschaar N, et al. Salt and metal tolerance involves formation of guttation droplets in species of the Aspergillus versicolor complex. Genes. 2022;13(9):1631. doi: 10.3390/genes13091631.
- Rodríguez-Pupo EC, Pérez-Llano Y, Tinoco-Valencia JR, et al. Osmolyte signatures for the protection of Aspergillus sydowii cells under halophilic conditions and osmotic shock. JoF. 2021;7(6):414. doi: 10.3390/jof7060414.
- Patyshakuliyeva A, Falkoski DL, Wiebenga A, et al. Macroalgae derived fungi have high abilities to degrade algal polymers. Microorganisms. 2019;8(1):52. doi: 10.3390/microorganisms8010052.
- Suryanarayanan TS. Fungal endosymbionts of seaweeds. In: Raghukumar C, editor, Biology of marine fungi. Berlin (Germany): Springer; 2011. p. 53–69.
- Suryanarayanan TS, Venkatachalam A, Thirunavukkarasu N, et al. Internal mycobiota of marine macroalgae from the Tamilnadu coast: distribution, diversity and biotechnological potential. De Gruyter. 2010;53(5):457–468.
- Zuccaro A, Schoch CL, Spatafora JW, et al. Detection and identification of fungi intimately associated with the brown seaweed Fucus serratus. Appl Environ Microbiol. 2008;74(4):931–941.
- Park MS, Oh S-Y, Lee S, et al. Fungal diversity and enzyme activity associated with sailfin sandfish egg masses in Korea. Fungal Ecol. 2018;34:1–9. doi: 10.1016/j.funeco.2018.03.004.