Abstract
The intestinal lactic acid microflora of the edible snail Cornu aspersum was studied by culture-based methods and was phenotypically and molecularly characterized. The antibacterial activity of lactic acid bacteria (LAB) isolates was investigated. Snails in different stages of development were collected from farms located in several regions of Bulgaria. One hundred twenty-two isolates, belonging to the group of LAB, were characterized morphologically and were divided into four groups. Representative isolates from each morphological type were subjected to phenotypic characterization and molecular identification. The snail gut lactic acid microflora was composed by Enterococcus (17 isolates), Lactococcus (12 isolates), Leuconostoc (7 isolates), Lactobacillus (18 isolates) and Weissella (1 isolate). The species affiliation of Lactococcus lactis (12), Leuconostoc mesenteroides (4) and Lactobacillus plantarum (2) was confirmed by species-specific primers. The Lactobacillus isolates were identified by sequence analysis of 16S rDNA as Lactobacillus brevis (12), L. plantarum (2), Lactobacillus graminis (1) and Lactobacillus curvatus (3). The species L. brevis, L. graminis and L. curvatus were found in snails in a phase of hibernation, whereas L. plantarum was identified both in active and hibernation phases. Antibacterial activity (bacteriocine-like) was shown only by one strain of L. mesentereoides P4/8 against Propionibacterium acnes. The present study showed that the LAB are a component of the microbial communities in the snail digestive system. This is the first report on Lactobacillus strains detected in the gut of C. aspersum.
Introduction
Lactic acid bacteria (LAB) are an important part of the microbial population in the digestive tract of many animal species including pigs, fowls, rodents, chicken, horses, gastropods and insects, and play a significant role in maintaining the ecological equilibrium between the different species of microorganisms inhabiting these environments. This microbiota could also participate in the digestive process (fermentation) and the energy supply (L-lactate, acetate).[Citation1] Terrestrial gastropods, as herbivore, eat fresh plants with high protein and calcium contents [Citation2] and participate in the decomposition of leaf litter.[Citation3] Their extraordinary efficiency in plant digestion (60%–80%) depends predominantly on the metabolitic activities of the intestinal microflora.[Citation1] It was presumed that the alimentary tract is a major component of interaction between an animal's ecosystem and its physiology. The gut health of farm animals is closely related to the microbial balance of the intestinal flora. The diversity of bacterial microflora in the intestine of edible snails Cornu aspersum (Syn: Helix aspersa) and Helix pomatia has been studied by culture-based methods, 16S rRNA sequence analyses and phenotypic characterizations.[Citation1] The bacterial species in the snail gut were arranged into two taxa: γ-Proteobacteria (Buttiauxella, Citrobacter, Enterobacter, Kluyvera, Obesumbacterium, Raoultella) and the Firmicutes (Enterococcus, Lactococcus and Clostridium). These genera are mostly assigned to enteric environments or to phyllosphere, data in favour of culturing snails in contact with soil and plants.[Citation1] However, few studies investigated the presence and relative roles of LAB in food digestion and host nutrition. Enterococcus casseliflavus was already described as a dominant Gram-positive species in the gut of C. aspersum and was generally assigned to ‘enteric’ environments. This prevalence was explained by Charrier et al. [Citation4] with the balance between the physico-chemical conditions in the snail intestine and the environmental requirements of epiphyte enterococci (growth at a pH > 5.0, a high humidity, temperature of 10 °C). Furthermore, the occurrence of active pullulanases in E. casseliflavus suggests culturing C. aspersum with amylopectin-rich cereals (maize, rice and sorghum) and to favour plant decaying – infestation by pullulan rich fungi, like basidiomycetes, part of gastropod food.[Citation5] The presence of lactococci in the snail gut could be related with production of lactic acid. Moreover, Lactococcus lactis possesses a surface protein (HtrA), which is a key factor in the response to specific stress conditions [Citation6] and significant for the survival of bacteria in the snail gut during hibernation. The presence of these two genera in the snail's intestine suggests that lactic acid might play an important role during the digestive process in the snail. Lactic acid has also been found to be a stimulatory response in a marine gastropod Nassarius obsoletus.[Citation7] The occurrence of epiphyte enterococci as the dominant lactic bacterium in the snail's intestine, and not lactobacilli such as Lactobacillus plantarum also widespread on decaying plants is interesting.[Citation8] Currently, there are no publications demonstrating the presence of other plant associated LAB (as Lactobacillus and Leuconostoc) in the intestinal tract of snails.
The aim of the present work was to isolate, cultivate, characterize and identify by phenotypic and genotypic methods the LAB associated with the snail's gut in order to improve the understanding of this microbial environment. In vitro determination of antimicrobial spectra against the bacterial and human foodborne pathogen was also the object of this study.
Materials and methods
Snail intestinal tract sampling
Six samples of C. aspersum (Gastropoda, Pulmonata) in different physiological stages were taken from various private snail farms in Bulgaria. All investigated samples were kept under starvation for approximately two weeks. The shells of snails were sterilized with ethanol (70 °C) and were removed aseptically. Snails were washed with sterile physiological solution and were dissected in vivo under aerobic conditions. The whole intestinal tract (esophagus to rectum) was aseptically handled to avoid contamination from external surface of the snail's body. The samples were used to obtain homogenates in physiological solution (0.85% w/w NaCl).
Culture media
The aliquots (100 μL) of the different dilutions of the gut samples were spread in duplicate onto MRS and M17 agar medium (Merck). The plates were incubated at 28 and 37 °C for 48–72 hours in anaerobic conditions (Anaerocult, BioMerieux) in anoxic jars. The number of colony-forming units (CFU) per mL was counted after the incubation period.
Phenotypic characterization of LAB
The morphology of the colonies forming after inoculation and cultivation on the media with sample dilutions was studied. Distinct colonies, which possessed different morphology, that were catalase and oxydase negative and consisted of Gram-positive cells were picked and further studied. Carbohydrate fermentation patterns (API CH50, API Strep 20, Biomerieux) and phenotypic identification according to basic biochemical tests were carried out for representative isolates.[Citation9] All strains were stored as 40% glycerol stock cultures at −20 °C.
Molecular differentiation of LAB
Molecular differentiation of isolated LAB at the genus level was carried out by polymerase chain reaction (PCR) amplification of 16S-23S ITS rDNA with the universal primers 16S/4 and 23S/7 as previously described.[Citation10] Species grouping and species identification were performed by a two-step multiplex PCR according Song et al.[Citation11] The genus and species affiliation of the strains obtained by the above methods was confirmed by PCR amplification with genus- and species-specific primer pairs – LbLMA1/R16-1,[Citation10] Lpla2/Lpla-3,[Citation11] LmeF/LmeR, and LlaF/LlaR,[Citation12] respectively. The primers CA1/CA2, Efm1/Efm2 and Efs1/Efs2 were applied for differentiation and identification of E. casseliflavus,[Citation13] Enterococcus faecium and Enterococcus faecalis,[Citation14] respectively.
Total DNA of selected LAB strains was extracted from overnight culture on MRS medium with DNA kit prep GEMTM Bacteria (ZyGEM). PCRs were performed in a total volume 25 μL containing 6.5 μL VWR Red Taq polymerase master Mix (VWr, Denmark), 1 μL of each primer (0.3 μmol/L concentration), 1 μL DNA (ca. 50 ng) and 15.5 μL H2O. PCRs were carried out in a thermocycler TC 312 (Techne). The reaction conditions were as follows: an initial denaturation step at 94 °C for 5 min, followed by 25 cycles at 94 °C for 45 s, 58 °C, 55 °C or 52 °C (according primers specificity) for 45 s, extension step at 72 °C for 45 s, and a final extension step for 7 min at 72 °C. The PCR products (aliquots of 5–10 μL) were resolved electrophoretically in 1.5% agarose gels (Agarose, DNA grade, Electran) in TBE 10× buffer at 100 V for 45 min. Gels were stained with ethidium bromide (5μg mL−1) and the bands were visualized under ultraviolet illumination at 254 nm. A 100 bp DNA Ladder was used as molecular mass marker (GeneRuler100 bp, 0.5μL/mL).
16S rRNA sequencing
The respective 16S rDNA genes were amplified by PCR using the universal primers 9F and 1542R.[Citation15] The PCR products were purified and the DNA sequencing was carried out by Macrogen Services. Sequences were compared and aligned with those from the GenBank database using the BLAST program of the National Center for Biotechnology Information (NCBI; http://www. ncbi.nlm.nih.gov) network server.
Antimicrobial activity of LAB
The antimicrobial activity of isolated LAB was determined by the agar overlay method.[Citation16] The antibacterial activity of cell-free supernatants (CFS), obtained after cultivation of the LAB, was evaluated by the agar well diffusion method.[Citation17] The CFS were obtained after centrifugation (10,000 g, 15 min) of 24 h LAB cultures in MRS and M17 broth (for lactobacilli and lactococci, respectively) cultivated at 28 °C for 24 h. CFSs were filter-sterilized and kept at 4 °C. In order to eliminate the action of lactic acid on the test-bacteria the pH of portions of the supernatants was adjusted to 6.0 with 6 mol/L NaOH. The sensitivity of the active substances to proteolytic enzymes and heat was estimated by treatment of the neutralized supernatants with proteinase K (0.2 mg/mL, Boehringer, Mannheim, Germany) and heating at 60 and 80 °C for 10 min, respectively. Nine different Gram-positive and Gram-negative test-bacteria – Salmonella enteritica serotype Enteritidis, Salmonella enteritica serotype Choleraesuis NBIMCC 2333, Propionibacterium acnes KPA, Propionibacterium acnes PA 266, Listeria innocua F (ONIRIS, Nantes, France) Bacillus subtilis NBIMCC 2353 (ATCC 6051), Escherichia coli NBIMCC 3397, E. coli NBIMCC 3548 and Stapyloccocus epidermidis NBIMCC 1093 were used in this study. The antibacterial activity of all variants CFSs (native, neutralized, treated with proteinase K and heated) was detected as inhibitory zones around the wells in the agar, inoculated with the test-bacteria.
Results and discussion
Enumeration and isolation of LAB
Six cultivated snails C. aspersum were investigated in this study. The snails in different stages in the life cycle were collected from private farms located in different regions of Bulgaria. The total number of LAB enumerated on MRS and M17 medium was higher (108 CFU/mL) in breeding conditions than during hibernation (105 CFU/mL).
Of about 350 colonies that were formed on the two medium, 122 small, round and opaque, and white colonies showed characteristics of LAB: Gram-positive non-spore-forming rods or cocci, catalase and oxydase negative, and aerotolerant. Fifty-five presumptive LAB strains were purely isolated and were phenotypically characterized (). Thirty-nine of the strains were homofermentative and 15 isolates – heterofermentative.
Table 1. Phenotypical characterization of the most representative lactic acid bacteria isolated from snail's gut.
Molecular differentiation of LAB
All purified strains were subjected to a preliminary molecular characterization using the 16S-23S ITS PCR approach and were differentiated at the genus level. The results of the amplifications are shown in . Thirty-five strains formed amplification pattern with two fragments. The larger fragment was the same in all studied strains – about 650 bp, whereas the smaller fragment was about 450 bp in 18 and about 550 bp in 17 of these strains. Seven isolates showed the profile with one major band of approximately 600 bp, 12 isolates – one fragment of 500 bp and one LAB isolate – three fragments (450, 550, 650 bp). Comparing our results with the literature data,[Citation10] it could be concluded that 18 of our isolates belonged to the genus Lactobacillus, 17 – to the genus Enterococcus, 7 – to the genus Leuconostoc, 12 – to the genus Lactococcus, and one strain – to the genus Weissella, as well they formed characteristic amplification pattern to the corresponding genera.
Figure 1. PCR-amplification of 16S-23S ITRs (16S-4/23S-7 primers) of LAB strains. Lane 1: strain P3/3, lane 2: P3/4, lane 3: P3/5, lane 4: L. lactis 454, lane 5: P3/8, lane 6: P3/9, lane 7: P4/1, lane 8: P4/8, lane 9: P4/9, lane 10: P4/11, lane 11: P1/30, lane 12: P2/4, lane13: Enterococcus faecium, lane 14: P2/18, lane 15: P2/19, lane 16: P2/24, lane 17: P4/6, lane 18: P2/32, lane 19: P4/2, lane 20: Ln mesenteroides ATCC 8293 and lane 21: L. plantarum NBIMCC 297, M-molecular weight marker (100-bp DNA ladder, Fermentas).
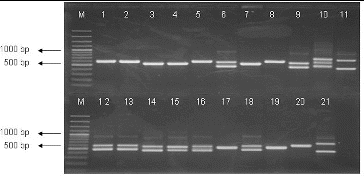
Identification of lactobacilli
The confirmation of the affiliation of the strains identified by 16S-23S ITS PCR as member of the genus Lactobacillus was performed with lactobacilli genus-specific primer (LbLMA1). All 18 strains amplified an expected fragment of about 250 bp [Citation18]. These results proved their belonging to the genus Lactobacillus ().
Figure 2. PCR-amplification of Lactobacillus strains with genus specific primer LBMA1. Lane 1: strain P1/1, lane 2: strain P1/2, lane 3: strain P1/3, lane 4: strain P1/4, lane 5: strain P1/5, lane 6: strain P1/14, lane 7: strain P1/22, lane 8: strain P1/24, lane 9: strain P1/26, lane 10: strain P1/27; lane 11: strain P1/28, lane 12: strain P5/4, lane 13: strain P5/5, lane 14: strain P5/8, lane 15: strain P5/9, lane 16: : L. plantarum NBIMCC 297, lane 17: L.fermentum NBIMCC 505, lane 18: Ln mesenteroides ATCC 8293, lane 19: L. lactis 454 and lane 20: L. plantarum 117. M-molecular weight marker (100-bp DNA ladder, Fermentas).
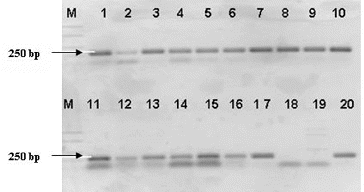
Since lactobacilli are extremely heterogeneous, they were separated into groups according Song et al.[Citation11] Therefore, we applied a two-step multiplex PCR to differentiate our isolates into previously described groups. The first multiplex PCR was performed with the four pairs of group specific primers, namely Ldel-7/Lac 2, Lu1/Lac2, Lu-5/Lac2 and Lu-3/Lac2. This approach separated the lactobacilli into four groups: Group I (Lactobacillus delbrueckii ssp. bulgaricus/lactis), Group II (Lactobacillus acidophilus, Lactobacillus helveticus, Lactobacillus amylovorus, Lactobacillus crispatus, Lactobacillus gasseri, Lactobacillus johnsonii), Group III (Lactobacillus paracasei, Lactobacillus casei and Lactobacillus rhamnosus.) and Group IV (L. plantarum and Lactobacillus fermentum), respectively.[Citation11] The obtained results showed that only the primers pair for Group IV (Lu-3/Lac2) gave the positive reaction for six of our strains (P1/30, P4/4, P5/4, P5/5, P5/8 and P5/9) forming an expected amplification band – 350 bp. These strains were subjected on a second multiplex PCR with species-specific primers for this group, and two of them (P1/30 and P4/4) were identified as L. plantarum. The four strains (P5/4, P5/5, P5/8 and P5/9) which were not identified by a two-step multiplex PCR showed similarity by fermentation patterns (API CH50) to the species Lactobacillus curvatus (91%), Lactobacillus graminis (89%) or Lactobacillus sakei (87%) ().
The rest of the investigated strains formed an amplicon of 500 bp which did not correspond to any of the described group. API 50CH fermentation profiles of these strains revealed the similarity to Lactobacillus brevis (90%) that was not confirmed by species-specific PCR.
Selected Lactobacillus strains analysed by the above-applied methods were subjected to 16S rDNA sequence analysis. Comparison of the obtained sequences with the corresponding Lactobacillus sequences available in GenBank database showed that all 14 investigated strains laid in the evolutionary clade of the Lactobacillus. Eight strains had 99%–100% identity with L. brevis ATCC 367, three strains showed 99% homology score to L. curvatus DSM 20019, one strain was identified with 99% similarity to L. graminis DSM 20719 and two strains were 100% identical with L. plantarum WCFS1. A phylogenetic tree of lactobacilli (determined in this study) was constructed using the neighbour-joining algorithms,[Citation19,20] by comparing the available sequences (NCBI). The phylogenetic relationships based on 16S resulted in the separation of isolated lactobacilli strains into two group – group I brevis and group II plantarum – curvatus – graminis ().
Figure 3. Phylogenetic tree constructed by the platform http://www.phylogeny.fr (8) based on sequences of 16S rDNA of selected LAB strains associated snail-gut: L.plantarum P1/30, L.curvatus P5/4, L.curvatus P5/8. L.graminis P5/5, L.brevis P1/1, L.brevis P1/26, L.brevis P1/28 and GenBank available sequences: L.brevis ATCC 367, L.curvatus DSM 20019 and L.graminis DSM 20719.
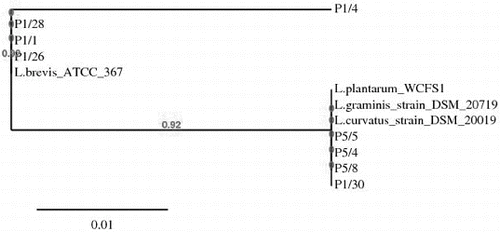
Identification of cocci
The isolates identified to the genus Lactoccoccus (12) and Leuconostoc (7) on the basis of 16S-23S ITS rDNA were subjected to species-specific multiplex PCR.[Citation12] All Lactococcus isolates gave a positive reaction with primers specific for L. lactis, forming an amplification product of about 248 bp. Four Leuconostoc isolates were identified as Leuconostoc mesenteroides on the base of resulted amplification fragment of about 358 bp (). For the all 17 Enterococcus strains the PCR amplification with species-specific primers (CA1/CA2, Efm1/Efm2 and Efs1/Efs2) was applied. Only one isolate (P4/9) was identified as E. casseliflavus. It should be noted that the test of the Enterococcus strains based on the API Strep 20 showed similarity to the species of faecium group. Our result confirmed the finding of Charier et al. [Citation1] that E. casseliflavus actually reside in snail gut. The authors also found that the lactococci occurred in H. pomatia gut, while the enterococci were present in French populations of both species (H. pomatia and C. aspersum). Our results extend the available information on the spread of lactococci in the snail species.
Figure 4. Multiplex PCR identification of strains belonging to species Lactococcus lactis and Leuconostoc mesenteroides. Lane 1: Ln mesenteroides ATCC 8293, lane 2: Р3/8, lane 3- Р4/8, lane 4 - Lactococcus lactis ssp. lactis 454, lane 5- P 3/1, lane 6 - P 3/5, lane 7- P3/6 and lane 8- P4/1, M- molecular weight marker (100-bp DNA ladder, Fermentas).
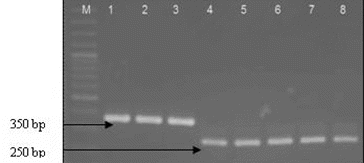
Antibacterial assessment
Antimicrobial activity of whole cells of isolated Lactobacillus (13 strains), Lactococcus (12 strains) and Leuconostoc (3 strains) strains were screened against four test-bacteria: S. enteritica serotype Enteritidis, S. enteritica serotype Choleraesuis, B. subtilis and S. epidermidis. The highest activity of lactobacilli (inhibition zone – 25–30 mm) was observed against S. enteritica serotype Enteritis, S. enteritica serotype Choleraesuis and S. epidermidis. The activity of Lactococcus and Leuconostoc strains varied.
The native CFS of the strains showed antibacterial activity against S. serotype Enteritis, S. serotype Cholaeresuis, P. acnes, L. innocua F., B. subtilis, E.coli, and S. epidermidis forming a relatively small inhibition zones (10–11 mm). Only two Leuconostoc isolates (L. mesenteroides P4/8 and P3/2) showed antibacterial activity of the neutralized CFS (pH 6.0) especially against P. acnes. This activity was reduced after heating at 80 °C for 10 min and disappeared after proteinase K treatment (). It could be suggested that the ability of the L. mesenteroides P4/8 and P3/2 to inhibit P. acnes strains may be due to the production of heat labile bacteriocin-like substance.
Conclusions
The present study confirmed that LAB are endogenous and could be assigning to ‘enteric’ environment. For the first time by molecular approach, the presence of the species L. brevis, L. plantarum, L. curvatus, L.graminis and L. mesenteroides was determined. This investigation is the first molecular typing of LAB associated with the gut of the snail C. aspersum.
Additional information
Funding
References
- Charrier M, Fonty G, Gaillard-Martinie B, Ainouche K, Andant G. Isolation and characteriazation of cultivable fermentative bacteria from the intestine of two edible snails. Helix pomatia and Cornu aspersum (Gastropoda:Pulmonata). Biol Res. 2006;39:669–681. doi:10.4067/S0716-97602006000500010.
- Iglesias J, Castillejo Y. Field observations of the land snail Helix aspersa. Müller. J Molluscan Stud. 1999;65:411–423. doi:10.1093/mollus/65.4.411.
- Hatziioannou M, Eleutheriadis N, Lazaridou-Dimitriadou M. Food preferences and dietary overlap by terrestrial snails in Logos Area (Edessa, Macedonia, Northern Greece). J Molluscan Stud. 1994;60:331–341. doi:10.1093/mollus/60.3.331.
- Charrier M, Combat-Blanc Y, Ollivier B. Bacterial flora in the gut of Helix aspersa (Gastropoda Pulmonata): evidnce for a permanent population with a dominant homolactic intestinal bacterium Enterococcus casseliflavus. Can J Microbiol. 1998;44:20–27. doi:10.1139/w97-120.
- Speiser B. Food and feeding behaviour. In: Barker GM, editor. The biology of terrestrial molluscs. Wallingford: CAB International; 2001. p. 259–288.
- Foucaud-Scheunemann C, Poquet I. HtrA is a key factor in the response to specific stress conditions in Lactococcus lactis. FEMS Microbiol Lett. 2003;224:53–59. doi:10.1016/S0378-1097(03)00419-1.
- Carr WES. Chemoreception in the mud snail, Nassarius obsoletus. II. Identification of stimulatory substances. Biol Bull. 1967;133:106–127.
- Beimfohr C, Krause A, Amann R, Ludwig W, Schleifer KH. In situ Identification of lactococci, enterococci and streptococci. Syst Appl Microbiol. 1993;16:450–456. doi:10.1016/S0723-2020(11)80279-1.
- Hammes WP, Weiss N, Holzapfel WH. The genera Lactobacillus and Carnobacterium. In: Balows A, Truper HG, Dworkin M, Harder W, Schleifer K-H, editors. The Prokaryotes, a handbook for the biology of bacteria: ecophysiology, isolation, identification. New York: Springer; 1992. p. 1535–1594.
- Kabadjova P, Dousset X, Le Cam V, Prevost H. Differentiation of Closely Related Carnobacterium Food Isolates Based on 16S-23S Ribosomal DNA Intergenic Spacer Region Polymorphism. Appl Environ Microbiol. 2002;68:5358–5366. doi:10.1128/AEM.68.11.5358-5366.2002.
- Song Y, Kato N, Liu C, Matsumiya Y, Kato H, Watanabe K. Rapid identification of 11 human intestinal Lactobacillus species by multiplex PCR assays using group- and species-specific primers derived from the 16S–23S rRNA intergenic spacer region and its flanking 23S rRNA. FEMS Microbiol Lett. 2000;187:167–173. doi:10.1111/j.1574-6968.2000.tb09155.x.
- Cho KM, Math RK, Asraful Islam SMd, Lim WJ, Hong SY, Kim JM, Yun MG, Cho JJ, Yun HD. The genetic diversity analysis of the bacterial community in groundwater by denaturing gradient gel electrophoresis (DGGE). Mol Cell Probe. 2009;23:90–94. doi:10.1016/j.mcp.2008.12.006.
- Jackson CR, Fedorka-Cray PJ, Barrett JB. Use of a Genus- and Species-Specific Multiplex PCR for Identification of Enterococci. J Clin Microbiol. 2004;42:3558–3565. doi:10.1128/JCM.42.8.3558-3565.2004.
- Bensalah F, Flores M-J, Mouats A. A rapid PCR based method to distinguish between Enterococcus species by using degenerate and species-specific sodA gene primers. Afr J Biotechnol. 2006;5:697–702. Available from: http://www.academicjournals.org/AJB
- Yoon JH, Lee ST, Park YH. Inter- and intraspecific phylogenetic analysis of the genus Nocardioides and related taxa based on 16S rRNA gene sequences. Int J Syst Bacteriol. 1998;48:187–194. doi:10.1099/00207713-48-1-187.
- Chowdhury A, Malaker R, Nur Hossain Md, Fakhruddin Md, Noor R. Bacteriocin Profiling of Probiotic Lactobacillus spp. Isolated from Yoghurt. Int J Pharm Chem. 2013;3:50–56. doi:10.7439/ijpc.
- Tagg JR, McGivern AR. Assay system for bacteriocins. Appl Microbiol. 1971;21:943.
- Dubernet S, Desmasures N, Guéguen M. A PCR-based method for identification of lactobacilli at the genus level. FEMS Microbiol Lett. 2002;214:271–275. doi:10.1111/j.1574-6968.2002.tb11358.x.
- Dereeper A, Guignon V, Blanc G, Audic S, Buffet S, Chevenet F, Dufayard JF., Guindon S, Lefort V, Lescot M, Claverie JM, Gascuel O. Phylogeny.fr: robust phylogenetic analysis for the non-specialist. Nucleic Acids Res. 2008;36:W465–469. doi:10.1093/nar/gkn180.
- Dereeper A, Audi S, Claverie JM, Blanc G. Blast-Explorer helps you building datasets for phylogenetic analysis. BMC Evol Biol. 2010;12:10–8. doi:10.1186/1471-2148-10-8.