Abstract
The world's population is increasing very rapidly, reducing the cultivable land of rice, decreasing table water, emerging new diseases and pests, and the climate changes are major issues that must be addressed to researchers to develop sustainable crop varieties with resistance to biotic and abiotic stresses. However, recent scientific discoveries and advances particularly in genetics, genomics and crop physiology have opened up new opportunities to reduce the impact of these stresses which would have been difficult if not impossible as recently as the turn of the century. Marker assisted backcrossing (MABC) is one of the most promising approaches is the use of molecular markers to identify and select genes controlling resistance to those factors. Regarding this, MABC can contribute to develop resistant or high-yielding or quality rice varieties by incorporating a gene of interest into an elite variety which is already well adapted by the farmers. MABC is newly developed efficient tool by which using large population sizes (400 or more plants) for the backcross F1 generations, it is possible to recover the recurrent parent genotype using only two or three backcrosses. So far, many high yielding, biotic and abiotic stresses tolerance, quality and fragrance rice varieties have been developed in rice growing countries through MABC within the shortest timeframe. Nowadays, MABC is being used widely in plant breeding programmes to develop new variety/lines especially in rice. This paper reviews recent literature on some examples of variety/ line development using MABC strategy.
Introduction
Recurrent backcrossing is a traditional breeding method commonly employed to transfer alleles at one or more loci from a donor to an elite variety.[Citation1,Citation2] The expected recurrent parent (RP) genome recovery would be 99.2% by six backcrosses, which is most similar to improved variety. The proportion of the RP genome is recovered at a rate of 1−(1/2) t+1 for each of the generations of backcrossing.[Citation3] However, any specific backcross progeny (BC3 or BC2), they will be deviated during crossing over resulting in a great chance to get the expected result that is not possible to detect phenotypically. For example, in BC1 population, theoretically the average percentage of the RP genome is 75% for the entire population. But some individuals possess more or less of the RP genome than others. Those individuals that contain the highest RP genome are selected. Advancement of genomic research in rice and completion of the rice genome sequence have been developed and it has been possible to detect and map finely a number of genes through linkage to DNA markers. Backcrossing is a widely used technique in rice breeding for introgression or substitution of a target gene from donor parent to recipient. It provides a precise way to improve varieties that excel in a large number of attributes.[Citation1,Citation4,Citation5] The main purpose of backcrossing is to decline the donor genome content into the progenies.[Citation6] Backcross breeding has been adopted in the South and Southeast Asia [Citation7,Citation8] as breeding strategy to improve elite varieties such as KDML105, Basmati and Manawthukha for their resistances to blast.[Citation9] In the meantime, a potential backcrossing approach has been established by applying molecular markers named as simple sequence repeats (SSRs) and single nucleotide polymorphisms. One of the early benefits of molecular markers in plants is to enhance the backcrossing process by reducing the number of backcrosses required to recover the recurrent phenotype.[Citation10] This approach was first reported for rice by Chen et al. [Citation11] They introduced resistance to bacterial blight (BB) disease into Chinese hybrid parents. It was also described for submergence tolerance using the sub1 gene at International Rice Research Institute (IRRI).[Citation12]
Therefore, molecular markers are the tools that can be used to detect the presence of desire character which is economically important. Marker assisted backcrossing (MABC) is an attractive tools for breeding vastly using in a large number of research aim at identifying genomic regions of interest. Molecular markers that are tightly linked with economically important traits have been identified and/or used for MABC in rice including resistance of BB, blast, brown plant hopper, green leaf hopper, gall midge, virus infections, and tolerance to salinity, submergence, drought, cold, semi-dwarf, grain quality and much more. As for molecular marker based research no need of genetic transformation and cultivars are developed by MABC, therefore, there is no contradiction or ethical issues as already raised with transgenic crops. This review focuses on the potential application of MABC for the improvement of rice. This updated information will be a helpful guidance for rice breeders to develop a durable biotic and abiotic stress tolerant rice variety.
The backcross approach
The backcrossing approach was proposed by Harlan and Pope.[Citation13] Since then, backcrossing has become a widely used plant breeding approach in diverse crop species.[Citation5,Citation14,Citation15] This method is most commonly used to incorporate one or a few traits into an adapted or elite variety. In most cases, the elite variety used for backcrossing has a large number of desirable attributes but is deficient in only a few characteristics.[Citation1] The other parent, called the ‘donor parent’, possesses one or more genes controlling an important trait which is lacking in the elite variety. The prime aim of backcross breeding is to transfer one or more genes of interest trait from donor parent into the background of the improved variety and recover the RP genome by eliminating the undesirable genes (linkage drag) as quick as possible. Those unwanted genes transferred through linkage drag cause a negative effect on agronomical traits, such as low yield or disease susceptible. Depending on the linkage distances, the size of the flanking regions can be decreased by additional backcrossing [Citation16] although breeders have not had any direct control over the size of the region or the recombination breakpoints.
Backcross populations are made by crossing the RP with the donor parent to produce an F1 hybrid and then crossing the F1 with the RP to produce the first backcross generation (BC1F1 or just BC1). After phenotypic screening, the next backcross generation is made by crossing selected BC1 plants (that have been screened for the target trait) with the RP to produce the BC2. Subsequent backcross populations are made by repeatedly crossing the selected backcross (BC) plants with the RP. It should be emphasized that backcross progeny with the target trait must be selected based on phenotype during each round of backcrossing. There is no absolute number for how many backcrosses need to be performed but generally between six to eight backcross generations are performed. After the final backcross generation, selected individuals are self-pollinated so that selected lines will be homozygous for the target trait. Typical backcross breeding is shown in . The recessive backcross scheme can also be used for target traits but is difficult to get desired result accurately due to phenotype based on single plants. The end product of a backcrossing programme is to obtain lines that are as similar as possible to the RP but also possess the target traits.
Figure 1. Schematic representation of conventional backcrossing (Modified from George Acquaah [Citation17]).
![Figure 1. Schematic representation of conventional backcrossing (Modified from George Acquaah [Citation17]).](/cms/asset/0365ad88-89cb-4bd8-9b80-178d668031bf/tbeq_a_995920_f0001_oc.jpg)
Essential elements for successful backcross programme
Three main factors are essential to the success of a backcross breeding programme: the selection of the RP, an effective means to screen for the target trait(s) and the number of backcrosses used to reconstitute the RP.[Citation1,Citation18]
Recurrent parent
The choice of the RP must be carefully made based on the factors such as agronomic performance, traits, target environment and popularity with farmers.
Screening for target trait
There must be an effective method for phenotypic screening (i.e. a method which clearly discriminates between segregating progeny) due to the number of rounds of crossing. Traits with high heritability will be more effectively incorporated compared to traits with low heritability.
Number of backcrosses
In the early backcross generations, breeders may visually select which progeny most closely resembles the RP. However at later generations (i.e. after BC2), it may be impossible to discriminate between backcross progeny and the RP based on individual plants. In order to retain the desirable characteristics of the RPs, additional backcrosses must be made on the assumption that additional backcrossing until at least BC6 will restore the RP as much as possible ().
Marker-assisted backcrossing (MABC)
MABC is a precise and an effective method to introgress a single locus controlling a trait of interest while retaining the essential characteristics of the RP.[Citation19] It is known that MABC is effective for genes or quantitative trait loci (QTLs) with large variations in phenotype. MABC is the process of using markers to select for target loci, minimize the length of the donor segment containing a target locus and/or accelerate the recovery of the RP genome during backcrossing.[Citation20,Citation21] The main objective of MABC is to integrate a targeted gene from agronomical substandard sources (the donor parent) into an exclusive breeding line (the RP). The anticipated product is an improved line comprising solely the targeted gene from the donor parent, through the recipient parent line existing all over else in the genome. One of the early benefits envisioned with the use of molecular markers in plants was their use to accelerate the backcrossing process by reducing the number of backcrosses required to recover the RP phenotype.[Citation10]
MABC is superior to conventional backcrossing in precision and efficiency. Background selection can greatly accelerate the backcrossing programme compared to using conventional backcrossing.[Citation22] This approach has been widely used and due to the prevalence of several rice ‘mega varieties’ it is likely to continue being a successful approach.[Citation23] MABC involves successive backcrossing to remove the genetic background of the donor while recovering genetic properties of RPs as much as possible. Statistical methods and schedule of backcrosses to create effective MABC have been reviewed in various published papers.[Citation20,Citation21,Citation24] MABC with marker-based genome scanning has allowed a speedy recovery of most recurrent genome in a few crosses.[Citation25,Citation26] MABC can also be used to develop near isogenic lines (ILs) by minimizing carried-over donor segments flanking the target locus, providing precise introgression of individual genes for detailed characterization of the QTLs. Several applications of marker-assisted backcross breeding have been shown in .
Table 1. Examples of marker-assisted backcross breeding in rice.
The efficiency of marker-assisted backcrossing depends on a number of factors, including the population size of each backcross generation, distance of markers from the target locus and number of background markers used. Data from Hospital [Citation59] showed faster recovery of the RP genome with marker assisted selection (MAS) compared to conventional backcrossing when foreground and background selections are combined (). The RP genome is recovered more slowly on the chromosome carrying the target locus than on other chromosomes because of the difficulty in breaking linkage with the target donor allele. Methods for optimizing sample sizes and selection strategies in marker-assisted selection have been discussed by several researchers [Citation22,Citation25,Citation60,Citation61] ().
Table 2. Expected recovery of recurrent parent genome comparing conventional and marker assisted backcrossing in subsequent generations
The use of this powerful approach for MABC was first reported for rice by Chen et al. [Citation11] introducing resistance to BB disease into Chinese hybrid parents. It was also described for submergence tolerance using the Sub1 gene at IRRI.[Citation12] The basic approach has been described in more detail in several papers by this group.[Citation12,Citation19,Citation45] Using large population sizes (400 or more plants) for the backcross F1 generations, it is possible to recover the RP genotype using only two or three backcrosses. The three selection steps [Citation19] are as follows.
Foreground selection
Foreground selection, in which the breeder selects plants having the marker allele of the donor parent at the target locus. The objective is to maintain the target locus in a heterozygous state (one donor allele and one RP allele) until the final backcross is completed. Then, the selected plants are self-pollinated and progeny plants identified that are homozygous for the donor allele (). Those markers which have already been developed and they are tightly linked to the target gene or QTL should be used to select the target locus of donor parent in early (BC) progenies for the selection of plants that having the target gene. This is referred to as ‘foreground selection’,[Citation21] although referred to ‘positive selection’.[Citation57] Marker-assisted foreground selection was proposed by Tanksley [Citation62] and investigated in the context of introgression of resistance genes by Melchinger.[Citation63] This may be particularly useful for traits that have laborious or time-consuming phenotypic screening procedures. It can also be used to select for reproductive-stage traits in the seedling stage, allowing the best plants to be identified for backcrossing.
Figure 2. Schematic representation of selection of homozygous plants for the donor allele. Source: modified from IRRI, (2014) with permission (www.knowledgebank.irri.org).
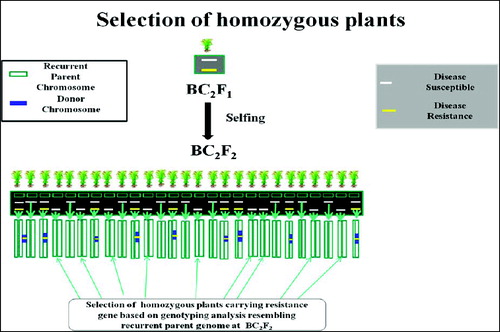
Recombinant selection
The second level involves selecting BC progeny with the target gene and recombination events between the target locus and linked flanking markers is termed as ‘recombinant selection’.[Citation19] The purpose of recombinant selection is to reduce the size of the donor chromosome segment containing the target locus (i.e. size of the introgression). This is important because the rate of decrease of this donor fragment is slower than for unlinked regions and many undesirable genes that negatively affect crop performance may be linked to the target gene from the donor parent (i.e. as linkage drag, ).[Citation15] Using conventional breeding methods, the donor segment can remain very large even with many backcross generations (e.g. >10 cM; [Citation64,Citation65]. By using markers that flank a target gene (e.g. <5 cM) on either side), linkage drag can be minimized. Since double recombination events occurring on both sides of a target locus are extremely rare, recombinant selection is usually performed using at least two BC generations.[Citation25] It must be emphasized that this is only possible for genes or QTLs for which the map position has been well defined. Fine mapping (or high-resolution mapping) is usually required prior to recombinant selection. Furthermore, recombinant selection can minimize the size of the donor chromosome segment, thus reducing ‘linkage drag’ – a ‘universal enemy’ of the plant breeder.[Citation20]
Figure 3. Schematic representation of transferring undesirable genes with target gene. Source: modified from IRRI, (2014) with permission (www.knowledgebank.irri.org).
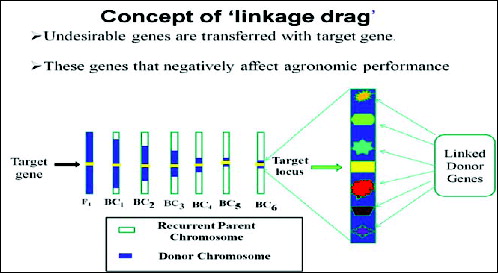
Background Selection
Except target locus, all genomic regions can be selected in background selection using RP marker alleles and the selection of target locus is done on the basis of phenotype. This selection is important in order to reduce unnecessary genes (linkage drag, ) introduced from donor. By using molecular markers, it is easy to delete the unwanted donor alleles in the same genomic region as the target locus.
The third level of MABC involves selecting BC progeny with the greatest proportion of RP genome, using markers that are unlinked to the target locus – we refer to this as ‘background selection’. In the literature, background selection refers to the use of tightly linked flanking markers for recombinant selection and unlinked markers to select RP.[Citation21,Citation25] This was also referred to as ‘negative selection’ by Takeuchi et al. [Citation57] Background markers are markers that are unlinked to the target gene/QTL on all other chromosomes, in other words, markers that can be used to select against the donor genome. This is extremely useful because the RP recovery can be greatly accelerated ( and ). With conventional backcrossing, it takes a minimum of six BC generations to recover the RP and there may still be several donor chromosome fragments unlinked to the target gene. The use of background selection during MABC to accelerate the development of an RP with an additional one or more genes has been referred to as ‘variety development or enhancement’ [Citation45] and ‘complete line conversion’ ().[Citation66]
Figure 4. Schematic representation of selection of heterozygous carrying resistance gene based on genotyping analysis resembling RP genome at BC1F1. Source: modified from IRRI, (2014) with permission (www.knowledgebank.irri.org).
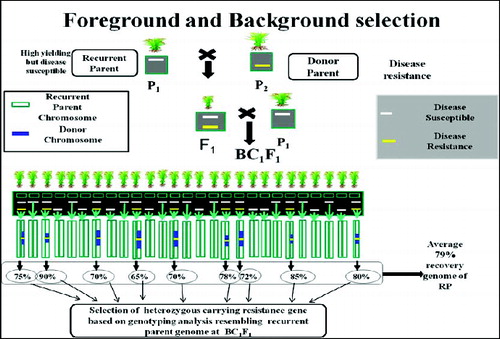
Figure 5. Schematic representation of selection of heterozygous carrying resistance gene based on genotyping analysis resembling RP genome at BC2F1. Source: modified from IRRI, (2014) with permission (www.knowledgebank.irri.org).
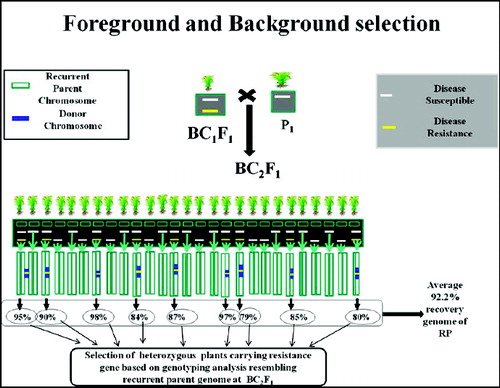
Figure 6. Schematic representation of development of resistant rice variety through marker-assisted backcrossing (MABC). Source: modified from Babu et al. [Citation67].
![Figure 6. Schematic representation of development of resistant rice variety through marker-assisted backcrossing (MABC). Source: modified from Babu et al. [Citation67].](/cms/asset/93aad94b-6342-4462-a794-aaa441a9697a/tbeq_a_995920_f0006_b.gif)
MABC has several advantages over conventional backcrossing
Speed up recovery of recurrent parent genome
Conventional backcross breeding needs six backcrosses to recover the RP genome. But RP genome may be recovered by BC4 or BC3 even BC2 using MABC approach,[Citation21,Citation22,Citation25,Citation68] thus saving two to four BC generations ().
Figure 7. Schematic representation of difference between conventional backcrossing and marker-assisted backcrossing. Source: modified from IRRI, (2014) with permission (www.knowledgebank.irri.org).
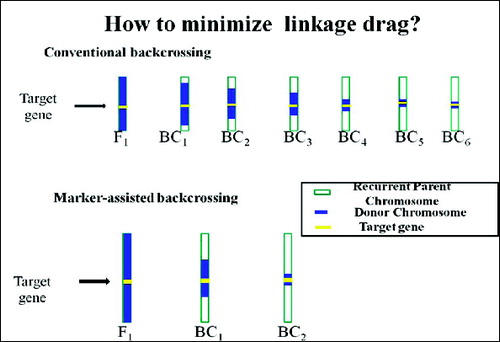
Consistency
Environmental factors are of great concern and may hamper the expression of plant characteristics. But molecular markers are consistent from any significant impact of environmental stresses, which presents great opportunities for selecting molecular markers for MABC.
Minimize the linkage drag
To reduce the large number of donor chromosome, minimum six backcross generations are needed whether MABC may need two or three backcross generations. Linkage drag requires many additional backcross generations, and if the undesirable genes are really tightly linked to the target locus it may be difficult to eliminate these genes using conventional backcrossing.[Citation19]
Biosafety
Without inoculation of pathogen, the definite characters for disease resistance can be conducted by molecular markers which are tightly linked with the target gene. They also facilitate introgression of genes into high-yielding variety that are disease susceptible.
Efficiency
By sorting of breeding lines in few generations with the application of molecular markers, it is easy to discard all the progenies from the programme except our targeted lines.
More accurate selection
By applying conventional breeding techniques, it is very difficult to identify polygenic characters. But in the case of MABC, it is possible to select on the basis of gene expression using markers.
Marker-assisted backcrossing in biotic stresses
Blast resistance
Recently, Pusa1602 (PRR78+Piz5) and Pusa1603(PRR78+Piz54) lines have been developed through incorporation of blast resistance genes Piz-5 and Pi54 derived from donor lines C101A51 and Tetep into the background of PRR78 (highly blast susceptible) through MABC breeding strategy.[Citation40] Foreground selection for the genes Piz-5 and Pi54 were effected using tightly linked molecular markers, AP5930 and RM206, respectively in two independent backcross series. Background analysis revealed the RP genome recovery up to 89.01% and 87.88% in Pusa1602 and Pusa1603 lines, respectively. The hybrids produced by crossing Pusa6A with improved lines of PRR78, were on par with original Pusa RH10 in terms of yield, grain and cooking quality traits with an added advantage of blast resistance.
Leaf blast resistance line D521, neck blast resistance line D524 and BB resistance have been developed through introgression of leaf resistance gene Pi1, neck blast resistance gene Pi2 derived from donor BL122 and bacterial resistance gene Xa23 derived from donor CBB23 into an elite, early maturing maintainer line of hybrid rice disease susceptible to both blast and blight, Ronfeng B hybrid rice through marker-assisted backcross breeding programmes.[Citation41] By using three SSR markers (MRG4766, AP22 and RM206), Pi1, Pi2 and Xa23 have been identified and also used to test the recovery of the genetic background for the improved lines by using 131 polymorphic markers. After four backcrosses, the recovery ratios of the recurrent-parental genome at non-target loci for D521 and D524 were 96.18% and 96.56%, respectively. The resistance frequencies for the rice blast and the length of lesions resulting from BB ranged from 96.7% to 100% and 0.77 to 1.18 cm, respectively. A new cytoplasmic male sterile line, Rongfeng 3A, with Pi1, Pi2 and Xa23, was successfully developed through successive backcross breeding. MABC has been successfully utilized for developing elite parental lines of hybrid rice with inbuilt resistance to BB and blast diseases.[Citation69–71] The hybrid Pusa RH10 is more susceptible to BB and blast. Pusa RH10 would improve its adaptability to disease endemic region and also sustain the productivity of the rice by incorporating the bacterial and blast resistance gene. In order to detect QTLs controlling blast resistance, mapping population of 192 F2:3 families derived from the cross of two Iranian rice varieties Tarom Mahali (TAM), blast susceptible cultivar and the blast resistance cultivar cul, Khazar (KHZ) were developed. By using 74 polymorphic markers, two parental lines TAM and KHZ showed high genetic variability between the two varieties on chromosomes 1, 2, 3, 4, 5, 9, 11 and 10.[Citation72]
Bacterial blight resistance
A novel BB resistance gene, Xa23, identified from Oryza rufipogon was introgressed into three popular restorer lines (Minghui63, YR293 and Y1671) for wild abortive cytoplasmic male sterility by marker-assisted backcross breeding approach in combination with artificial inoculation and stringent phenotypic selections. Foreground selection against Xa23 gene was used to select plants carrying Xa23 gene prior to transplanting through MAS using a closely linked SSR marker (RM206) of 1.9 cm apart from the Xa23 locus. [Citation73] The newly developed BB resistant restorers and their derived hybrids were identical to their respective original versions for agronomic traits especially under disease free condition.[Citation71] In the Philippines, the gene combination of Xa4+Xa5+Xa21 derived from two rice cultivars (NSIC Rc142 and NSIC Rc154) for blight resistance have been integrated into the susceptible cultivar IR64 genetic background using MABC.[Citation35] Recently, it has been successfully incorporated two genes for BB resistance namely, Xa13 and Xa21 in the restorer line PRR78 using MABC.[Citation70]
In lowland rice in Thailand, BB resistance gene Xa21 obtained from rice variety IR1188 was introgressed into popular variety KDML105 through three rounds of MABC and phenotypic selection. The developed introgression lines carrying BB resistant gene (Xa21) have showed the characteristics identical to the local variety KDML105 resulted from different locational tests at research centre in lowland rice cultivation area.[Citation29] In their study, three seedling resistance loci inherited from IR1188 were identified on rice chromosomes 1 (RM302, RM212), 8 (RM210, RM149) and 11 (RM287, RM224). Sixty-seven rice microsatellite marker (SSR) well distributed over the whole rice genome, together with markers that are associated with the cooking characteristics of KDML105, were used to determine the background genotype of the Koshihikari backcross inbred lines (KBILs).
In China, BB resistance genes Xa7 and Xa21 derived from Huahui20 have been introgressed into the popular restorer line, Yihui1577 using the MABC approach.[Citation30] The genotype of each BC progenies was determined using tightly linked SSR marker, RM20582 in the presence of Xa7 to map a 0.14 cM interval between the markers RM20582 and RM20593 on chromosome 6.[Citation74] At BC3F1 generation, plants were selfed to produce BC3F2 population. Homozygous plants carrying Xa7, Xa21 and both of them were selected in BC3F2 to produce BC3F3 families. In China, a successful introgression of four BB resistance genes (Xa7, Xa21, Xa22 and Xa23) derived from cytoplasmic male sterile line Jinke 1A, was done into an elite hybrid rice restorer line Huahui 1035 through MABC.[Citation31] Result revealed that three promising high-yielding F1 hybrid lines were selected for hybrid rice production in China. Two BB resistance genes Xa21 and Xa13 obtained from an elite high-yielding, fine grain-type variety, Samba Mashuri has been introgressed into BB susceptible two elite varieties, Taraori Basmati and Basmati 386 by using the MABC programme.[Citation27]
Gall midge resistance
Rice gall midge resistance gene Gm8 achieved from donor parent Aganni, has been introgressed into an improved variety Samba Mashuri carrying gene Xa21 through MABC. Results revealed that in BC2F2, four plants were tested and Gm8 and Xa21 genes were identified. In F3 progeny, these plants were phenotyped for resistance to BB and gall midge.[Citation53] Breeding for gall midge resistance has been one of the most successful stories of modern crop improvement. However, rapid evolution of virulent biotypes against the resistant rice varieties carrying a single major gene during the 1980s and thereafter [Citation75] has called for a rethink of the breeding approach. So far, eleven resistance genes in the plant [Citation76] and seven biotypes of the pest have been reported.[Citation77]
Virus resistance
An RNA interference construct (ORF IV of RTBV, placed both in sense and anti-sense orientation under CaMV 35S promoter) was transferred to two high-yielding Tungro-susceptible indica rice cultivars (IET4094 and IET4786) from the transgenic Pusa Basmati (PB-1) scented rice line using backcross breeding till the BC2F3 stage and the progenies (BC2F1) showed mild symptoms of Tungro, in contrast to severe symptoms displayed by the RPs.[Citation78] In another research, two QTLs were derived from an upland resistant japonica variety ‘Azucena’ which were partial resistance to Rice yellow mottle virus (RYMV) (located on chromosome 12 and 7) have been introgressed into a lowland susceptible indica variety ‘IR64’ using MABC.[Citation79] In their study, the valuation of RYMV was done in F2 and F3 progenies of BC1 and BC2 generations. In BC3 progeny, it was the highest recovery of the RP genome i.e. 95% for the 10 non-carrier chromosomes.
Brown plant hopper resistance
Brown plant hopper resistant gene bph3 derived from donor cultivar ‘Rathu Heenati 'has been transferred into the most popular Thai rice variety 'Khao Dawk Mali 105’ (KDML105).[Citation80] In this scheme, two SSR markers, RM589 and RM190, closely linked to Bph3 and Wx-RH loci, respectively, were used to identify the genotype of BC3F2 individuals. Only progenies carrying heterozygous genotypes at the Wx-RH and Bph3 region were selected to generate BC3F3. A total of 75 polymorphic SSR markers distributed throughout rice genome were then used to determine the recurrent genetic background of the 50 selected ILs from the BC3F4 generation.
Recently the Bph18 gene from donor indica line IR65482-7-216-1-2 has been successfully transferred to Junambyeo, an elite japonica cultivar, by MAS and several generations of backcrossing.[Citation52] The most tightly linked co-dominant sequence-tagged site (STS) marker, 7312T4A was used to detect the presence of the Bph18 gene in backcross-derived BPH-resistant breeding lines.[Citation81] Some 260 SSR markers of known chromosomal positions distributed evenly on the 12 chromosomes with an average interval of 5.9cM were used in a genome-wide survey to identify the chromosome segment substitution locations in the four advanced backcross breeding lines compared with the donor line. The SSR markers polymorphic between the two parents were used for background genotyping to recover the recipient parent genome.
Marker-assisted backcrossing in abiotic stresses
Submergence tolerance
Submergence sub1 gene at the locus RM23805 derived from IR64 was incorporated into susceptible variety OM1490. The band corresponds to an allele from susceptible parent OM1490 and tolerant one IR64-Sub1 as 240 and 230 bp bands, respectively, at the locus RM23805.[Citation44] sub1A gene has been introgressed into a popular high-yielding variety from India, Swarna following MABC procedure within 2 years.[Citation12] In Vietnam, successful introgression sub1 derived from donor rice variety IR64, has been done into popular rice variety AS996 through MABC. The introgression of sub1 was confirmed using ART5 and SC3.[Citation43] Parental diversity was carried out with 460 markers, 53 polymorphic markers of which were used for assessment on BC1F1, BC2F1 and BC3F1 generations having recipient allele 87.5%, 93.75% and 96.15%, respectively. In BC4F1, there was the highest genetic background i.e. 100%. All mega varieties (Samba Mahsuri and CR1009 from India, IR64 from the Philippines (IRRI), Thadokkham 1 (TDK1) from Laos and BR11 from Bangladesh with sub1 introgression had a significantly higher survival rate than the original parents by using the MABC strategy. The tolerance level of an intolerant sub1C allele combined with the tolerant sub1A-1 allele did not significantly reduce and the expression of sub1C-1 was independent over the sub1A allele. Plants remained intolerant when sub1C-1 expression was completely turned off in the presence of sub1A-2. Survival rates and sub1A expression were significantly lower in heterozygotes compared with the homozygous tolerant parent.[Citation82]
Salt tolerance
Very recently in Vietnam, the Saltol QTL obtained from the highly salt tolerant rice variety FL478 has been transferred into the high-yielding and widely grown cultivars, ASS996 by following the MABC strategy.[Citation47] In this study, in each backcross generations, AP3206, RM3412 and RM10793 were used for screening heterozygous plants and 63 polymorphic markers were used to be distributed on 12 chromosomes. Two plants P284 and P307 had the highest recipient alleles up to 89.06% and 86.36% were used to develop BC2F1 populations. The recombinant selection was done with RM10694, RM562, RM7075 along the Saltol region on chromosome 1. Plant P284-112-209 was the best BC3F1 individual with all the recipient alleles screened based on a total of 63 markers. The four plants P307-305-21, P284-112- 195, P284-112-198 and P284-112-213 were the second ranking with only one loci heterozygous. All those five plants were chosen as the breeding lines for result of Saltol-AS996 introgression.
Saltol QTL derived from FL478 was introgressed in genetic background of Bacthom 7 cultivar. The background analysis in the introgression line revealed the recovery up to 96.8%–100% of RP alleles based on the screened markers after three generations.[Citation48] In this study, 8 markers were used to identify Saltol locus and 81 markers were used in other loci between the parents. Then, 88 markers were applied to analyse genotyping of each backcross generation with the three steps of selection (foreground, recombinant and background). The results revealed that the best plant of BC3F1 generation bear the highest recovery of recipient genome i.e. 96.8%–100%. This study revealed that the introgression lines can be directly developed into the salinity tolerance variety, which is suitable for cultivating in coastal areas of the Vietnamese Deltas using MABC.
Drought tolerance
Near isogenic lines (NILs) were developed by introgression of three root QTLs from CT9993, an upland japonica into IR20, a lowland indica cultivar through MABC procedure.[Citation49] Among the NILs considerable, variation in drought response and grain yield under rain-fed condition in target populations was monitored. Among the 41 NILs, only 5 showed high yield permanence in both rain-fed and irrigated conditions compared to the IR20. Two NILs namely, 212 and 297, with three and two root QTLs, respectively had thicker and longer nodal roots and higher total and deep nodal roots weight than IR20. In addition, NIL 297 had higher nodal root volume and surface area, while NIL 212 had increased number of nodal roots compared to IR20. QTLs for drought tolerant traits were transferred into Thai variety KMDL105 by following MABC programme. The backcrossing with target selection resulted in 103 KMDL105 introgression lines carrying 1, 2 or 3 target combinations, where 79, 20 and 4 lines were derived from KDML105 x IR68586-F2-CA-143 (DH212) (cross 1), KDML105 x IR68586-F2-CA-31 (DH103) (cross 2) and KDML105 x IR68586-F2-CA-54 (DH126) (cross 3) crosses, respectively. Genome scanning revealed that carrier chromosomes in all crosses showed a low percentage of the recipient parent genome. Also, non-carrier chromosomes, especially in crosses 1 and 2, were found to carry segments of the donor, which was reflected in the low percentage of the recipient genome of KDML105 in all crosses.[Citation83]
Phosphorus tolerance
Pup1 has been introgressed into two irrigated rice varieties and three Indonesian upland varieties by using MABC approach. The first assessment of phenotypic of introgression lines suggests that Pup1 is effective in different genetic backgrounds and environments and it has the potential to increase significantly the grain yield under field conditions.[Citation50]
Grain fragrance
A molecular marker for grain aroma was first observed by Ahn et al [Citation84]. The genomic clone RG28, a restriction fragment length polymorphism (RFLP) marker, was tightly linked to the fragrance locus in rice chromosome 8 at a genetic interval of 4.5 cM.[Citation85] RG28 can be changed by sequencing into a STS marker and utilized as a marker for the polymerase chain reaction (PCR) of genomic DNA from rice varieties differing in their aroma.[Citation86] Using this locus, different PCR-based markers were developed that can discriminate between aromatic and non-aromatic rice cultivars.[Citation87,Citation88] MABC has been successfully used for the improvement of aromatic rice. The quality traits of the Manawthukha cultivar were promoted by MABC, using Basmati 370 as a donor [Citation89,Citation90] suggested the progress of improved Pusa Basmati 1 and the improved versions of PRR78 successfully utilizing MABC.
Semi-dwarfing
The rice semi-dwarfing gene, sd1, has been studied intensively due to its contribution to the increase of crop production. Although sd1 breeding was extensively applied since the 1960s, the recent advances in the molecular basis of this gene allowed designing a more precise breeding strategy – MABC – to track sd1 introgression in two traditional rice varieties. MABC and background selection revealed as useful tools to assist breeding for semi-dwarfism in traditional rice varieties (japonica).[Citation91]
Cost effective of MABC programme
There are many factors like inheritance of the trait, method of phenotypic evaluation, field/ glasshouse and labour costs, and the cost of resources influence the cost of utilizing MABC. In some case, MABC is not much cheaper than phenotypic screening.[Citation92,Citation93] But in other cases, phenotypic evaluation is more time consuming and difficult. Using markers, may be cheaper and preferable.[Citation93–95] From the simulation studies, in some cases, MABC has the ability to improve selection efficiency over phenotypic selection in breeding programmes.[Citation21,Citation96–98] Factors that influence the cost of utilizing MABC or MAS include inheritance of the trait, method of phenotypic evaluation, field/glasshouse and labour costs, and the cost of resources. By using DNA markers, the evaluation of backcross progenies may be expensive. Before starting MABC programme, an approximate cost estimate should be calculated. To minimize the costs, the protocols of marker genotyping should also be standardized and optimized. Initially the cost of MABC would be more expensive compared to the conventional breeding in short-term, but it would be economically benefitted by saving time. This conception is very much an important consideration for our plant breeders, because the accelerated release of an improved variety may translate into more rapid profits by the release of new cultivars as soon as possible.[Citation99] Estimates of cost including consumable and labor per data point for rice marker genotyping during MAS is US$ 0.30 to 1.00.[Citation19]
Conclusion and future prospects
With newer technologies and through advances in the field of genomics, the challenge for plant breeders is to judiciously utilize these novel tools in molecular marker-assisted breeding for developing commercially viable improved cultivars which address specific problems in different crops. This can be made possible only when MAS is integrated into traditional breeding practices, rather than being considered as a substitute. Plant breeding keeps a great contribution in the rice production and balancing the food security year after year. It is a fact that rice researchers faced many difficulties for doing their research due to global warming and climate change. As a result, various types of new diseases and insects, and several biotic and abiotic stresses occur, which often decreases the rice yield. In this aspect, advances in rice biotechnology and genomics have paved the way to meeting the challenges and new genes for resistance to biotic and abiotic stress and MABC has already proven to transfer major genes into elite rice parents, using both foreground and background selection. Now MABC is more popular to rice researchers as a potential and simple technique, because the major benefit of the MABC technique is to use the varieties that are already well accepted by farmers so that the improved variety will be ensured of possessing the target traits prized by the farmers. In rice, the existence of popular varieties that are already grown offers the opportunity to use this approach with new traits. In addition, the MABC approach plays vital role for basic research applications in rice to develop new varieties with much greater precision than conventional backcrossing.
MABC has generated a good deal of expectations, which in some cases led to over-optimism and in others to disappointment, because many of the expectations have not yet been realized. Although there is the consideration of bright future possibilities and potentials effects of MABC, there are also some constrains to its use, including equipment, infrastructure, skilled manpower, poor private sector involvement, supplies or consumables. If we consider the financial support in agricultural sector in many developing countries, their development priorities are not included for genetic enrichment programmes using molecular tools. Various stages in the MABC development and application process were regarded as being costly. The most significant cost prior to MABC is the development of a genetic linkage map for the species of interest and identification of associations between genes or QTLs and economically important traits. Such cost could be significantly high for developing countries. In developing countries, in order to take up breeders, the returns to investments should be far superior compared with those in developed countries, given the significant opportunity costs and various constraints associated with availability of facilities and supplies. We expect that the cost of MABC can be decreased, resources pooled and shared and a capacity to be developed if we make good partnership between developing and developed countries, including public–private sector collaboration. Currently, the cost of utilizing markers is possibly the most important factor that limits the implementation of MABC. Therefore, new marker technology can potentially reduce the cost of MAS considerably. If the effectiveness of the new methods is validated and the equipment can be easily obtained, this should allow MABC to become more widely applicable for crop breeding programmes, especially in rice. An efficient cost-effective MABC technology must be developed that will allow breeders to assess the genotype across the full genome and to recombine genes of agronomic importance from diverse sources.
Disclosure statement
The authors declare that they have no conflict of interests concerning this paper.
Additional information
Funding
References
- Allard RW. Principles of plant breeding. New York, NY: John Wiley & Sons Inc.; 1960.
- Reyes-Valde´s MH. A model for marker-based selection in gene introgression breeding programs. Crop Sci. 2000;40:91–98.
- Babu R, Nair SK, Prasanna BM, Gupta HS. Integrating marker assisted selection in crop breeding – prospects and challenges. Curr Sci. 2004;87:607–619.
- Miah G, Rafii MY, Ismail MR, Puteh AB, Rahim HA, Asfaliza R, Latif MA. Blast resistance in rice: a review of conventional breeding to molecular approaches. Mol Biol Rep. 2013;40:2369–2388.
- Allard RW. Principles of plant breeding. 2nd ed. New York, NY: Wiley; 1999.
- Xi ZY, He FH, Zeng RZ, Zhang ZM, Ding XH, Li WT, Zhang GQ. Development of a wide population of chromosome single-segment substitution lines in the genetic background of an elite cultivar of rice (Oryza sativa L.). Genome. 2008;49(5):476–484.
- Joseph M, Gopalakrishnan S, Sharma RK, Singh VP, Singh AK, Mohapatra T. Combining bacterial blight resistance and Basmati quality characteristics by phenotypic and molecular marker-assisted selection in rice. Mol Breed. 2004;13:377–387.
- Toojinda T, Tragoonrung S, Vanavichit A, Siangliw JL, Pa-In N, Jantaboon J, Siangliw M, Fukai S. Molecular breeding for rainfed lowland rice in the Mekong region. Plant Prod Sci. 2005;8:330–333.
- Sreewongchai T, Toojinda T, Thanintorn N, Kosawang C, Vanavichit A, Tharreau D, Sirithunya P. Development of elite indica rice lines with wide spectrum of resistance to Thai blast isolates by pyramiding multiple resistance QTLs. Plant Breed. 2010;129:176–180.
- Tanksley SD, Young ND, Patterson AH, Bonierbale MW. RFLP mapping in plant breeding: new tools for an old science. Nat Biotechnol. 1989;7:257–263.
- Chen S, Lin XH, Xu CG, Zhang QF. Improvement of bacterial blight resistance of ‘Minghui 63’, an elite restorer line of hybrid rice, by molecular marker-assisted selection. Crop Sci. 2000;40:239–244.
- Neeraja CN, Maghirang-Rodriguez R, Pamplona A, Heuer S, Collard BCY, Septiningsih EM, Vergara G, Sanchez, D, Xu K, Ismail AM, Mackill DJ. A marker-assisted backcross approach for developing submergence-tolerant rice cultivars. Theor Appl Genet. 2007;115:767–776.
- Harlan HV, Pope MN. The use and value of back-crosses in small-grain breeding. J Hered. 1922;13:319–322.
- Stoskopf NC, Tomes DT, Christie BR. Plant breeding: theory and practice. San Francisco, CA; Oxford: Westview Press Inc.; 1993.
- Hospital F. Selection in backcross programmes. Philosophical Trans Royal Soc B. 2005;360:1503–1511.
- Young ND, Zami D, Ganal MW, Tanksley SD. Use of isogenic lines and simultaneous probing to identify DNA markers tightly linked to the Tm-2a gene in tomato. Genetics. 1988;120:579–585.
- Acquaah G. Principles of plant genetics and breeding. Oxford, UK: Blackwell Publishing; 2007.
- Briggs FN, Allard RW. The current status of backcross method of plant breeding. Agronomy J. 1953;45:131–138.
- Collard BCY, Mackill, DJ. Marker-assisted selection: an approach for precision plant breeding in the twenty-first century. Philosophical Trans Royal Soc B. 2007;363:557–572.
- Hospital F. Size of donor chromosome segments around introgressed loci and reduction of linkage drag in marker-assisted backcross programs. Genetics. 2011;158(3):1363–1379.
- Hospital F, Charcosset A. Marker-assisted introgression of quantitative trait loci. Genetics. 1997;147:1469–1485.
- Frisch M, Bohn M, Melchinger AE. Comparison of selection strategies for marker-assisted backcrossing of a gene. Crop Sci. 1999a;39:1295–1301.
- Mackill DJ, Collard BCY, Neeraja CN, Rodriguez RM, Heuer S, Ismail AM. QTLs in rice breeding: examples for abiotic stresses. In: Brar DS, Mackill DJ, Hardy B, editors. Proceedings of the 5th International Rice Genetics Symposium; 2005 November 19–23; International Rice Research Institute, Manila, Philippines. Singapore: World Scientific Publishing.
- Herzog E, Frisch M. Selection strategies for marker-assisted backcrossing with high-throughput marker systems. Theor Appl Genet. 2011;123:251–260.
- Frisch M, Bohn M, Melchinger AE. Minimum sample size and optimal positioning of flanking markers in marker-assisted backcrossing for transfer of a target gene. Crop Sci. 1999b;39:967–975.
- Frisch M, Melchinger AE. Selection theory for marker-assisted backcrossing. Genetics. 2005;170:909–917.
- Pandey MK, Rani NS, Sundaram RM, Laha GS, Madhav MS, Rao KS, Sudharshan I, Hari Y, Varaprasad GS, Rao LVS, Suneetha K, Sivaranjani AKP, Viraktamath BC. Improvement of two traditional Basmati rice varieties for bacterial blight resistance and plant stature through morphological and marker-assisted selection. Mol Breed. 2013;31:239–246.
- Guvvala L D, Koradi P, Shenoy V, Marella LS. Improvement of resistance to bacterial blight through marker assisted backcross breeding and field validation in rice (Oryza sativa). Res J Biol. 2013;1:52–66.
- Win KM, Korinsak S, Jantaboon J, Siangliw M, Lanceras-Siangliw J, Sirithunya P, Vanavichit A, Pantuwan G, Jongdee B, Sidhiwong N, Toojinda T. Breeding the Thai jasmine rice variety KDML105 for non-age-related broad-spectrum resistance to bacterial blight disease based on combined marker-assisted and phenotypic selection. Field Crops Res. 2012;137:186–194.
- Xu J, Jiang J, Dong X, Ali J, Mou, T. Introgression of bacterial blight (BB) resistance genes Xa7 and Xa21 into popular restorer line and their hybrids by molecular marker-assisted backcross (MABC) selection scheme. Afr J Biotechnol. 2012;11(33):8225–8233.
- Huang B, Xu JY, Hou MS, Ali J, Mou TM. Introgression of bacterial blight resistance genes Xa7, Xa21, Xa22 and Xa23 into hybrid rice restorer lines by molecular marker-assisted selection. Euphytica. 2012;187:449–459.
- Ronald PC, Albano B, Tabien R, Abenes MLP, Wu KS, McCouch SR, Tanksley SD. Genetic and physical analysis of the rice bacterial blight disease resistance locus Xa-21. Mol Gen Genet. 1992;236:113–120.
- Singh S, Sidhu JS, Huang N, Vikal Y, Li Z, Brar DS, Dhaliwal HS, Khush GS. Pyramiding three bacterial blight resistance genes (xa5, xa13 and Xa21) using marker-assisted selection into indica rice cultivar PR106. Theor Appl Genet. 2001;102:1011–1015.
- Sanchez AC, Brar DS, Huang N, Li Z, Khush GS. Sequence Tagged site marker-assisted selection for three bacterial blight resistance genes inrRice. Crop Sci. 2000;40:792–797.
- Toenniessen GH, O'Toole JC, DeVries J. Advances in plant biotechnology and its adoption in developing countries. Curr Opin Plant Biol. 2003;6:191–198.
- Sundaram RM, Vishnupriya MR, Biradar SK, Laha GS, Reddy AG, Rani NS, Sarma NP, Sonti RV. Marker assisted introgression of bacterial blight resistance in Samba Mahsuri, an elite indica rice variety. Euphytica. 2008;160:411–422.
- Sundaram RM, Priya MRV, Laha GS, Rani NS, Rao PS, Balachandran SM, Reddy GA, Sarma NP, Sonti RV. Introduction of bacterial blight resistance into Triguna, a high yielding, mid-early duration rice variety by molecular marker assisted breeding. Biotechnol J. 2009;4:400–407.
- Gopalkrishnan RK, Sharma, Rajkumar AK, Josheph M, Singh VP, Singh AK, Bhat KV, Singh NK, Mohapatra T. Integrating marker assisted background analysis with foreground selection for identification of superior bacterial blight resistant recombinants in Basmati rice. Plant Breed. 2008;127:131–139.
- Pinta W, Toojinda T, Thummabenjapone P, Sanitchon J. Pyramiding of blast and bacterial leaf blight resistance genes into rice cultivar RD6 using marker assisted selection. Afr J Biotechnol. 2013;12(28):4432–4438.
- Singh VK, Singh A, Singh SP, Ellur RK, Choudhary V, Sarkel S, Singh D, Krishnan SG, Nagarajan M, Vinod KK, Singh UD, Rathore R, Prashanthi Sk, Agrawal PK, Bhatt JC, Mohapatra T, Prabhu KV, Singh AK. Incorporation of blast resistance into “PRR78”, an elite Basmati rice restorer line, through marker assisted backcross breeding. Field Crops Res. 2012;128:8–16.
- Fu C, Wu T, Liu W, Wang F, Li J, Zhu X, Huang H, Liu ZR, Liao Y, Zhu M, Chen J, Huang Y. Genetic improvement of resistance to blast and bacterial blight of the elite maintainer line Rongfeng B in hybrid rice (Oryza sativa L.) by using marker-assisted selection. Afr J Biotechnol. 2012;11(67):13104–13124.
- Liu DW, Oard SV, Oard JH. High transgene expression levels in sugarcane (Saccharum officinarurn L) driven by rice ubiquitin promoter RUBQ2. Plant Sci. 2003;165:743–750.
- Cuc LM, Huyen LTN, Hien PTM, Hang VTT, Dam NQ, Mui PT, Quang VD, Ismail AM, Ham LH. Application of marker assisted backcrossing to introgress the submergence tolerance QTL SUB1 into the Vietnam elite rice variety-AS996. Am J Plant Sci. 2012;3:528–536.
- Lang N T, Tao NV, Buu BC. Marker-assisted backcrossing (mab) for rice submegence tolerance in Mekong delta. Omonrice. 2011;18:11–21.
- Mackill DJ. Breeding for resistance to abiotic stresses in rice: the value of quantitative trait loci. In: K.R. Lamkey and M. Lee, editors. Plant breeding: the Arnel R. Hallauer international symposium. Ames, IA: Blackwell; 2006. p. 201–212.
- Huyen LTN, Cuc LM, Ham LH, Khanh TD. Introgression the SALTOL QTL into Q5DB, the elite variety of Vietnam using marker assisted selection (MAS). Am J BioSci. 2013;1(4):80–84.
- Huyen LTN, Cuc LM, Ismail AM, Ham LH. Introgression the salinity tolerance QTLs Saltol into AS996, the elite rice variety of Vietnam. Am J Plant Sci. 2012;3:981–987.
- Vu HTT, Le DD, Ismail AM, Le HH. Marker-assisted backcrossing (MABC) for improved salinity tolerance in rice (Oryza sativa L.) to cope with climate change in Vietnam. Aust J Crop Sci. 2012;6(12):1649–1654.
- Suji KK, Prince KSJ, Mankhar PS, Kanagaraj P, Poornima R, Amutha K, Kavitha S, Biji KR, Gomez M, Babu RC. Evaluation of rice (Oryza sativa L.) near iso-genic lines with root QTLs for plant production and root traits in rainfed target populations of environment. Field Crops Res. 2012;137:89–96.
- Chin JH, Gamuyao R, Dalid C, Bustamam M, Prasetiyono J, Moeljopawiro S, Wissuwa M, Heuer S. Developing rice with high yield under phosphorus deficiency: Pup1 sequence to application. Plant Physiol. 2011;156:1202–1216.
- Xu J. Pyramiding of two BPH resistance genes and Stv-bi gene using marker-assisted selection in japonica rice. Crop Breed Appl Biotechnol. 2013;13:99–106.
- Suh JP, Yang SJ, Jeung JU, Pamplona A, Kim JJ, Lee JH, Hong HC, Yang CI, Kim YG, Jena KK. Development of elite breeding lines conferring Bph18 gene-derived resistance to brown plant hopper (BPH) by marker-assisted selection and genome-wide background analysis in japonica rice (Oryza sativa L.). Field Crops Res. 2011;120:215–222.
- Sama VSAK, Himabindu K, Naik SB, Sundaram RM, Viraktamath BC, Bentur JS. Mapping and marker-assisted breeding of a gene allelic to the major Asian rice gall midge resistance gene Gm8. Euphytica. 2012;187:393–400.
- Nas TMS, Sanches DL, Diaz GQ, Mendioro MS, Virmani SS. Pyramiding of thermosensitive genetic male sterility (TGMS) genes and identification of a candidate tms5 gene in rice. Euphytica. 2005;145:67–75.
- Shen ZK, Wang M, Li Y, Jackson D, Yin A, Dong D, Peng F. Crustal deformation associated with the Altyn Tagh fault system, western China, from GPS. J Geophys Res. 2001;106(30):607–621.
- Steele AD, Emsley JG, Ozdinler PH, Lindquist S, Macklil DJ. Prion protein (PrPc) positively regulates neural precursor proliferation during developmental and adult mammalian neurogenesis. Proc Natl Acad Sci USA. 2006;103:3416–3421.
- Takeuchi N, Ebitani T, Yamamato T, Sato H, Ohta H, Nemoto H, Imbe T, Yano M. Development of isogenics of rice cultivar Koshihikari with early and late heading by marker-assisted selection. Breed Sci. 2006;56:405–413.
- Zhou PH, Tan YF, He YQ, Xu CG, Zhang Q. Simultaneous improvement for four quality traits of Zhenshan 97, an elite parent of hybrid rice, by molecular marker-assisted selection. Theor Appl Genet. 2003a;106:326–331.
- Hospital F. Marker-assisted breeding. In: H.J. Newbury, editor. Plant molecular breeding. Oxford and Boca Raton: Blackwell Publishing and CRC Press; 2003. p. 30–59.
- Bonnett DG, Rebetzke GJ, Spielmeyer W. Strategies for efficient implementation of molecular markers in wheat breeding. Mol Breed. 2005;15:75–85.
- Frisch M, Melchinger AE. Marker-assisted backcrossing for introgression of a recessive gene. Crop Sci. 2001;41:1485–1494.
- Tanksley SD. Molecular markers in plant breeding. Plant Mol Biol Rep. 1983;1:1–3.
- Melchinger AE. Use of molecular markers in breeding for oligogenic disease resistance. Plant Breed. 1990;104:1–19.
- Ribaut JM, Hoisington D. Marker-assisted selection: new tools and strategies. Trends Plant Sci. 1998;3: 236–239.
- Salina E, Dobrovolskaya O, Efremova T, Leonova I, Roder MS. Microsatellite monitoring of recombination around the Vrn-B1 locus of wheat during early backcross breeding. Plant Breed. 2003;122:116–119.
- Ribaut JM, Jiang C, Hoisington D. Simulation experiments on efficiencies of gene introgression by backcrossing. Crop Sci. 2002;42:557–565.
- Babu, R, Nair SK, Kumar A, Venkatesh S, Sekhar JC, Singh NN, Srinivasan G, Gupta HS. Two-generation marker-aided backcrossing for rapid conversion of normal maize lines to quality protein maize (QPM). Theor Appl Genet. 2005;111:888–897.
- Visscher PM, Haley CS, Thompson R. Marker-assisted introgression in backcross breeding programs. Genetics. 1996;144:1923–1932.
- Basavaraj SH, Singh VK, Singh A, Singh D, Nagarajan M, Mohapatra T, Prabhu KV, Singh AK. Marker aided improvement of Pusa6B, the maintainer parent of hybrid Pusa RH10, for resistance to bacterial blight. Indian J Genet Plants Breed. 2009;69:10–16.
- Basavaraj SH, Singh VK, Singh A, Singh A, Singh A, Yadav S, Ellur RK, Singh D, GopalaKrishnan S, Nagarajan M, Mohapatra T, Prabhu KV, Singh AK. Marker-assisted improvement of bacterial blight resistance in parental lines of Pusa RH10, a superfine grain aromatic rice hybrid. Mol Breed. 2010;26:293–305.
- Zhou YL, Uzokwe VNE, Zhang CH, Cheng LR, Wang L, Chen K, Gao X, Sun Q, Chen Y, Zhu JJ, Zhang LH, Ali Q, Xu JL, Li ZK. Improvement of bacterial blight resistance of hybrid rice in China using the Xa23 gene derived from wild rice (Oryza rufipogon). Field Crop Res. 2011;30:637–644.
- Sabouri S, Sabouri A, Jafarzadeh,and MR, Mollashahi M. Detection of QTLs controlling field blast resistance in rice (Oryza sative L.). Plant Omics J. 2011;4(1):1–5.
- Pan HJ, Wang CL, Zhao KJ, Zhang Q, Fan YL, Zhou SC, Zhu LH. Molecular mapping by PCR-based markers and marker-assisted selection of Xa23, a bacterial blight resistance gene in rice. Acta Agron Sin. 2003;29(4):501–507.
- Chen S, Huang ZH, Zeng LX, Yang JY, Liu QG, Zhu XY. High-resolution mapping and gene prediction of Xanthomonas Oryzae pv. Oryzae resistance gene Xa7. Mol Breed. 2008;22:433–441.
- Bentur JS, Srinivasan TE, Kalode MB. Occurrence of a virulent gall midge (GM) Orseolia oryzae Wood mason in Andhra Pradesh, India. Int Rice Res Newsl. 1987;12:33–34.
- Hindu K, Suneetha K, Sama VSAK, Bentur JS. A new rice gall midge resistance gene in the breeding line CR57-MR1523, mapping with flanking markers and development of NILs. Euphytica. 2010;174:179–187.
- Vijayalakshmi P, Amudhan S, Himabindu K, Cheralu C, Bentur JS. A new biotype of the Asian rice gall midge Orseolia oryzae (Diptera: Cecidomyiidae) characterized from the Warangal population in Andhra Pradesh, India. Int J Trop Insect Sci. 2006;26:207–211.
- Roy S, Banerjee A, Tarafdar J, Senapati BK, Dasgupta I. Transfer of transgenes for resistance to rice tungro disease into high-yielding rice cultivars through gene-based marker-assisted selection. J Agric Sci. 2012;150(5)610–618.
- Ahmadi N, Alabar L, Pressori G, Pinel A, Fargette D, Ghesquiere A. Genetic basis and mapping of the resistance to rice yellow mottle virus: III. Analysis of QTL efficiency in introgressed progenies confirmed the hypothesis of complementary epistasis between two resistance QTL. Theor Appl Genet. 2001;103:1084–1092.
- Jairin J, Teangdeerith S, Leelagud P, Kothcharerk J, Sansen K, Yi M, Vanavichit A, Toojinda T. Development of rice introgression lines with brown plant hopper resistance and KDML105 grain quality characteristics through marker-assisted selection. Field Crops Res. 2009;110:263–271.
- Jena KK, Jeung JU, Lee JH, Choi HC, Brar DS. High-resolution mapping of a new brown plant hopper (BPH) resistance gene, Bph18(t), and marker-assisted selection for BPH resistance in rice (Oryza sativa L.). Theor Appl Genet. 2006;112:288–297.
- Septiningsih EM, Pamplona AM, Sanchez DL, Neeraja CN, Vergara GV, Heuer S, Ismail AM, Mackill DJ. Development of submergence-tolerant rice cultivars: the Sub1 locus and beyond. Ann Bot. 2009;103:151–160.
- Siangliwa JL, Jongdeeb B, Pantuwanc G, Toojinda T. Developing KDML105 backcross introgression lines using marker-assisted selection for QTLs associated with drought tolerance in rice. ScienceAsia. 2007;33:207–214.
- Ahn SN, Bollich CN, Tanksley SD. RFLP tagging of a gene for aroma in rice. Theor Appl Genet. 1992;84:825–828.
- Lorieux M, Petrov M, Huang N, Guiderdoni E, Ghesquière A. Aroma in rice: genetic analysis of a quantitative trait. Theor Appl Genet. 1996;93:1145–1151.
- Lang NT, Buu BC. Initial marker-assisted selection in rice breeding at Cuu Long Delta Rice Research Institute. Omonrice. 2010;17:8–21.
- Jin Q, Waters D, Cordeiro G M, Henry R J, Reinke R F. A single nucleotide polymorphism (SNP) marker linked to the fragrance gene in rice (Oryza sativa L.). Plant Sci. 2003;165:359–364.
- Mahdav MS, Pandey MK, Kumar PR, Sundaram RM, Prasad GSV, Sudarshan L, Rani NS. Identification and mapping of tightly linked SSR marker for aroma trait for use in marker assisted selection in rice. Rice Genet Newsl. 2009;25:38–39.
- Yi M, Nwe K T, Vanavichit A, Chai-arree W, Toojinda T. Marker assisted backcross breeding to improve cooking quality traits in Myanmar rice cultivar Manawthukha. Field Crops Res. 2009;113:178–186.
- Singh AK, Gopalakrishnan S, Singh SVP, Prabhu KV, Mohapatra T, Singh NK, Sharma TR, Nagarajan M, Vinod KK, Singh D, Singh UD, Chander S, Atwal SS, Seth R, Singh VK, Ellur RK, Singh A, Anand D, Khanna A, Yadav S, Goel N, Singh A, Shikari A B, Singh A, Marathi B. Marker assisted selection: a paradigm shift in Basmati breeding. Indian J Genet Plant Breed. 2011;71(2):120–128.
- Negrao S, Palaniappan J, Maroco J, Lourenço T, Mackill DJ, Oliveira MM. Bridging sd1 molecular knowledge with recent breeding strategies for the improvement of traditional rice varieties - a japonica case-study. Afr J Biotechnol. 2010;9(15):2192–2200.
- Bohn M, Groh S, Khairallah MM, Hoisington DA, Utz HF, Melchinger AE. Re-evaluation of the prospects of marker-assisted selection for improving insect resistance against Diatraea spp. in tropical maize by cross validation and independent validation. Theor Appl Genet. 2001;103:1059–1067.
- Dreher K, Khairallah M, Ribaut JM, Morris M. Money matters (I): costs of field and laboratory procedures associated with conventional and marker-assisted maize breeding at CIMMYT. Mol Breed. 2003;11:221–234.
- Young ND. A cautiously optimistic vision for marker-assisted breeding. Mol Breed. 1999;5:505–510.
- Yu K, Park S, Poysa V. Marker-assisted selection of common beans for resistance to common bacterial blight: efficacy and economics. Plant Breed. 2000;119:411–415.
- Knapp SJ. Marker-assisted selection as a strategy for increasing the probability of selecting superior genotypes. Crop Sci. 1998;38:1164–1174.
- Charmet G, Robert N, Perretant MR, Gay G, Sourdille P, Groos C, Bernard S, Bernard M. Marker-assisted recurrent selection for cumulating additive and interactive QTLs in recombinant inbred lines. Theor Appl Genet. 1999;99:1143–1148.
- Kuchel H, Ye G, Fox R, Jefferies S. Genetic and economic analysis of a targeted marker-assisted wheat breeding strategy. Mol Breed. 2005;16:67–78.
- Morris M, Dreher K, Ribaut JM, Khairallah M. Money matters (II): cost of maize inbred line conversion schemes at CIMMYT using conventional and marker assisted selection. Mol Breed. 2003;11:235–247.