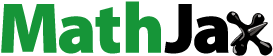
Abstract
The removal of chromium (VI) from aqueous solutions by spent Polysiphonia urceolata and Chondrus ocellatus was investigated with respect to initial pH, amount of biosorbent, initial heavy metal concentrations and temperature. The Cr(VI) removal process was influenced significantly by variations in pH, and the optimum pH was determined to be about 2.0. The optimum algal dosage was 4 g/L and maximum biosorption was achieved at 45 °C in the experiment. The rate of Cr(VI) removal was relatively high in the first 40 min, and biosorption equilibriums were rapidly established in about 60 min for P. urceolata and 40 min for C. ocellatus. The biosorption kinetics followed well a pseudo-second-order model. Adsorption equilibriums were well described by Langmuir and Freundlich isotherm models. The maximum adsorption capacities were 170.6 mg/g for P. urceolata and 113.4 mg/g for C. ocellatus at 298 K and pH 2.0. Fourier transform infrared spectroscopy suggested that amino, hydroxy, C˭O and C–O groups could be involved in the biosorption of Cr(VI) onto P. urceolata and C. ocellatus.
Introduction
Chromium is one of the key contaminants in the wastewaters released by leather tanning, dye, wood preservation and electroplating industries, primarily as trivalent and hexavalent form.[Citation1] Cr(III) is essential to mammalian systems, while Cr(VI) is more toxic than the trivalent form. The adverse effects of Cr(VI) are associated with its potential to modify the DNA transcription process. Cr(VI) is also a carcinogen and is reported to induce epigastric pain, nausea, vomiting, severe diarrhoea and haemorrhage.[Citation2] Thus, it is essential to pursue the development of viable technologies for controlling the concentration of Cr(VI) in industrial wastewaters.
The traditional methods for removing Cr(VI) ions from wastewater include chemical precipitation, chemical oxidation, ion exchange, filtration, electrochemical treatment and evaporative recovery.[Citation3,Citation4] These processes, however, have some serious limitations, e.g. incomplete metal removal, high reagent and energy requirements, and generation of secondary environmental problems due to waste disposal.[Citation5,Citation6] Thus, more efficient and environmentally friendly methods need to be developed for reduction of Cr(VI) content. Biological methods to remove Cr(VI) from aqueous systems afford a promising alternative technology because the process does not produce chemical sludge and is more efficient. In recent years, various kinds of biosorbents, including bacteria, fungi and algae, have attracted the attention of many investigators as organisms to be tested for removal of heavy metals.[Citation7–9] Marine algae are promising biosorbents with high metal-binding capacity due to amino, hydroxyl, carboxyl and sulphate functional groups of polysaccharides, proteins or lipids on the cell wall surface.[Citation10,Citation11] Seaweeds are also available in abundance and at a low cost as a renewable natural biomass.[Citation12] That is why, considerable attempts have been made to use seaweeds for heavy metal removal.
The mechanism involved in the removal of Cr(VI) is complex and depends on the properties of the biosorbents.[Citation13] Previous research shows that the adsorption of Cr(VI) by biomass is mainly through ion exchange and binding on functional groups, e.g. carboxyl, sulphonate, hydroxyl and amino groups.[Citation14]
Polysiphonia urceolata and Chondrus ocellatus are red macroalgae, which grow abundantly in the Yellow Sea from February to April. The aim of this study was to evaluate the capacity of the dried red algae P. urceolata and C. ocellatus to remove Cr(VI) from water and the mechanism of Cr(VI) biosorption.
Materials and methods
Algal biomass preparations
P. urceolata and C. ocellatus were collected at the seaside of Qingdao, China. They were washed three times with distilled water to remove dirt and dried in an oven at 60 °C for 48 h. After that, the algae were fragmented in a triturator and the particles used for the experiments were of an average size of 0.5 mm.
Chromium solution
Stock chromium solution (5 g/L) was prepared by dissolving K2Cr2O7 (GR, Aladdinin Industrial Corporation, China) in deionized distilled water. All working concentrations were obtained by diluting the stock solution with deionized water.
Batch experiments
Each trial was performed by bringing the desired amount of biomass into contact with 100 mL of a Cr(VI) solution of a known concentration in a 250 mL Erlenmeyer flask. The following parameters were chosen as the standard conditions: 1.0 g/L of biomass, 100 mg/L of initial Cr(VI) concentration at pH 2.0 and at temperature 25 °C. To study the effect of biomass concentration on the Cr(VI) reduction rate, biomass concentrations of 0.4, 0.8, 1.2, 1.6, 2.0, 2.4, 2.8, 3.2, 3.6 and 4.0 g/L were employed. To study the effect of the initial Cr(VI) concentration, concentrations of 25, 50, 75, 100, 125, 150, 175, 200, 225 and 250 mg/L were used. To study the effect of pH, the pH value of metal solutions was adjusted to 2.0, 3.0, 4.0, 5.0, 6.0, 7.0 or 8.0. To study the effect of temperature, the flasks were incubated at 25 °C, 35 °C or 45 °C. The flasks were agitated on a shaker at 150 r/min. In the experiments, the solution pH was adjusted at the desired value, using 0.1 mol/L HCl or 0.1 mol/L NaOH solutions.
Equilibrium studies of biosorption
The extent of adsorption on a particular adsorbent depends on the concentration of a metal ion and algal dosage at a constant temperature. The equilibrium is established between adsorbed metal ions on the cell surface and the unadsorbed metal ions in the solution. The amount of Cr(VI) uptake by P. urceolata and C. ocellatus in each flask was determined using the mass balance equation [Citation11]:
(1)
(1) where q is the adsorption amount at equilibrium (mg/g), C0 is the concentration of heavy metal (mg/L), Ce is the concentration remaining in solution at equilibrium (mg/L) and W is the biosorbent dosage (g/L).
Analysis of chromium (VI) ions
The concentration of unadsorbed Cr(VI) ions in the biosorption medium was measured by a UNICO UV 2000 recording spectrophotometer at 540 nm, using diphenylcarbazide as the complexing agent.
Fourier transform infrared analysis (FTIR)
The infrared spectra of both algae were analysed by a Fourier transform infrared spectrometer NICOLET 5700 (Thermo Electron Corporation). For the FTIR study, 30 mg of the biomass was encapsulated in 300 mg of KBr (Sigma) in order to prepare translucent sample disks.
Results and discussion
Effect of pH on Cr(VI) uptake
The initial pH of the metal solution is a key environmental factor affecting the biosorption of metal ions. The initial pH affects not only the cell wall metal-binding sites, but also the metal ion chemistry in water such as hydrolysis, complexation by organic and/or inorganic ligands and redox reactions. Moreover, precipitation, speciation and biosorption availability of the heavy metals are also strongly influenced by pH.[Citation15] Therefore, the effect of initial pH in the range of 1–8 on the efficiency of Cr(VI) removal by dried red algae P. urceolata and C. ocellatus was studied. The results presented in show that, for all the studied concentrations, the percentage of Cr(VI) removal was maximum at pH 2.0 and thereafter decreased with further increase in pH from 2.0 to 8.0. Similar results have been reported for another macroalgal species, Sargassum thunbergii, which could remove 84% Cr(VI) at pH 2.[Citation16] This could be explained by the nature of the biosorption process, the ionic state of the functional group of the sorbent and the metal chemistry at different pH values.[Citation17]
Figure 1. Cr(VI) removal efficiencies by Polysiphonia urceolata and Chondrus ocellatus at different pH.
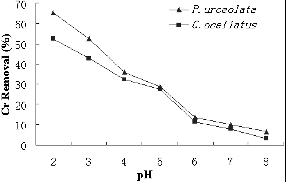
Biosorption of Cr(VI) varies as a function of pH, with chromic acid (H2CrO4) and its salts, hydrogen chromate (HCrO4−) and chromate (CrO42−) ions present as dominant species.[Citation18] At pH < 3, HCrO4− is the dominant species.[Citation5] The surface charge of P. urceolata and C. ocellatus should be positive and this should promote binding of the negatively charged chromium species. In the pH range 3–5, the decrease in adsorption with the increasing pH could most likely be due to the relatively weak coordination bonds between metal ions and phenolic hydroxyl functional groups and other mechanisms such as physical adsorption on the sorbent surface.[Citation19,Citation20] At higher pH (pH > 5), there was further decrease in adsorption, which may be due to the formation of soluble hydroxylated complexes of the metal ions and/or to the ionized nature of the cell wall surface of the adsorbent.[Citation21]
Effect of temperature and adsorbent dose on Cr(VI) adsorption
The biosorption of Cr(VI) at different temperatures for both algal species is shown in . There was enhanced biosorption at higher temperatures, indicating the endothermic nature of Cr(VI) biosorption using P. urceolata and C. ocellatus biomass. These higher removal efficiencies observed at higher temperatures might be due to a higher affinity of metal-binding sites or an increase in binding sites.[Citation22]
Figure 2. Cr(VI) removal efficiencies by Polysiphonia urceolata (a) and Chondrus ocellatus (b) at different temperature. C0 – initial Cr(VI) concentration (mg/L).
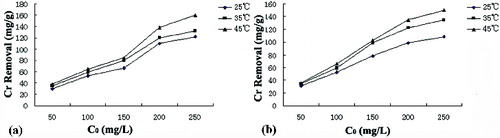
Biosorption is highly dependent on the initial adsorbent concentration because the extent of biosorption is proportional to the special binding area, which can be defined as the portion of the total area that is available for biosorption. The effects of algal doses on the removal of Cr(VI) from aqueous solutions were investigated using different adsorbent concentrations from 0.4 to 4.0 g/L. As shown in , the percentage of removal of Cr(VI) increased with increasing doses (biomass) of both adsorbents due to greater surface area and pore volume.[Citation23] At very low adsorbent concentrations, the biosorbent surface most probably becomes saturated with Cr(VI), and a large amount of Cr(VI) that remains in the solution.
Kinetic studies of Cr(VI) removal
The uptake of heavy metal ions by seaweeds often occurs in two stages: an initial rapid uptake due to surface adsorption on the cell walls and a subsequent uptake due to membrane transport of the metal ions on the cytoplasm of the cells.[Citation24] shows the Cr(VI) removal (in percentage) by P. urceolata and C. ocellatus at 100 mg/L initial Cr concentration. The removal rate was fast in the first 40 min, and biosorption equilibriums were rapidly established in about 60 min for P. urceolata and 40 min for C. ocellatus.
The pseudo-second-order kinetic model based on the sorption capacity of the solid phase was used in this case, with the assumption that the measured concentrations are equal to the cell-surface concentrations.[Citation10] The pseudo-second-order kinetic rate equation is expressed as follows:
(2)
(2) To obtain a linearized form of the pseudo-second-order model, EquationEquation (2)
(2)
(2) can be integrated for the boundary conditions qt = 0, at t = 0, and qt at time t:
(3)
(3) where k2 is the second-order biosorption rate constant, qe and qt are the amounts of sorbed metal ions on the biosorbent at equilibrium and at any time t, respectively.
shows the plots of t/qt vs. t at 100 mg/L Cr. The values of the parameters k2, qe and R2 are presented in . The coefficient of determination (R2) obtained was 0.995. The linear relationship between t/qt and t suggests that the sorption of Cr(VI) onto P. urceolata and C. ocellatus fitted the second-order model well, and this stage may depend on the diffusion rate of the ions to the cell surface. Therefore, the topography and the surface properties of the biosorbent may be the major influencing factors.[Citation25] The longer contact time needed for a state of equilibrium to be reached with P. urceolata than that with C. ocellatus suggests a possible difference in adsorption kinetic models.
Table 1. Pseudo-second-order adsorption constants for Polysiphonia urceolata and Chondrus ocellatus.
Effect of initial Cr(VI) concentration on biosorption
The initial metal ion concentration is generally considered a key driving force to overcome the mass transfer resistance of metal ions between the aqueous and the solid phase. The effect of different initial concentrations of Cr(VI) on the biosorption of Cr(VI), using the red algae P. urceolata and C. ocellatus, is demonstrated by the results in . When the initial concentration of Cr(VI) was increased from 25 to 250 mg/L, the loading capacity was increased from 16.1 to 128.2 mg/L for P. urceolata and from 17.3 to 105.2 mg/L for C. ocellatus.
Figure 6. The effect of initial metal concentrations on the removal percentage of Cr(VI). C0 – initial Cr(VI) concentration (mg/L).
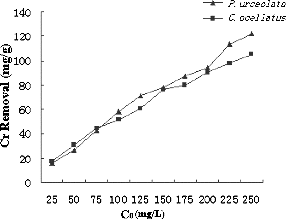
Adsorption isotherms are used to express the equilibrium relationship between the concentration of metal ions adsorbed per unit mass of original biosorbent (qeq; [mg/g]) and the concentration of adsorbed metal ions at a constant temperature (Ceq). Out of many adsorption isotherms available, the Freundlich and Langmuir adsorption isotherms were used to fit the data in this study. The Freundlich adsorption isotherm is given as
(4)
(4) where KF and 1/n are Freundlich adsorption constants. The Langmuir isotherm is derived with the assumption that the surface is uniform with finite identical sites and that there is monolayer adsorption of the adsorbate.[Citation26] The Langmuir adsorption isotherm is given by the following relation:
(5)
(5) where, qmax and b are maximum adsorbate loading (mg/L) and Langmuir adsorption constant (mg/L), respectively.
The results shown in indicate a non-linear relationship between the amount (mg) of Cr(VI) sorbed per unit mass (g) of P. urceolata or C. ocellatus and the concentration of Cr(VI) remaining in solution (mg/L). The adsorption equilibria of Cr(VI) by P. urceolata and C. ocellatus at different initial Cr concentration were well described by the Langmuir and Freundlich isotherm models. The equilibrium constants and coefficient of determination (R2) are presented in . The 1/n values for both macroalgal species were between 0 and 1, indicating that the biosorption of Cr(VI) using P. urceolata and C. ocellatus biomass was favourable at the studies conditions. The maximum adsorption capacity of P. urceolata and C. ocellatus obtained from the Langmuir isotherm was 170.6 and 113.4 mg/g, respectively. The value of b indicates the affinity of the sorbate for the solute.[Citation27] The b values were 0.0081 and 0.023 L/mg for P. urceolata and C. ocellatus, respectively, suggesting that P. urceolata biomass is more effective at adsorbing Cr(VI).
Figure 7. Adsorption isotherms of Cr(VI) by Polysiphonia urceolata and Chondrus ocellatus. Ce – the Cr(VI) concentration remaining in solution at equilibrium (mg/L).
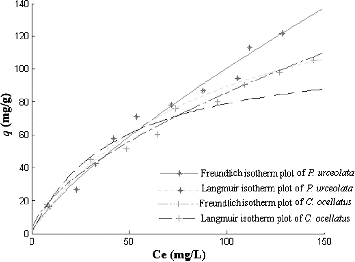
Table 2. Langmuir and Freundlich sorption constants of Polysiphonia urceolata and Chondrus ocellatus.
Comparative analysis
The comparative analysis of our results with those of other studies shows that the value of pH 2.0, which was found optimal for Cr(VI) removal in our experiments, is in agreement with reports for Polysiphonia lanosa and Bengal gram husk.[Citation28,Citation30] However, there may be different optimal pH values for Cr(VI) removal by different adsorbents. For example, pH 1.0 is optimal for Ulva lactuca.[Citation12]
The Langmuir adsorbing capacity (qmax) can be used to compare the efficiency of P. urceolata and C. ocellatus with that of other materials which have been tested as biosorbents for Cr(VI). As shown in , P. urceolata has a greater capacity than some of those biosorbents, which, together with the large amount of P. urceolata biomass available in the Yellow Sea, suggests that P. urceolata biomass could be considered as a promising and cost effective adsorbent for Cr(VI) removal at least on a local scale.
Table 3. Comparison of the biosorption capacity of Polysiphonia urceolata and Chondrus ocellatus for Cr(VI) with that of various biosorbents.
Fourier transform infrared spectroscopy (FTIR)
As a next step in our study, the FTIR spectra of unloaded biomass and of Cr(VI)-loaded biomass were taken to obtain information on the possible nature of the interactions between the functional groups of P. urceolata biomass and the metal ions (). The broad and strong band at 3392.7 cm−1 (a)) may be due to the overlapping of O–H and N–H stretching vibration, showing the presence of hydroxyl and amine groups on the adsorbent. The band at 2921 cm−1 is assigned to the –CH stretch. The absorption peaks at 1654 and 1540 cm−1 corresponded to the amide I and amide II bands, respectively. The bands at 1249.7 and 1068.2 cm−1 represent P–O and C–O bands of polysaccharides, respectively. After Cr(VI) biosorption, the band at 3392.7 cm−1 was shifted to 3407 cm−1, and the bands observed at 1654.2, 1504.7 and 1449.3 cm−1 were shifted to 1653.2, 1541.4 and 1451.8 cm−1 (b)).
The FTIR spectra of unloaded C. ocellatus biomass (a)) and hexavalent chromium-loaded biomass (b)) showed that after chromium exposure, the broad and strong band at 3372.7 cm−1 was shifted to 3422 cm−1, and the bands observed at 1653.2 and 1407.2 cm−1 were shifted to 1637.9 and 1544 cm−1. The band at 1071.9 cm−1 that corresponds to stretching of C–O groups was shifted to 1080.8 cm−1 after loading with Cr(VI). The changes in the wave numbers of these specific peaks suggests that amino, hydroxy, C˭O and C–O groups could be involved in the biosorption of Cr(VI) onto P. urceolata and C. ocellatus.
The spectrometer analysis (FTIR) confirmed that the main adsorption sites for Cr(VI) on the biomass of P. urceolata and C. ocellatus are amino groups. Similar results have been reported in Chlorella miniata and some aquatic weeds.[Citation31,Citation32] Other adsorption sites for Cr(VI) biosorption are hydroxy, C˭O and C–O groups. Therefore, depending on the pH value of the aqueous solution, several functional groups can bind Cr(VI) ions on P. urceolata and C. ocellatus surface.
Conclusions
This study on the properties of two marine red algae (P. urceolata and C. ocellatus) abundantly present in the Yellow Sea as potential biosorbents for Cr(VI) removal focused on the operating parameters, pH of solution, biomass dosage, temperature and time, as factors affecting the biosorption capacity. The adsorption kinetics fitted a pseudo-second-order model. The equilibrium data followed the Langmuir and Freundlich isotherm models well and the maximum adsorption capacities were 170.6 mg/g for P. urceolata and 113.4 mg/g for C. ocellatus. Taken together, the obtained results suggest that P. urceolata biomass has the potential to be used as an efficient and economic biosorbent for the removal and recovery of toxic Cr(VI) from wastewater due to its high Cr(VI) biosorption capacity and low cost.
Disclosure statement
No potential conflict of interest was reported by the authors.
Additional information
Funding
References
- Barnhart J. Occurrences, uses and properties of chromium. Regul Toxicol Pharmacol. 1997;26(1):S3–S7. Available from: http://dx.doi.org/10.1006/rtph.1997.1132.
- Dakiky M, Khamis M, Manassra A, Mer'eb M. Selective adsorption of chromium(VI) in industrial wastewater using low-cost abundantly available adsorbents. Adv Env Res. 2002;6:533–540. Available from: http://dx.doi.org/10.1016/S1093-0191(01)00079-X.
- Elangovan R, Philip L, Chandraraj K. Biosorption of chromium species by aquatic weeds: kinetics and mechanism studies. J Hazard Mater. 2008;152:100–112. Available from: http://dx.doi.org/10.1016/j.jhazmat.2007.06.067.
- Kurniawan TA, Chan GYS, Lo W-H, Babel S. Physico-chemical treatment techniques for wastewater laden with heavy metals. Chem Eng J. 2006;118:83–98. Available from: http://dx.doi.org/10.1016/j.cej.2006.01.015.
- Arslan G, Pehlivan E. Batch removal of chromium(VI) from aqueous solution by Turkish brown coals. Bioresour Technol. 2007;98:2836–2845. Available from: http://dx.doi.org/10.1016/j.biortech.2006.09.041.
- Volesky B, Holan ZR. Biosorption of heavy metals. Biotechnol Prog. 1995;11:235–250. Available from: http://dx.doi.org/10.1021/bp00033a001.
- Dursun AY, Uslu G, Cuci Y, Aksu Z. Bioaccumulation of copper(II), lead(II) and chromium(VI) by growing Aspergillus niger. Process Biochem. 2003;38:1647–1651. Available from: http://dx.doi.org/10.1016/S0032-9592(02)00075-4.
- Jácome-Pilco CR, Cristiani-Urbina E, Flores-Cotera LB, Velasco-Garcí R, Ponce-Noyola T, Caňzares-Villanueva RO. Continuous Cr(VI) removal by Scenedesmus incrassatulus in an airlift photobioreactor. Bioresour Technol. 2009;100:2388–2391. Available from: http://dx.doi.org/10.1016/j.biortech.2008.10.053.
- Quintelas C, Rocha Z, Silva B, Fonseca B, Figueiredo H, Tavares T. Removal of Cd(II), Cr(VI), Fe(III) and Ni(II) from aqueous solutions by an E. coli biofilm supported on kaolin. Chem Eng J. 2009;149:319–324. Available from: http://dx.doi.org/10.1016/j.cej.2008.11.025
- Deng LP, Zhu XB, Wang XT, Su YY, Su H. Biosorption of copper(II) from aqueous solutions by green alga Cladophora fascicularis. Biodegradation. 2007;18:393–402. Available from: http://dx.doi.org/10.1007/s10532-006-9074-6.
- Deng LP, Su YY, Su H, Wang XT, Zhu XB. Sorption and desorption of lead (II) from wastewater by green algae Cladophora fascicularis. J Hazard Mater. 2007;143(1–2):220–225. Available from: http://dx.doi.org/10.1016/j.jhazmat.2006.09.009.
- El-Sikaily A, Nemr AE, Khaled A, Abdelweha O. Removal of toxic chromium from wastewater using green alga Ulva lactuca and its activated carbon. J Hazard Mater. 2007;148:216–228. Available from: http://dx.doi.org/10.1016/j.jhazmat.2007.01.146.
- Han X, Wong YS, Wong MH, Tam NFY. Biosorption and bioreduction of Cr(VI) by a microalgal isolate, Chlorella miniata. J Hazard Mater. 2007;146:65–72. Available from: http://dx.doi.org/10.1016/j.jhazmat.2006.11.053.
- Donmez G, Aksu Z. Removal of chromium(VI) from saline wastewaters by Dunaliella species. Process Biochem. 2002;38:751–762. Available from: http://dx.doi.org/10.1016/S0032-9592(02)00204-2.
- Esposito A, Pagnanelli F, Veglio F. pH-related equilibria models for biosorption in single metal systems. Chem Eng Sci. 2002;57(3):307–313. Available from: http://dx.doi.org/10.1016/S0009-2509(01)00399-2.
- Wang Y, Li YX, Zhao FJ. Bisorption of chromium(VI) from aqueous solutions by Sargassum thunbergii Kuntze. Biotechnol Biotechnological Equipment. 2014;28(2):259–265, Available from: http://dx.doi.org/10.1080/13102818.2014.907028.
- Matheickal JT, Yu Q, Woodburn GM. Biosorption of cadmium from aqueous solutions by pre-treated biomass of marine alga Durvillaea potatorum. Water Res. 1999;33:335–342. Available from: http://dx.doi.org/10.1016/S0043-1354(98)00237-1.
- Kota J, Stasicka Z. Chromium occurrence in the environment and methods of its speciation. Environ Pollut. 2000;107(3):263–283. Available from: http://dx.doi.org/10.1016/S0269-7491(99)00168-2.
- Gupta VK, Shrivastava AK, Jain N. Biosorption of chromium(VI) from aqueous solutions by green algae Spirogyra species. Water Res. 2001;35:4079–4085. Available from: http://dx.doi.org/10.1016/S0043-1354(01)00138-5.
- Argun ME, Dursun S, Ozdemir C, Karatas M. Heavy metal adsorption by modified oak sawdust: thermodynamics and kinetics. J Hazard Mater. 2007;141:77–85. Available from: http://dx.doi.org/10.1016/j.jhazmat.2006.06.095.
- Sarı A, Tuzen M. Biosorption of total chromium from aqueous solution by red algae (Ceramium virgatum): equilibrium, kinetic and thermodynamic studies. J Hazard Mater. 2008;160:349–355. Available from: http://dx.doi.org/10.1016/j.jhazmat.2008.03.005.
- Zhou M, Liu Y, Zeng G, Li X, Xu W, Fan T. Kinetic and equilibrium studies of Cr (VI) biosorption by dead Bacillus licheniformis biomass. World J Microbiol Biotechnol. 2007;23:43–48. Available from: http://dx.doi.org/10.1007/s11274-006-9191-8.
- Malkoc E, Nuhoglu Y. The removal of chromium (VI) from synthetic wastewater by Ulothrix zonata. Fresenius Environ Bull. 2003;12:376–381.
- Davis TA, Volesky B, Vieira RHSF. Sargassum seaweed as biosorbent for heavy metals. Water Res. 2000;34(17):4270–4278. Available from: http://dx.doi.org/10.1016/S0043-1354(00)00177-9.
- Shen L, Xia J-I, He H, Nie Z-y. Comparative study on biosorption of Pb(II) and Cr(VI) by Synechococcus sp. Trans Nonferrous Met Soc China. 2008;18:1336–1342.
- Langmuir I. The adsorption of gases on plane surfaces of glass, mica and platinum. J Am Chem Soc. 1918;40(9):1361–1403.
- Holan ZR, Volesky B, Prasetyo I. Biosorption of cadmium by biomass of marine algae. Biotechnol Bioeng. 1993;41:819–825. Available from: http://dx.doi.org/10.1002/bit.260420422.
- Ahalya N, Kanamadi RD, Ramachandra TV. Biosorption of chromium (VI) from aqueous solutions by the husk of Bengal gram (Cicer arientinum). Electron J Biotechnol. 2005;8(3):258–264. Available from: http://dx.doi.org/10.2225/vol8-issue3-fulltext-10.
- Gokhale SV, Jyoti KK, Lele SS. Kinetic and equilibrium modeling of chromium (VI) biosorption on fresh and spent Spirulina platensis/Chlorella vulgaris biomass. Bioresour Technol. 2008;99:3600–3608. Available from: http://dx.doi.org/10.1016/j.biortech.2007.07.039.
- Murphy V, Hughes H, McLoughlin P. Comparative study of chromium biosorption by red, green and brown seaweed biomass. Chemosphere. 2008;70:1128–1134. Available from: http://dx.doi.org/10.1016/j.chemosphere.2007.08.015.
- Han X, Wong YS, Wong MH, Tam NFY. Biosorption and bioreduction of Cr(VI) by a microalgal isolate, Chlorella miniata. J Hazard Mater. 2007;146:65–72. Available from: http://dx.doi.org/10.1016/j.jhazmat.2006.11.053.
- Elangovan R, Philip L, Chandraraj K. Biosorption of chromium species by aquatic weeds: kinetics and mechanism studies. J Hazard Mater. 2008;152:100–112. Available from: http://dx.doi.org/10.1016/j.jhazmat.2007.06.067.