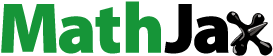
Abstract
The aim of this study was to determine the optimal conditions for adding eggshells as a bioflocculant (including concentration dosage, pH, mixing time, mixing rate and settling time) in harvesting Chlorella vulgaris cells from liquid cultures, using the well-known jar-test experimental method. Eggshells separated successfully 99 ± 0.4% of the C. vulgaris biomass at the following optimal parameters: eggshell concentration 80 mg/L, mixing time 20 min, mixing rate 150 r/min and settling time 20 min at pH 6. These results indicated that eggshells, as a biopolymer, are a promising flocculant due to their high separation efficiency, low dose requirements and short mixing and settling time. Eggshells not only act as an adsorbent, but also spontaneously coagulate to agglomerate the microalgae cells.
Introduction
Biomass energy, as a green and renewable resource, is considered as one of the most promising ways to solve the global energy crisis.[Citation1,Citation2] Microalgae have been found to be an economical and potential raw material for biomass energy,[Citation3] since they do not require large areas of land for cultivation, have a short growth period and a high growth rate and contain more high-lipid materials than the usual food crops.[Citation2,Citation4] However, as pointed out by Malm and Tanner [Citation5], harvesting microalgae biomass is challenging mainly due to the small size of microalgae, their tendency to grow as single cells and the low cell density in the culture medium.[Citation6,Citation7] The solution volumes to be treated are large and the amount of processing and energy needed renders the separation of pure microalgae biomass demanding and expensive. It has been calculated that up to 20%–30% of the total production costs are due to the biomass recovery stage.[Citation8] Therefore, an efficient/cost effective technique for harvesting of microalgae is required to be developed. This could significantly reduce the input cost of biodiesel production. Briefly, there are several relatively efficient ways for microalgae harvesting, viz., centrifugation, sonication, filtration, air floatation, coagulation, flocculation, etc. Among these, flocculation is often considered a harvesting technique of choice, as it is relatively cheap. Inorganic salts, such as ferric chloride and aluminium sulphate, could serve as ligands to cause microalgal cells to flocculate and settle down efficiently.[Citation9–12] These chemicals are known as efficient flocculants, but despite their effectiveness, have limited potential of implementation.[Citation13] This is due to their high cost, which makes the process economically unviable. Moreover, the addition of chemical flocculants makes the overall downstream process complicated. Reuse of spent medium, which is absolutely necessary to reduce fuel production cost, becomes difficult and cumbersome.[Citation9,Citation14]
The use of eggshells as a coagulating natural material is greatly recommended since eggshells are non-toxic, non-corrosive and easy/safe to handle. They are biodegradable, biocompatible and show promising adsorption properties and flocculation ability. Moreover, they can be regenerated and reused several times.[Citation15] Eggshells have a high cationic charge density and can, thus, strongly adsorb and destabilize negative particles, such as the negatively charged microalgae cells. The main ingredient in eggshells (and sea shells) is calcium carbonate. It constitutes about 95% of eggshells and the remaining 5% include calcium phosphate, magnesium carbonate, and soluble and insoluble proteins.[Citation16]
There are many different methods for harvesting of microalgae. However, to the best of our knowledge, there are no reports on the effect of eggshells on the precipitation of microalgae. Therefore, the aim of this study was to investigate the role of eggshells in the flocculation of living microalgae from aqueous solutions. The effects of several factors, viz., pH, dosage, mixing time, mixing rate and settling time, in the settling of microalgae were further investigated.
Materials and methods
Microalgae cultures and medium
Chlorella vulgaris (C. vulgaris: KMMCC 145) was obtained from KMMCC (Korea Marine Microalgae Culture Center) and was cultivated in Jaworski's medium (JM) under LED (light-emitting diode) lamps at ambient temperature. JM contains 4.0 g Ca(NO3)2·H2O, 2.48 g KH2PO4, 10.0 g MgSO4·7H2O, 3.18 g NaHCO3, 0.45 g ethylenediaminetetraacetic acid (EDTA) ferric sodium salt (EDTA-FeNa), 0.45 g EDTA-Na2, 0.496 g H3BO3, 0.278 g MnCl2·4H2O, 0.20 g (NH4)6Mo7O24·4H2O, 0.008 g cyanocobalamin, 0.008 g thiamine HCl, 0.008 g biotin, 16.0 g NaNO3 and 7.2 g Na2HPO4·12H2O in 200 mL deionized water. The microalgae were cultured in 200 mL conical flasks containing 100 mL of JM with 10 mL C. vulgaris inoculum at pH 7.2 ± 0.3. The cultures were maintained in a dark and light cycle of 8 and 16 h, respectively, for 10 days. The temperature was maintained at 23 ± 1 °C using LEDs. The LED light source was used because it is efficient and provides the required wavelength light from 430 to 670 nm, which is selective for microalgal growth. Moreover, the light intensity was 200–250 µmol photons/(m2·s). An equivalent CO2 aeration rate of 0.02 vvm was used for cultivation. The initial concentration of the inoculated microalgae was 0.357 ± 0.7 g/L.
Preparation of eggshells solution
The collected eggshells were rinsed with distilled water and dried at 35 °C in a thermostat. Dried eggshells were ground to obtain the fine powder and sieved mechanically using a 325 mesh size sieve. The eggshell powder (100 mg) was dissolved in 10 mL of 0.1 mol/L acid solution with continuous stirring for 30 min. The acid solution was then diluted to 100 mL using deionized water to a final eggshell concentration of 1000 mg/L.
Analytical method
To determine the biomass concentration, a sample of microalgae in growth medium was centrifuged for 10 min at 628g, washed with distilled water and then dried in an oven at 105 °C for 24 h to obtain constant weight. The biomass productivity P (g/(L·day)) was calculated from the variation in biomass concentration (g/L) within a cultivation time (in days), according to the following equation:(1)
(1)
The specific growth rate µ (in days) was calculated using the following equation:(2)
(2) where X1 and X0 are the biomass concentration (g/L) on days t1 and t0, respectively.
To determine the separation efficiency, biomass was recovered at different flocculants dosage, i.e. 0–120 mg/L with an increment of 20 mg/L, and at different pH values, i.e., 2–10 with an increment of 1. pH values were maintained using NaOH 0.5 mol/L and HCl 0.5 mol/L. Ten millilitres of microalgal cells were placed in a 15 mL falcon tube. Eggshell solution was added at a designated concentration and mixed at 0–300 r/min, using a mini orbital shaker (VWR® Advanced Orbital Shaker, Model 15000). After mixing, the algal cells were allowed to settle down. The supernatant was taken over time from the middle of the algae suspension to measure its optical density (OD). The OD was measured at 600 nm (OD600) by a spectrophotometer (Beckman Coulter, model DU 730) equipped with a carousel cell holder. The OD 600 values were calibrated against dry cell weight. The separation efficiency (%) was calculated as follows:(3)
(3) where
is the OD of samples taken at time zero (t0) and ODt is the OD at time t.
The zeta potential of the different eggshell doses was determined with a Malvern ZetaSizer Nano ZS90 (Malvern Instruments Ltd., UK). One millilitre of suspension was pipetted into a cuvette and inserted into the unit for zeta potential without pH and osmotic adjustment.[Citation17] Zeta potential was measured in triplicate at room temperature and the average values were then determined. illustrates the procedure of microalgae harvesting.
Data analysis
Data presented in tables and figures are mean values ±3σ from five replications. Where error bars are not visible, errors were smaller than or equal to the symbols. The differences between mean values were calculated using Tukey's test at the 0.05 level by Origin software (v.7.5, OriginLab, Northampton, MA, USA).
Results and discussion
Food industry generates thousands of tons of eggshell waste annually. In Korea, egg production reached 0.72 m tons (about 12.1 billion eggs) in 2014. It would be advantageous to find a method of cost-effective utilization of this waste. For this purpose we explored the possibility of using eggshells for flocculation of microalgae, which might possibly find application in biofuel production.
Growth of microalgae
presents graphically the growth of C. vulgaris in JM medium. During the first five days, the microalgae growth was rapid; thereafter it attained almost a constant level. The highest biomass concentration and maximum specific growth rate of C. vulgaris achieved was 2.25 g/L and 0.453 1/day, respectively. Soletto et al. [Citation18] reported 2.8 g/L maximum cell concentrations on the fed-batch pulse-feeding cultivation of Spirulina platensis in a helical photobioreactor. Mezzomo et al. [Citation19] reported a maximum specific growth rate of 0.4 1/day by cultivation of S.platensis from biological treatment of swine wastewater. However, this was significantly higher compared to the reported specific growth rate of 0.6 1/day (doubling time 11.4 days) using Chlorella sp. in a photobioreactor with transparent rectangular chambers.[Citation20] In this present study, the biomass productivity and maximum specific growth rate were obtained in the normal range compared to other studies.
Effect of pH
The efficiency of separation of C. vulgaris cells at different pH values was studied (). In the presence of eggshells, the highest harvesting efficiency of 99 ± 0.3% (at 80 mg/L eggshells) was obtained. The results further showed that pH 6.0–7.0 was optimal. However, with further increase in pH, i.e., beyond pH 6.0, a slight decrease in the separation efficiency was observed, reaching to 88 ± 1.3% at a pH 10. The pH effect could be explained with the physical properties of eggshells and physicochemical interactions between eggshells and microalgae cells.[Citation16] In fact, a change in pH is known to affect the flocculant structure. At neutral pH, flocculants possess a coil-like structure.[Citation2] The surface of C. vulgaris cells is likely to be protonated at very low pH values and to become deprotonated with increasing the pH. Variations in the surface charge affect the interaction between particles, and in turn, the flocculation efficiency as well. Thus, as a result of acid dissociation, at higher pH values, i.e., pH beyond 6.0, the cell surface may become negatively charged. On the other hand, eggshells are also likely to become protonated at acidic conditions, which can greatly hinder the flocculation and decrease the settling of microalgae. However, at pH 6.0, the net negative charge carried by the microalgae cell surface most likely favours the electrostatic attraction between the eggshells and microalgae. This could apparently be considered to enable in enhanced settling of the microalgae. Moreover, the slight decrease observed in the settling within the pH region of 6.0–10.0 could be possibly due to the fact that at high pH conditions the eggshells also become negatively charged; hence, the partial decrease in the settling of negatively charged microalgae. Previously, it was reported that repulsive forces decrease the interaction between the particles, and thus, flocculation is impaired.[Citation21] These findings are in agreement with the results reported by Xu et al. [Citation13] Likewise, at high pH (>8), the particles are highly negatively/positively charged, which also causes repulsion, resulting in decreased separation efficiency. Other studies report that divalent metal ions, such as Mg2+, Ca2+, etc., play an important role in flocculating microalgae by pH variation.[Citation15,Citation21–24] Such metal ions in the growth medium are hydrolyzed to form positive precipitates which coagulate negatively charged microalgal cells by sweeping flocculation and charge neutralization.[Citation2]
In dense microalgal cultures, pH is often highly variable: it may increase to 10 due to intensive primary production or decreased to 6 during CO2 addition or as a result of respiration.[Citation10] The pH values affect the zeta potential of charged particles, and interfere with the flocculation capacity.[Citation10,Citation14] The increase in flocculation efficiency at high pH observed by some authors is probably due to some autoflocculation of microalgae, which occurs at pH 10 or even higher.[Citation8,Citation9] As an alternative to the use of eggshells; an increase in the pH by intense microalgae photosynthesis may be considered. In cultures of C. vulgaris, Vandamme et al. [Citation21] observed that flocculation occurred when CO2 supply was interrupted and pH was increased to 9.5 as a result of photosynthesis. Putt et al. [Citation25] also noted that flocculation of microalgae in high rate algal ponds occurred during warm and sunny days, when low solubility of CO2 and high photosynthetic uptake of CO2 resulted in high pH conditions. However, a disadvantage of this method is that the combination of high pH and warm conditions may lead to significant destruction of microalgae and, thus, to production of less biomass.
Effect of eggshell dose
The results from the experiments on the effect of different eggshell doses (0–120 mg/L) on the efficiency of harvesting of C. vulgaris showed that the separation efficiency was increased with increasing the eggshell dose. The maximum separation efficiency (99 ± 0.4%) was observed with an eggshell dose of >80 mg/L, whereas the lowest efficiency (50 ± 2%), with a dose of 20 mg/L (). Thus, the maximum separation efficiency achieved using our experimental design exceeds the 60% reported by Papazi et al. [Citation24] in Chlorella minutissima, using a comparatively high dose of chemical coagulants, i.e., 1000 mg/L of Al2(SO4)3, and incubation time of 6 h. A 99% harvesting efficiency of C. vulgaris similar to that achieved in our experiments was also observed by Rashid et al. [Citation8] at pH 6, but using a higher dose (120 mg/L) of chitosan than the 80 mg/L of eggshells applied by us.
Since the microalgae culture and eggshells could be considered as a colloidal dispersion, the zeta potential was determined as a key indicator of the dispersion stability, i.e., of the degree of electrostatic repulsion between adjacent dispersed particles with a similar charge. Generally, high (negative or positive) zeta potentials are indicative of electrically stabilized colloids, whereas low zeta potentials are indicative of coagulation or flocculation trends.[Citation8,Citation9] shows the changes in the zeta-potential with eggshell dose. The zeta-potential of microalgae culture generally increased with flocculants dose: from −22.3 ± 2.4 mV in the C. vulgaris culture without eggshells to +1.12 ± 0.4 mV at 120 mg/L of eggshells. Such an increase in the zeta-potential suggests a decrease in the surface charge of microalgae cells as a result of neutralization by eggshells.[Citation9]
Salim et al. [Citation26] demonstrated that during microalgae growth, positively charged polymers become accumulated on the negatively charged outer cell surface. However, inactive cells or dead cells do not show ionic transport, and thus, the surface charge is determined by the surface equilibrium charge. Rashid et al. [Citation8] also reported an increasing trend of zeta-potential with increase in flocculants dose. Zeta potential values of ±15 mV usually represent the onset of agglomeration.[Citation27] There is a threshold region of either coagulation or dispersion from about ±14 to ±30 mV.[Citation28] Generally, zeta-potential values from 0 to ±5 represent rapid coagulation or flocculation, from ±10 to ±30 incipient instability and values more electronegative than ±30 mV generally represent sufficient mutual repulsion to result in stability (i.e. no agglomeration).[Citation9,Citation27] In the present study, an eggshell dose of over 60 mg/L corresponded to a zeta-potential in the range of 0 to ±5. This eggshell dose was considered favourable to achieve over 95% separation efficiency.
Effect of mixing rate
The mixing speed is a critical factor in the flocculation process from the perspective of both separation efficiency and energy saving. represents the separation efficiency at different mixing rates. The results indicated that the mixing rate indeed had a significant impact on the separation efficiency. A mixing speed of 150 r/min resulted in the coagulation 99.4 ± 0.5% of microalgae cells. That is, this rate was sufficient for nearly all microalgae cells to be agglomerated with the eggshells. However, when the mixing rate was increased further, the removal percentage was decreased slightly, reaching 95.2 ± 1.3% at a rate of 300 r/min. This was most probably due to re-stabilization of the cells at fast mixing speeds, as high-speed mixing tends to break the flocs, causing the coagulated cells to re-disperse again into the medium.
Table 1. Separation efficiency at different mixing rate and mixing time.
It has been recommended for rapid mixing to be followed by slow mixing during the coagulation process, as the speed of mixing may affect the coagulation before the optimal dosage is reached. Then, after reaching optimal dosage, no significant difference appears to be observed in the size of flocs with the settling velocity.[Citation26,Citation29] Another factor that affects the destabilization of the colloid and the downstream aggregation of the particles during coagulation is the time of rapid mixing.[Citation26] Actually, high mixing rate allowed the negatively charged algal cells and protonated eggshell particles to mix evenly and rapidly throughout the suspension. In contrast, low mixing rate is likely to cause non-uniform distribution of flocculants. The flocs formed are relatively weak and become destabilized after some time, decreasing the separation efficiency.[Citation10,Citation13,Citation29] The mixing rate also had a significant impact on the charge neutralization and sweep coagulation due to the restabilization of the cells when the mixing speed is very fast.[Citation29]
Effect of mixing time
The relationship between mixing time and the separation efficiency of C. vulgaris cells showed that at least 20 min of mixing time was needed for 99.1 ± 0.5% of C. vulgaris cells to coagulate in the presence of eggshells (). The shorter the mixing times were, the less the percentage of C. vulgaris cells separation was. Only 86.2 ± 1.2% of C. vulgaris cells were coagulated after 2 min of operation. The lower separation efficiency was most probably caused by the decrease in contact between the cells and the flocculant.[Citation7,Citation29] As the mixing time was increased from 5 to 20 min, the total number of collisions was increased significantly. Similar to the results obtained with other flocculants,[Citation9,Citation29] this could be due to higher probability of cells and eggshell particles to collide with each other, allowing coagulation and adsorption to occur spontaneously. For example, the adsorption kinetics of emulsifiers at oil–water interface show that longer mixing times result in an increase in the interfacial area because of increased number of oil droplet breakage.[Citation3,Citation29] This suggests that, similar to the study of Ahmad et al. [Citation29] with chitosan as a flocculant, the increased time of mixing of eggshells and microalgae cells in our experiments may cause increased adsorption.
In this study, with mixing times greater than 20 min, the separation efficiency stayed at the maximal value. The eggshell-aggregated flocs remained intact even after the highest duration (60 min) of mixing. Thus, it could be speculated that eggshell particles were not just adsorbed onto the surface but also formed strong bonds with the cell surface, bridging the flocs more firmly and tightly than conventional chemical coagulants.[Citation29] In addition, the rapid harvesting, even at low doses, is one distinct advantage of eggshells.
Effect of settling time
shows the relationship between the separation efficiency and settling time of C. vulgaris in the presence of different concentrations of eggshells. A settling time of 20 min resulted in a separation efficiency of approximately 99 ± 0.3% with 60–120 mg/L of eggshells. However, with eggshell concentrations of 60 mg/L and lower, the settling time needed to achieve 99 ± 0.5% separation increased to 30 min for 60 mg/L of eggshells and to 50 min for 98 ± 0.7% separation with 20–40 mg/L of eggshells. The settling time depends on the floc size: higher floc size increases the free settling velocity compared to that of individual particles which have not formed flocs. Moreover, when the flocs settle faster, the separation efficiency is better.[Citation2,Citation29] It could be hypothesized that, by a mechanism similar to that proposed for chitosan,[Citation29] eggshells might promote faster aggregation of cells through the formation of bridges between the dispersed cells, allowing the formation of particles of sufficient size that settle faster.
Figure 6. Relationship between separation efficiency and settling time of C. vulgaris in the presence of various concentrations of eggshells.
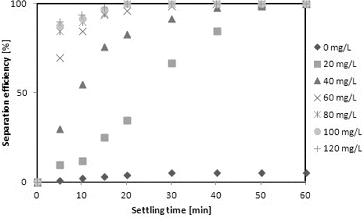
The flocculation method presented in this study has the advantages of simplicity, effectiveness and low cost. Other traditional harvesting methods, e.g. ultrasound, flotation, centrifugation and filtration, which have also been successfully applied to a range of microalgal species, have a higher input cost.[Citation2,Citation30] Also, the viability at small scale may restrict them from large-scale harvesting systems.[Citation11,Citation29] Eggshells as a flocculant could also be considered superior to inorganic flocculants (iron and/or aluminium based), which may hinder further use of the algae biomass in food and feed applications due to toxicity or in fuel production owing to the need for their removal before further processing.[Citation5] Unlike inorganic flocculants, organic ones, such as cationic polyacrylamide, cationic starch and chitosan, are biodegradable and have less or no known toxic effects. They are, however, rather expensive, with a market value of about $US 10 per kilogram for chitosan and $US 1–3 per kilogram for cationic starch.[Citation10,Citation15] In contrast, the eggshells used as flocculants in this study are inexpensive (about $US 0.18 per kilogram) and hence, their utilization could be considered cost effective.[Citation15] What is more, eggshells do not contaminate the growth medium. Such methods are considered advantageous, as the growth medium can be recycled to reduce not only the cost and the demand for water, but also the extra operational costs for reusing growth medium.[Citation2] Thus, the harvesting method proposed here may decrease the cost of biodiesel production from algae.
Conclusions
The results from the present study demonstrate that the separation of high microalgae biomass, using eggshells, could be considered a promising and economical approach due to the low dose requirements and short settling time. The separation efficiency of microalgae biomass was over 99% at the optimal conditions: 80 mg/L flocculant dose (eggshells), 150 r/min mixing rate, 20 min mixing time, 20 min settling time and pH 6. The harvesting of microalgae using waste eggshells is not only effective, but also environmentally-friendly.
Disclosure statement
No potential conflict of interest was reported by the author.
Additional information
Funding
References
- Chisti Y. Biodiesel from microalgae. Biotechnol Adv. 2007;25:294–306.
- Liu J, Tao Y, Zhang Y, Li A, Sang M, Zhang C. Freshwater microalga harvested via flocculation induced by pH decrease. Biotechnol Biofuels [Internet]. 2013;6:98. Available from: http://www.biotechnologyforbiofuels.com/content/6/1/98.
- Pragya N, Pandey KK, Sahoo PK. A review on harvesting, oil extraction and biofuels production technologies from microalgae. Renewable Sustainable Energy Rev. 2013;24:159–171.
- Chen CY, Yeh KL, Aisyah R, Lee DJ, Chang JS. Cultivation, photobioreactor design and harvesting of microalgae for biodiesel production: a critical review. Bioresour Technol. 2011;102:71–81.
- Malm A, Tanner R. Method for harvesting algae. Patent US 2011/0312063 A1; 2011.
- Borowitzka MA, Moheimani NR. Sustainable biofuels from algae. Mitig Adapt Strateg Glob Change. 2013;18:13–25.
- Gerde JA, Yao LY, Lio JY, Wen Z, Wang T. Microalgae flocculation: Impact of flocculant type, algae species and cell concentration. Algal Res. 2014;3:30–35.
- Rashid N, Rehman SU, Han JI. Rapid harvesting of freshwater microalgae using chitosan. Process Biochem. 2013;48:1107–1110.
- Rashid N, Rehman MS, Han JI. Use of chitosan acid solution to improve separation efficiency for harvesting of the microalgae chlorella vulgaris. Chem Eng J. 2013;226(15):238–242.
- Vandamme D, Foubert I, Meesschaert B, Muylaert K. Flocculation of microalgae using cationic starch. J Appl Phycol. 2010;22:525–530.
- Renault F, Sancy B, Badot PM, Crini G. Chitosan for coagulation/flocculation processes – an eco-friendly approach. European Polymer J. 2009;45:1337–1348.
- Cheng YS, Zheng Y, Labavitch J, Vander Gheynst J. The impact of cell wall carbohydrate composition on the chitosan flocculation of Chlorella. Process Biochem. 2011;46:1927–1933.
- Xu Y, Purton S, Baganz F. Chitosan flocculation to aid the harvesting of the microalga Chlorella sorokiniana. Bioresour Technol. 2013;129:296–301.
- Wu Z, Zhu Y, Huang W, Xhang C, Li T, Zhang Y, Li A. Evaluation of flocculation induced by pH increase for harvesting microalgae and reuse of flocculated medium. Bioresour Technol. 2012;110:496–502.
- Podstawczyk D, Witek-Krowiak A, Chojnaka K, Sadowski Z. Biosorption of malachite green by eggshells: mechanism identification and process optimization. Bioresour Technol. 2014;160:161–165.
- Tsai WT, Hsien KJ, Hsu HC, Lin CM, Lin KY, Chiu CH. Utilization of ground eggshell waste as an adsorbent for the removal of dyes from aqueous solution. Bioresour Technol. 2008;99(6):1623–1629.
- Aspelund MT, Rozeboom G, Heng M, Glatz CE. Improving permeate flux and product transmission in the microfiltration of a bacterial cell suspension by flocculation with cationic polyelectrolytes. J Membr Sci. 2008;324:198–208.
- Soletto D, Binaghi L, Ferrari L, Lodi A. Effects of carbon dioxide feeding rate and light intensity on the fed-batch pulse-feeding cultivation of Spirulina platensis in helical photobioreactor. Biochem Eng J. 2008;39:369–375.
- Mezzomo N, Saggiorato AG, Siebert R, Tatsch PO, Lago MC, Hemkemeier M, Costa JAV, Bertolin TE, Colla LM. Cultivation of microalgae Spirulina platensis (Arthrospira platensis) from biological treatment of swine wastewater. Ciênc Tecnol Aliment Campinas. 2010;30(1):173–178.
- Choi HJ. Effect of optical panel distance in a photobioreactor for nutrient removal and cultivation of microalgae. World J Microbio Biotechnol. 2014;30(7):2015–2023.
- Vandamme D, Foubert I, Fraeye I, Meeschaert B, Muylaert K. Flocculation of Chlorella vulgaris induced by high pH: role of magnesium and calcium and practical implications. Bioresour Technol. 2012;105:114–119.
- Sher F, Malik A, Liu H. Industrial polymer effluent treatment by chemical coagulation and flocculation. J Environ Chem Eng. 2013;1(4):684–689.
- Zulfikar MA, Mariske ED, Djajanti SD. Adsotption of lignosulfonate compounds using powdered eggshell. Songklanakarin J Sci Technol. 2012;34:309–316.
- Papazi A, Makridis P, Divanach P. Harvesting Chlorella minutissima using cell coagulants. J Appl Phycol. 2010;2:349–355.
- Putt R, Singh M, Chinnasamy S, Das KC. An efficient system for carbonation of high-rate algae pond water to enhance CO2 mass transfer. Bioresour Technol. 2011;102:3240–3245.
- Salim S, Bosma R, Vermue M, Wijffels RH. Harvesting of microalgae by bioflocculation. J Appl Phycol. 2011;23:849–855.
- Duan J, Wang J, Guo T, Gregory J. Zeta potentials and sizes of aluminum salt precipitates – effect of anions and organics and implications for coagulation mechanisms. J Water Process Eng. 2014;4:224–232.
- Tiller FM, Li W, Chen W. Solid/liquid separation. In: Albright L, editor. Albright's chemical engineering handbook. Boca Raton (FL): CRC Press; 2008. p. 1597–1666.
- Ahmad AL, Mat Yasin NH, Derek CJC, Lim JK. Optimization of microalgae coagulation process using chitosan. Chem Eng J. 2011;173:879–882.
- Salim S, Vermuë MH, Wijffels RH. Ratio between autoflocculating and target microalgae affects the energy-efficient harvesting by bio-flocculation. Bioresour Technol. 2012;118:49–55.