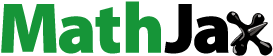
Abstract
Enzymatic preparation of linoleic acid from sunflower oil was examined through a statistical approach. Initially, enzyme screening for oil hydrolysis was investigated. The results indicated that lipases from yeast and bacteria provided higher rates of hydrolysis, when compared to lipases from fungi. In particular, the utilization of Candida rugosa lipase offered the highest hydrolysis rate of 67.12%. Once the optimal lipase for oil hydrolysis was identified, an optimization of the enzymatic process was further investigated. Central composite design was employed in this experiment. The independent variables, affecting the degree of hydrolysis, were found to be buffer-to-oil and enzyme-to-oil ratios, as well as the initial pH (p < 0.05). Statistical analysis suggested that the precision of the predictive model was adequate, due to the low p-values (p < 0.01) and satisfactory levels of the coefficient of determination (R2 = 0.89). In addition, verification of the statistical model suggested that the difference between the predicted and actual degree of hydrolysis was less than 5%. To achieve a hydrolysis degree of 76.07%, the optimal conditions were as follows: buffer-to-oil ratio of 4:1 (w/w), enzyme-to-oil ratio of 750.28 U/g and initial pH of 6.7. Subsequently, urea complex fractionation was performed to enrich the polyunsaturated fatty acids in the enzymatic hydrolysates. The results suggested that the purity of the linoleic acid could be enriched to 70% (w/w). Thus, this process could be used to effectively prepare the linoleic acid as a bio-based ingredient for nutraceutical and cosmetic products.
Introduction
Linoleic acid, an essential fatty acid, is used as a precursor for the biosynthesis of valuable fatty acids, such as γ-linolenic acid, arachidonic acid and prostaglandins.[Citation1,Citation2] Deficiency of the linoleic acid may cause numerous health abnormalities, such as diabetic neuropathy, rheumatoid arthritis, cardiovascular diseases and autoimmune disorders.[Citation3] The linoleic acid is widely applied in nutraceutical and cosmetic products, especially for its effect on the enhancement of skin, as well as for its anti-inflammatory, acne-reducing and moisture retention effects.[Citation4,Citation5] Moreover, the linoleic acid can be converted to conjugated linoleic acids (CLAs) through chemical and biological processes.[Citation6,Citation7] CLAs have several health benefits, such as anticarcinogenic, anti-atherosclerotic, anti-inflammatory and body fat reduction activities.[Citation8,Citation9]
Conventionally, the industrial production of fatty acids is usually conducted via hydrolysis of triglycerides through the Colgate–Emery process and must be carried out at high temperature and pressure levels (250 °C and 5000 kPa, respectively). Then, the desired fatty acid is separated and purified. However, this procedure leads to the formation of undesirable reactions and low purity products, and it is conducted with high-energy consumption. Alternatively, the enzymatic production of fatty acids has gained considerable attention, because it can be done under mild conditions. This process is conducted with low-energy consumption and a high purity of the obtained products is usually achieved.[Citation10,Citation11] Typically, lipase hydrolyses oil to fatty acids at the interphase between oil and aqueous phases. Moreover, this enzyme can catalyse various types of reactions, such as esterification and transesterification. The catalytic ability of lipase comprises several levels of specificities. For example, it is specific to the acyl group, the position and the stereoisomer of the substrate.[Citation12–14] To concentrate the desired fatty acid, a downstream process is usually required. Urea complex fractionation is frequently employed due to simple scale up and recovery and it is a relatively cheap process. This procedure can be applied to eliminate saturated and monounsaturated fatty acids.[Citation15,Citation16] Enzymatic preparation of valuable fatty acids has been widely investigated.[Citation17] For example, the concentration of eicosapentaenoic acid (EPA) and docosahexaenoic acid (DHA) from sardine oil, by using the enzymatic hydrolysis and urea complex fractionation, has been studied. Free and immobilized lipases from Burkholderia cepacia and Pseudomonas sp. were used to hydrolyse sardine oil. By utilizing lipase from B. cepacia as a biocatalyst, 81.5% of EPA and 72.3% of DHA were converted to free fatty acids. Then, urea complex fractionation was employed to enhance the concentrations of EPA and DHA to 46.2% (w/w) and 40.3% (w/w), respectively.[Citation18] The production of an arachidonic acid from single-cell oil through the enzymatic hydrolysis and selective esterification was also demonstrated. Oil hydrolysis, by using various types of lipases as biocatalysts, was investigated. Lipase from Alcaigenes sp. was found to be the most effective biocatalyst. The degree of hydrolysis was achieved to be 98% by using the enzyme loading of 1200 U/g and oil-to-water ratio of 1:2 (w/w) at 40 °C for 48 h. To obtain high purity of the fatty acid [97% (w/w)], a selective esterification was performed.[Citation19] The preparation of the α-linolenic acid from the flax seed oil was also examined. Lipases from Candida rugosa, B. cepacia, Pseudomonas fluorescens and Rhizomucor miehei were used to hydrolyse oil. Based on the high degree of hydrolysis, lipase from C. rugosa was selected for the preparation of the fatty acid. Conversion rate of 89.6% was obtained and purity of the fatty acid was improved to 80% (w/w) by using urea complex fractionation.[Citation20]
In order to enhance the productivity, further optimization of the production is required. Traditionally, optimization of any process requires altering the levels of one variable at a time to evaluate the process response. Therefore, large numbers of experimental trials are typically required. Unfortunately, this approach does not describe the interaction of the variables on the response and it is not assured to reach the optimal conditions for the process. To overcome these limitations, response surface methodology (RSM) is generally applied. RSM is a collection of statistical and mathematical techniques, useful for the development, improvement and optimization of a process. This statistical approach is an effective method to investigate the effects and interactions of the independent variables on the response. Optimal levels of the independent variables are usually obtained.[Citation21] Among several experimental designs, a central composite design (CCD) is commonly used to optimize the production of valuable fatty acids.[Citation22–25] In this study, an optimal preparation of the linoleic acid from sunflower oil was evaluated through the exploration of a suitable biocatalyst and optimal conditions for the oil hydrolysis.
Materials and methods
Materials
Sunflower oil was purchased from the local market (Thanakorn Vegetable Oil Product Co., Ltd, Samutprakan, Thailand). Aspergillus niger lipase (ANL), B. cepacia lipase (BCL), C. rugosa lipase (CRL) and P. fluorescens lipase (PFL) were kindly provided by the Amano Enzyme, Inc. (Nogaya, Japan). Mucor javanicus lipase (MJL) and Rhizopus niveus lipase (RNL) were purchased from Sigma-Aldrich Co., LLC. (St. Louis, MO, USA). Methyl esters of mixed fatty acids were obtained from Supelco Co. (Bellefonte, PA, USA). Borontrifluoride in methanol solution of 14% (v/v) was purchased from Sigma-Aldrich Co., LLC. (St. Louis, MO, USA). All solvents and reagents for the experiments were of analytical grade.
Enzyme screening for oil hydrolysis
Enzymatic hydrolysis of sunflower oil was performed according to previous reports.[Citation10,Citation18] The reaction conditions were as follows: enzyme-to-oil ratio of 500 U/g, sodium phosphate buffer-to-oil ratio of 2:1 (w/w) and initial pH of 7.0. The reaction was conducted at 40 °C and stirred at 500 rpm under nitrogen atmosphere. At specified time intervals, 0.50 g of the samples was withdrawn from the reaction to determine the degree of hydrolysis. The reaction was terminated by adding 50 mL solvent solution, containing ethanol and acetone at the ratio of 1:1 (v/v).
Optimization of the enzymatic hydrolysis
CCD was employed to evaluate the optimal conditions for the enzymatic hydrolysis. The examined variables were initial pH, enzyme-to-oil ratio, buffer-to-oil ratio and reaction time. In general, the variables can be described according to EquationEquation (1)(1)
(1) :
(1)
(1) where xi is the coded value of the independent variable, Xi is the real value of the independent variable, X0 is the real value of the independent variable at the centre point and △Xi is the step change value.
The levels of the variables in CCD are shown in . The experimental design consisted of 30 treatments (16 trials of 24 full factorial design, 8 trials of axial points and 6 replicates of the central points). The degree of hydrolysis was selected as the response. The predictive model was expressed as a quadratic polynomial equation, according to EquationEquation (2)(2)
(2) :
(2)
(2) where Y is the response, β0 is the intercept, βj, βjj and βjk are the linear, quadratic and interaction coefficients, respectively, and Xj and Xk are the coded values of the independent variables.
Table 1. Levels and values of the independent variables for the central composite design.
Enrichment of the linoleic acid by using urea complex fractionation
The fatty acids in hydrolysates were enriched through urea complex fractionation, according to a previous study.[Citation18] Briefly, 15.0 g of the resulting fatty acids was mixed with 100 mL of 25% (w/v) urea in ethanol at 60 °C with stirring, until the mixture was turned into a clear homogeneous solution. The mixture was allowed to crystallize at 4 °C for 10 h with stirring. The crystals were then removed by filtration. Finally, the composition of the total fatty acids in the crystals and filtrates were determined by gas chromatography.
Determination of the degree of hydrolysis
The degree of hydrolysis was determined by titration of the released fatty acids against 25 mmol/L NaOH solution. Briefly, the degree of hydrolysis was defined as the weight per cent of the free fatty acids in the sample to the maximum theoretical amount, according to EquationEquation (3)(3)
(3) .[Citation10]
(3)
(3) where VNa is the required volume of the NaOH solution during titration (L), CNa is the molar concentration of NaOH (mol/L), MW is the average molecular mass of fatty acids in sunflower oil, Wt is the weight of oil in the sample (g) and f0 is the fraction of oil in the mixture at the beginning of the reaction.
Composition of fatty acids
During the urea complex fractionation, the composition of total fatty acids in the crystals and filtrates was analysed, according to a previous report.[Citation18] All of the extracted fatty acids were methylated to fatty acid methyl esters (FAMEs), according to standard protocols.[Citation26] Methylation of the fatty acids was performed with 14% (v/v) boron trifluoride in the methanol solution at 55 °C for 5 min. FAMEs were extracted by the addition of distilled water and n-hexane. The hexane layer was collected and analysed by a gas chromatography (Shimadzu GC 2014, Kyoto, Japan) equipped with a flame ionization detector. A capillary column (30 m × 0.25 µm × 0.25 µm; DB-5) was used. The injector and flame ionization detector temperatures were 250 °C and 260 °C, respectively. The initial oven temperature was increased from 150 °C to 220 °C at 10 °C/min and held at 220 °C for 10 min. Helium was used as a carrier gas at 87.5 kPa. FAMEs were identified by comparison with standard and quantified as the weight percent.
Statistical analysis
Design Expert version 7.0.0 (Stat-Ease, Minneapolis, MN, USA) was used for the statistical and graphical analyses of the experimental data. The statistical significance was evaluated by using Fisher's F-test. The quality of the predictive model was statistically evaluated by using the coefficient of determination. All experiments were carried out in triplicate and the average values were reported.
Results and discussion
Enzyme screening for oil hydrolysis
Several types of lipases were used to hydrolyse the sunflower oil in the preparation of the linoleic acid. In general, the degree of hydrolysis was observed to initially increase and, subsequently, remain at a constant level ( and ). When fungal lipases were used as biocatalysts, the degree of hydrolysis steadily increased within the first 6 h to a relatively stable rate at 27%–43% (). The higher conversion rate of 42.76% was obtained by using MJL as a catalyst. When bacterial lipases (PFL and BCL) were used as biocatalysts, the conversion rates were observed to gradually increase within the first 12 h to stable rates of 42.44% and 54.46%, respectively (). When yeast lipase (CRL) was used as a biocatalyst, the degree of hydrolysis rapidly increased within the first 6 h and then steadily improved to 67.12% (). According to these results, the optimal lipase for the hydrolysis of sunflower oil was found to be CRL. These results were comparable with several reports. CRL has been shown to be an effective biocatalyst with high conversion rates in the hydrolysis of oil from several sources.[Citation20,Citation27–29]
Figure 2. Time course of the enzymatic hydrolysis by using lipases from bacteria and yeast as biocatalysts.
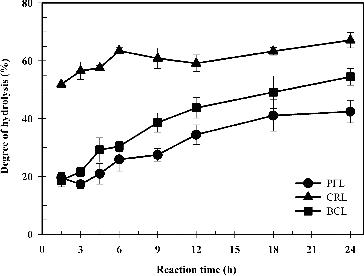
Typically, the catalytic activity of lipase toward triacylglycerols depends on its preference for fatty acids. This could be related to the structure of the binding site. Crevice-, funnel- and tunnel-like conformations have been observed in most binding sites. Lipases containing tunnel-like binding sites prefer to hydrolyse plant oil with long-chain fatty acids more than enzymes with crevice- and funnel-like binding sites.[Citation14] Moreover, a tunnel-like conformation is sterically suitable for the oleic acid.[Citation12] This unique conformation was found only in CRL and Geotrichum candidum lipase. Lipases from Pseudomonas sp. and fungi, such as Rhizomucor miehei lipase (RML) and RNL, exhibit a funnel- and crevice-like binding sites.[Citation29] Utilization of CRL as a biocatalyst for the preparation of polyunsaturated fatty acids (PUFAs) has been widely reported. For example, the enzymatic preparation of the α-linolenic acid from the flax seed oil was performed by using selective hydrolysis. Lipases from several sources, such as CRL, BCL, PFL and RML were examined. The results showed that CRL exhibited the highest degree of hydrolysis, because it preferentially hydrolysed the α-linolenic acid to a greater degree than the other lipases. A hydrolysis degree of 89.6% was obtained by using an enzyme loading of 300 U/g, buffer-to-oil ratio of 20:1 (v/w), initial pH of 7.5, temperature of 35 °C and reaction time of 2 h.[Citation20] CRL was also found to be a suitable biocatalyst for the selective hydrolysis of the soybean oil in the preparation of the stearidonic acid. A reaction rate of 66.20% was obtained at 4 h of incubation time by using the enzyme loading of 3218 U/g, oil-to-buffer ratio of 1:1.5 (w/v), initial pH of 7.5 and temperature of 40 °C.[Citation29] Similarly, the selective hydrolysis of salmon oil for the enrichment of omega-3 PUFAs was examined. Among the tested lipases, CRL was found to be the most active biocatalyst in the hydrolysis to produce the concentrated PUFAs of 27.81% (w/w). The highest hydrolysis degree of 91.89% was achieved after 24 h of incubation by using oil-to-water ratio of 1:2 (w/v) and the enzyme concentration of 40 U/g at 37 °C.[Citation28]
Optimization of the enzymatic hydrolysis
Once a suitable lipase for the sunflower oil hydrolysis was identified, RSM was used as a tool to determine the optimal conditions for the enzymatic process. In this study, the variables, supposed to affect the degree of hydrolysis, were initial pH, enzyme-to-oil ratio, buffer-to-oil ratio and reaction time. A CCD containing 16 treatments of 24 full factorial trials, 8 treatments of axial points and 6 replicates of centre points is displayed in . The results demonstrated that the degree of hydrolysis fluctuated from 38.67% to 77.85%. A higher degree of hydrolysis (>69%) was obtained when the independent variables were set to the centre point. Moreover, the initial pH, enzyme-to-oil and buffer-to-oil ratios appeared to be the variables that significantly affected the degree of hydrolysis, as evidenced by the significant differences in responses, when these variables were altered (i.e., experiment number of 17–24). In addition, the statistical analysis indicated that these variables significantly affected the degree of hydrolysis (p < 0.05). The calculated F-values of 9.16 and the low p-values of the model implied that the model had a high level of confidence (p < 0.01). The goodness, degree of precision and reliability of the model were evaluated according to the coefficient of determination (R2), the lack-of-fit and the coefficient of variation (CV). R2 values of 0.8953 suggested a high correlation between the experimental and the predicted values. The non-significance of the lack-of-fit (p > 0.05) and the low values of CV (5.92%) indicated that the model was precise and reliable (). According to EquationEquation (4)(4)
(4) , the model consisted of three linear effects and two squared effects. The positive coefficients in each term of the equation suggested that the independent variables had a linear effects on the response.[Citation21]
(4)
(4) where Y is the degree of hydrolysis; X1, X2 and X3, are the coded values of enzyme-to-oil ratio, initial pH and buffer-to-oil ratio, respectively.
Table 2. Central composite design with the predicted and the experimental values of the hydrolysis degree by using CRL as a biocatalyst.
Table 3. Analysis of variance for the response of hydrolysis degree by using CRL as a biocatalyst.
A response surface plot was used to observe the effects and interactions of the independent variables. Effects of enzyme–to-oil and buffer-to-oil ratios on the degree of hydrolysis are shown in . The influence of these two variables on the response was similar. The degree of hydrolysis improved at low levels of the variables to an approximately constant rate at higher levels of the variables. Likewise, shows the effects of the initial pH and buffer-to-oil ratio on the degree of hydrolysis. At a low level of buffer-to-oil ratio, the increase in the initial pH did not substantially affect the degree of hydrolysis. However, at a high level of buffer-to-oil ratio, the conversion rate decreased at elevated levels of the initial pH. Apart from the type of biocatalyst, several reaction parameters could affect the reaction rate. In this study, the buffer-to-oil and enzyme-to-oil ratios as well as the initial pH were found to be the parameters that significantly affected the degree of hydrolysis (p < 0.05). Typically, lipase-catalysed hydrolysis would not occur, because the substrate could not access to the catalytic triad. To initiate the activity of the enzyme, the lid has to leave the active site in order to gain substrate accessibility. The catalytic activity initially occurs after interfacial activation at the interface area of the water and oil phases.[Citation13] In this case, contents of buffer and oil during the hydrolysis could affect the interfacial dynamics between lipase and oil. At a high oil content, reduction of the catalytic activity is observed, due to the diminished access to the active centre and the aggregation of oil droplets around the active sites and substrate ().[Citation24,Citation30] Therefore, the effect of enzyme-to-oil ratio on the hydrolytic activity is associated with a limitation of the interfacial area between the biocatalyst and oil. At a high content of the enzyme loading, the degree of hydrolysis does not significantly increase because the contact area is occupied by activated lipases and the accumulated intermediates (). [Citation22,Citation23,Citation25,Citation27] In addition, at a high level of buffer-to-oil ratio, the conversion rate decreased with elevated levels of the initial pH (). This change could be attributed to the altered ionization of the protein structure, the ionic stage of the substrate and the catalytic ability of the enzyme.[Citation23,Citation25] These results were also reported in previous studies.[Citation25,Citation30] Statistical optimization of the erucic acid production from mustard oil was performed. CCD was used to optimize the enzymatic hydrolysis. Among several variables, the initial pH and the buffer concentration were found to significantly impact the yield of the fatty acid (p < 0.01). Lipase denaturation and product degradation occurred at extreme pH values. However, the catalytic activity improved at low buffer concentrations. The optimal conditions improved the fatty acid yield to 43.08% (w/w).[Citation25] The optimal conditions for lipase-catalysed hydrolysis of linseed oil were investigated. The effect of several parameters, including temperature, initial pH, oil-to-aqueous phase ratio, enzyme loading and reaction time on the degree of hydrolysis, were evaluated by using the Box–Behnken design. All variables were found to significantly affect the response (p < 0.05). A higher reaction temperature affected the response, owing to the increased probability of the substrate collision and the inactivation of the enzyme. High values of the oil-to-aqueous phase ratio and the initial pH decreased the hydrolytic activity as a result of the substrate inhibition and enzyme inactivation. High levels of enzyme loading did not improve the hydrolytic activity, because the interfacial area was saturated with the enzyme molecules. In addition, long periods of reaction time diminished the enzyme activity, owing to the reduction of the substrate concentration. The hydrolysis of linseed was enhanced to 93.93%.[Citation30]
Model validation
Model validation was performed at the optimal conditions. The results revealed that the difference between the predicted and the experimental responses was approximately 5%. To achieve a hydrolysis degree of 76.07%, the optimal conditions for oil hydrolysis were as follows: enzyme-to-oil ratio of 750.28 U/g, initial pH of 6.7, buffer-to-oil ratio of 4:1 (w/w) and reaction time of 19 h. The highest degree of hydrolysis was 96.72%, which was more than the minimum level of the response obtained from CCD.
Enrichment of the linoleic acid by using urea complex fractionation
Urea complex fractionation was used to concentrate the linoleic acid in the hydrolysates. The fatty acid composition of the crystals and filtrates during fractionation is depicted in . The results demonstrated that most of the saturated fatty acids (i.e., palmitic and stearic acids) and some oleic acid were removed from the non-urea complex fraction. The content of the linoleic acid in the fraction was enhanced to 70.07% (w/w).
Table 4. Composition of fatty acids in each fraction of urea complex fractionation.
Conclusions
In this study, an enzymatic preparation of the linoleic acid from sunflower oil was developed. The statistical approach is proven to be an effective tool for the optimization of the lipase-catalysed hydrolysis. The predicted model was reliable and displayed an adequate precision. Under the optimal conditions, the degree of hydrolysis was enhanced to about 76%. Urea complex fractionation was employed to achieve high purity of the desired fatty acid. By using the developed process, the bio-based linoleic acid can be directly used as a feed stock for the production of CLAs as well as a bioactive ingredient for nutraceutical and cosmetic products.
Acknowledgements
The authors would like to acknowledge Amano Enzymes Inc. (Nagoya, Japan) to provide enzymes in the research project.
Disclosure statement
No potential conflict of interest was reported by the authors.
Additional information
Funding
References
- Das UN. Essential fatty acids: biochemistry, physiology and pathology. Biotechnol J. 2006;1(4):420–439.
- Ruiz-Rodriguez A, Reglero G, Ibanez E. Recent trends in the advanced analysis of bioactive fatty acids. J Pharma Biomed Anal. 2010;51:305–326.
- Simopoulos AP. Essential fatty acids in health and chronic disease. Am J Clin Nutr. 1999;70(3):560s–569s.
- Lee MH, Kim HJ, Ha DJ, et al. Therapeutic effect of topical application of linoleic acid and lincomycin in combination with betamethasone valerate in melasma patients. J Korean Med Sci. 2002;17(4):518–523.
- Zhao G, Etherton TD, Martin KR, et al. Anti-inflammatory effects of polyunsaturated fatty acids in THP-1 cells. Biochem Biophys Res Commun. 2005;336:909–917.
- O'Quinn PR, Nelssen JL, Goodband RD, et al. Conjugated linoleic acid. Anim Health Res Rev. 2000;1(1):35–46.
- Andrade JC, Ascencao K, Gullon P, et al. Production of conjugated linoleic acid by food grade bacteria: a review. Int J Dairy Technol. 2012;65(4):467–481.
- Bhattacharya A, Banu J, Rahman M, et al. Biological effects of conjugated linoleic acids in health and disease. J Nutr Biochem. 2006;17(12):789–810.
- Park Y. Conjugated linoleic acid (CLA): good or bad trans fat. J Food Compost Anal. 2009;22S:S4–S12.
- Rooney D, Weatherley LR. The effect of reaction conditions upon lipase catalysed hydrolysis of high oleate sunflower oil in a stirred liquid–liquid reactor. Process Biochem. 2001;36:947–953.
- Hayes DG. Enzyme-catalyzed modification of oilseed materials to produce eco-friendly products. J Am Oil Chem Soc. 2004;81(12):1077–1103.
- Domínguez de María P, Sánchez-Montero JM, Sinisterra JV, et al. Understanding Candida rugosa lipases: an overview. Biotechnol Adv. 2006;24(2):180–196.
- Reis P, Holmberg K, Watzke H, et al. Lipases at interfaces: a review. Adv Colloid Interface Sci. 2009;147–148:237–250.
- Kapoor M, Gupta MN. Lipase promiscuity and its biochemical applications. Process Biochem. 2012;47:555–569.
- Wanasundara UN, Wanasundara PKJPD, Fereidoon S. Novel separation techniques for isolation and purification of fatty acids and oil by-products. In: Fereidoon S, editor. Bailey's industrial oil and fat products. New York (NY): John Wiley & Sons; 2005. p. 585–621.
- Shimada Y. Lipase reactions applicable to purification of oil- and fat-related materials. In: Akoh CC, Lai OM, editors. Healthful lipids. Champaign, IL: AOCS press; 2005. p. 395–410.
- Piazza GJ, Foglia TA, Xu X. Lipase-catalyzed harveating and/or enrichment of industrially and nutritionally important fatty acid. In Rastall R, editor. Novel enzyme technology for food application. Cambridge: Woodhead publishing; 2007. p. 285–313.
- Gámez-Meza N, Noriega-Rodrı́guez JA, Medina-Juárez LA, et al. Concentration of eicosapentaenoic acid and docosahexaenoic acid from fish oil by hydrolysis and urea complexation. Food Res Int. 2003;36:721–727.
- Yamauchi A, Nagao T, Watanabe Y, et al. Purification of arachidonic acid from mortierella single-cell oil by selective esterification with Burkholderia cepacia lipase. J Am Oil Chem Soc. 2005;82(11):833–837.
- Rupani B, Kodam K, Gadre R, et al. Lipase-mediated hydrolysis of flax seed oil for selective enrichment of α-linolenic acid. Eur J Lipid Sci Technol. 2012;114:1246–1253.
- Montgomery DC. Design and analysis of experiments. 5th ed. New York (NY): John Wiley & Sons; 2001.
- Teng D, Le R, Yuan F, et al. Optimization of Enzymatic hydrolysis of chicken fat in emulsion by response surface methodology. J Am Oil Chem Soc. 2009;86:485–494.
- Goswami D, Sen R, Basu JK, et al. Maximization of bioconversion of castor oil into ricinoleic acid by response surface methodology. Bioresource Technol. 2009;100:4067–4073.
- Avelar MHM, Cassimiro DMJ, Santos KC, et al. Hydrolysis of vegetable oils catalyzed by lipase extract powder from dormant castor bean seeds. Ind Crops Prod. 2013;44:452–458.
- Goswami D, Basu JK, De S. Optimal hydrolysis of mustard oil to erucic acid: a biocatalytic approach. Chem Eng J. 2012;181–182:542–548.
- AOCS. Official methods and recommemded pratices of American oil chemists' society, 5th ed. Champaign (IL): American Oil Chemists' Society; 1998.
- Brijwani K, Vadlani PV. Lipase-mediated hydrolysis of corn DDGS oil; kinetics of linoleic acid production. Biochem Eng J. 2010;52:289–295.
- Kahveci D, Falkeborg M, Gregersen S, et al. Upgrading of farmed salmon oil through lipase-catalyzed hydrolysis. Open Biotechnol J. 2010;4:47–55.
- Kleiner L, Vázquez L, Akoh CC. Lipase-catalyzed concentration of stearidonic acid in modified soybean oil by partial hydrolysis. J Am Oil Chem Soc. 2012;89:1999–2010.
- Chen W, Sun S, Liang S, et al. Lipase-catalyzed hydrolysis of linseed oil: optimization using response surface methodology. J Oleo Sci. 2014;63(6):619–628.