Abstract
MircroRNAs (miRNAs) are small non-coding RNAs about 21 nt in length. These short transcripts regulate developmental and stress responses in plants. Cold stress is one of the most restraining abiotic factors adversely affecting the plant yield. In the present study, some cold stress-related miRNAs (miR167, miR169, miR172, miR393 and miR397) in tomato (Solanum lycopersicum) were assessed at early time points (0, 1, 4 and 16 h) of cold exposure. Relative expression of miRNAs was measured by stem–loop quantitative reverse transcription polymerase chain reaction. The results showed that miR167, miR169, miR172 and miR393 were activated in the early time points of cold treatment. Especially, miR172 was found to have highest expression level. Furthermore, target genes of selected miRNAs were identified and their expression profiles were assessed between cold-sensitive and cold-tolerant cultivars of tomato. It was found that inferred expression patterns of target genes were differentiated between the cultivars. Analysis of cis-acting elements showed that miRNAs had stress-responsive elements. Meanwhile, since no miR393 sequence is available, putative miR393 sequence and its secondary structure were predicted in tomato. These results may provide a framework for further analysis in terms of understanding the response of miRNAs against cold stress in tomato.
Introduction
Plant microRNAs (miRNAs), which are non-coding short transcripts, play a key role in development and stress response in plants. To date, hundreds of miRNAs have been found and characterized. miRNAs are often located in gene families and conserved between closely related species.[Citation1–3] They repress gene expression by base-pairing with nearly perfect complementarity against coding sequences, leading to cleavage or translational repression of mRNAs.[Citation4–6] Thus, miRNAs regulate the gene expression post-transcriptionally.[Citation7] miRNAs have been shown to be involved in the response to various stress conditions.[Citation8–10] In Arabidopsis, miR398 was identified to target two closely related Cu/Zn superoxide dismustase genes, and down-regulation of miR398 led to improved tolerance of transgenic lines under oxidative stress.[Citation11] miR395 and miR399 were found to be involved in sulphate and phosphate starvation.[Citation12,Citation13] In another study, miR393 was strongly induced by cold stress.[Citation8] In addition, miRNAs regulate many developmental processes, including leaf development, auxin signalling, phase transition, flowering and genome maintenance.[Citation14–21] The knowledge about miRNAs mostly comes from Arabidopsis and accumulation of new data is still an ongoing process. To date, miRNAs have been identified and detected using high-throughput sequencing, cloning and bioinformatic approaches.[Citation22–24] Conventional technologies such as northern hybridization and microarray analysis have been widely used for investigation of miRNAs but it was reported that it may not be sensitive enough to detect less abundant miRNAs.[Citation25] Among the many techniques, stem–loop reverse transcription polymerase chain reaction (stem–loop RT–PCR) provides more specificity and sensitivity since it uses linear primers due to base-stacking and spatial constraint of the stem–loop structure.[Citation25,Citation26] Furthermore, detection sensitivity is increased by a pulsed RT reaction.[Citation27]
Cold stresses, such as chilling and freezing, affect the agricultural productivity around the world.[Citation28] Numerous physiological and molecular changes occur during cold acclimation.[Citation29] Among them, repression of genes, mRNA export and mRNA degradation have been found to be of central importance for the cold-stress response.[Citation29,Citation30] However, knowledge on the role of miRNA in cold stress is still limited compared to other abiotic stresses. Therefore, elucidating the cold-stress-regulated miRNAs and determination of their expression profile could improve our understanding of miRNAs and their functions in biological systems. Recently, expression profiles of all known miRNAs under cold stress in Arabidopsis, poplar and rice were validated with microarray analyses.[Citation19,Citation24,Citation28] Zhou et al. [Citation7] established a machine-learning transcriptome-based approach for annotating cold-inducible miRNAs in Arabidopsis. They found that miR165/166, miR169, miR172, miR393, miR396 and miR397 were over expressed in cold stress. They also formed a sketch pathway, implying possible regulatory role of miR169. Tang et al. [Citation31] found up-regulation of miR167 and role of trans-acting short-interfering RNA–auxin response factor (tasiRNA–ARF) in regulating the auxin-signalling pathway and possibly in the developmental response to cold stress in wheat. In rice, Jian et al. [Citation32] found several cold-regulated miRNAs by direct cloning and sequencing. Zhang et al. [Citation33] identified 28 cold responsive miRNAs in Brachypodium.
Plants from temperate regions are tolerant to chilling but not to freezing; however, they can increase their freezing tolerance by being exposed to chilling temperatures, by a process known as cold acclimation.[Citation34,Citation35] On the contrary, tropical and subtropical plants, such as rice, maize and tomato are sensitive to cold stress and largely lack the capacity for cold acclimation.[Citation35] Tomato suffers from chilling injuries at all stages of growth and development.[Citation36] Prolonging cold temperature can further reduce the plant production and growth.
It was predicted that there are many cold-induced miRNAs in plant species but knowledge about them is still limited. In this study, we investigated cold-induced miRNAs (miR167, miR169, miR172, miR397 and miR393) [Citation7,Citation8,Citation31] by a sensitive method, stem–loop qRT–PCR, in cold-treated tomato plants. In order to understand the expression profiles of miRNA target genes, we also retrieved the microarray data of three tomato cultivars from a public database.
Materials and methods
Plant growth and cold treatment
Tomato (Solanum lycopersicum var. H-2274) plants were grown in soil under greenhouse conditions with a 16:8 h (light:dark) photoperiod at 25 °C ± 2 °C. After 4 weeks of growth, plants were subjected to cold treatment (4 °C for 1, 4 and 16 h) with a light intensity of 20 µmol/(m2 s).[Citation37] Then plant leaves were quickly harvested and stored at –80 °C. Control plants were not subjected to cold treatment.
Preparation of RNA and cDNA
Total RNA of the leaf samples were extracted using Trizol® (Invitrogen) reagent, according to the manufacturer's instructions. Extracted RNA was dissolved in Rnase-free water and stored at −80 °C. RNA integrity was observed in a 2% agarose gel; three bands corresponding to ribosomal RNA (28S, 18S and 5S) were apparent. RNA concentration was calculated by using Cubit flour metric system (Invitrogen). An amount of 500 ng of total RNA was treated with 5 U of Rnase-free recombinant DNase I® (Roche) and incubated at 37 °C for 15 min. Reaction was stopped by adding 2 µL of 0.2 mol/L ethylenediaminetetraacetic acid (pH 8.0) to a final concentration of 8 mmol/L and heating to 75 °C for 10 min. Stem–loop RT primers were designed according to Varkonyi-Gasic et al. [Citation25]. RT reaction contained 30 ng RNA, 1 µL stem–loop RT primer (1 µmol/L) and 0.25 mmol/L deoxyribonucleoside triphosphates. The mixture was incubated at 65 °C for 5 min and then 5× reverse transcripatase buffer, 1 µL 20 units RiboLock RNase inhibitor, 1 µL RevertAid M–MuLV RT (Thermo Scientific™ RevertAid™ First Strand Synthesis Kit) and Rnase-free water were added into the mixture up to 20 µL per sample. This mixture was incubated for 30 min at 16 °C, followed by 60 cycles at 30 °C for 30 s, 42 °C for 30 s and 50 °C for 1 s for pulsed RT. Finally, reverse transcriptase was inactivated by incubation at 70 °C for 15 min.
Stem–loop reverse transcription–PCR
Prior to quantification analysis, miRNAs of interest were amplified by conventional PCR (Techne® TC512 Gradient Termal Cycler) and checked in a 2% agarose gel ((a)). For quantification analysis, primer-specific regions of targeted miRNAs were amplified using Luminaris™ Color HiGreen qPCR Master Mix kit (Thermo Scientific) and real-time PCR (qPCR) was performed in a RotorGene PCR machine (Qiagen). A miRNA-specific primer and a universal reverse primer were used for amplification of individual miRNAs (). Then, 0.5 μmol/L of each forward and reverse primer with 2 µL cDNA was mixed with 10 µL of qPCR Master Mix. The following program was set up: 95 °C for 10 min, 40 cycles of 95 °C for 15 s, 58 °C for 30 s, 72 °C for 30 s and melting analysis at a temperature gradient from 57 °C to 95 °C with an increment of 0.5 °C/min.
Figure 1. Expression of miRNAs in tomato at 1, 4 and 16 h of cold stress. (a) Agarose gel showing amplification of miRNAs. C – control. (b) Relative expression level of tomato miRNAs.
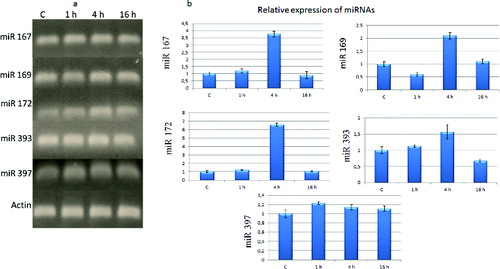
Table 1. Primers used for stem-loop qRT–PCR.
Relative quantity of individual transcripts was calculated by using a mathematical model, including an efficiency correction and crossing point (Cp) deviation of an unknown sample versus a control.[Citation38] Tomato actin gene was used as internal control for qPCR analysis. The fold change (FC) in the expression of each miRNAs was computed according to Pfaffl [Citation38].
Expression analysis of target gene under cold stress
Mature miRNA sequences were obtained from miRBase (http://www.mirbase.org).[Citation39] Target predictions were performed using the psRNATarget (http://plantgrn.noble.org/psRNATarget/) [Citation40] with default settings. We also extracted the expression profile of five miRNAs’ target genes from microarray data for three cold-treated tomato cultivars (LA1777, LA3969 and LA4024) in the Tomato Functional Genomics Database (http://ted.bti.cornell.edu/). The data was normalized using Print-tip lowess normalisation method.[Citation36] We also compiled some target genes, which were reported from previous studies. However, expressions of some target genes were not available in databases. Thus, we excluded these targets. Probe sequences were further used as query sequences for the BLASTN search against SGN tomato whole-genome chromosome database (http://solgenomics.net/tools/blast/index.pl) and NCBI (http://blast.ncbi.nlm.nih.gov/Blast.cgi#). Gene annotation analysis was performed using the Tomato Functional Genomics Database. The microarray data was deposited in the Tomato Functional Genomics Database with accession number E060,[Citation36] for three tomato genotypes. The cold tolerance level of these genotypes was reported by Liu et al. [Citation36]. In this manner, we were able to comparatively investigate how the expression profile of target genes differs among cold-tolerant and cold-sensitive tomato cultivars. Statistical analysis was carried out using the data obtained from three separate sets of biological samples. One-way analysis of variance with the post hoc test was performed using SPSS 18.0 for Windows. P < 0.05 was considered to be statistically significant.
Isolation of sly-miR393 and cis-elements of miRNAs
The pre-miRNA sequences for each of miRNA were obtained from miRBase and mapped to the sequences of tomato genome (http://www.phytozome.net/) using the BLAST search. Blast parameters were adjusted as follows: an expected value cutoff of 1; a low-complexity sequence filter; 1000 descriptions and alignments, and automatically adjusted parameters for short input sequences to improve the accuracy of outputs. For each miRNA, 1 kb of upstream putative promoter sequence was retrieved.[Citation19] PlantCARE (http://bioinforma tics.psb.ugent.be/webtools/plantcare/) was used to identify and categorize the cis-acting elements. The secondary structures of putative miR393 sequence in tomato and minimal folding free energies (MFEs ΔG in kcal/mol) were predicted by using the web-based software Mfold 2.3 (http://mfold.rit.albany.edu/?q = mfold/RNA–Folding–Form). Then, adjusted MFE and MFE index (MFEI) were calculated according to the previous report.[Citation41]
Results and discussion
Expression patterns of tomato miRNAs
The obtained results showed that miR167 was induced by approximately four FC comparing to the control group at 4 h of cold treatment ((b)). After 16 h of cold treatment, its transcript accumulation was decreased to control level. Transcript accumulation of miR169 was decreased at 1 h and then increased at 4 h of cold treatment. Expression of miR169 was not altered in comparison to control after 16 h of cold exposure. miR172 was induced to a highest extent among the tested miRNAs. Especially, its expression was significantly increased over six times at 4 h. Similar to miR167, its expression pattern showed a decrease in expression at 16 h of cold treatment as that in the control. The transcript abundance of miR393 was nearly 1.6 FC increased at 4 h. The expression level of miR393 was lower than that in the control at 1 h, whereas cold treatment did not significantly change the expression of miR397.
When we compared the expression profile of miRNAs with that in the control group, it was miR172 that stood out as the most induced one ((b)). Following miR172, the levels of expression of miR167 and miR169 were higher than that of other miRNAs. Interestingly, it was indicated that 4 h of cold treatment induced expression of miR172, miR167, miR169 and miR393. After 4 h, the expression of those miRNAs decreased but nevertheless they showed a nearly constant expression profile compared to the control. We suggest that 4 h of cold treatment was a suitable duration for up-regulation of miR167, miR169, miR172 and miR393 in tomato plants.
Isolation of sly-miR393 and cis-element analysis of miRNAs
We should note that miR393 expression in tomato was previously reported [Citation42,Citation43] but no sequence was deposited in any database. Using Solanum tuberosum (potato) and Arabidopsis mature miR393 sequences, which are well conserved, we identified putative mature sly-miR393 sequence location (100% identity with mature miRNA sequences of potato and Arabidopsis). Additionally, we tried to elucidate the sly-miR393 sequence by blasting stem–loop miRNA sequences of potato and Arabidopsis against the tomato genome (http://www.phytozome.net/), and only potato miRNA sequences showed 93% identity (100% coverage; data not shown) with tomato sequence in the same location as those of mature sequences of both plants. The matched sequences were obtained and further screened for the potential hairpin structure by an RNA-fold prediction program. The output of the program, which gave rise to the characteristic stem–loop structure, is shown in (a). The MFE of putative sly-miR393 was –39.30 kcal/mol and the MFEI was 1.22. This finding indicated that the miRNA precursor sequence had a significantly higher MFEI value than other non-coding or coding RNAs, e.g. tRNA, rRNA.[Citation44] Furthermore, putative mature sly-miR393 sequences are in the stem portion of hairpin structures ((a)).
Figure 2. Secondary structure of putative pre-miR393 in tomato (a); horizontal red line indicates mature sequences in the stem region. (b) Graphical representation of cis-acting elements in promoter region.
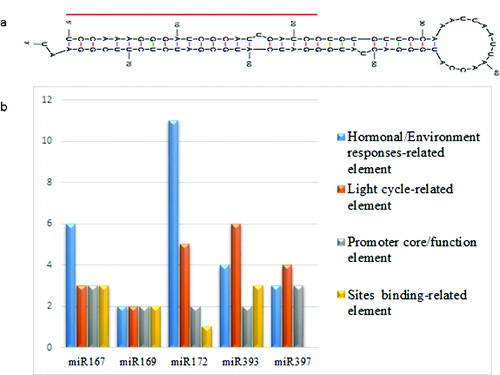
The gene expression level largely depends on cis-elements existing in the promoter region.[Citation45] Cis-elements analysis could also be an effective approach for the miRNAs as for protein-coding genes.[Citation46,Citation47] In the analysis, unknown cis-elements were not included. Cis-elements were heterogeneously distributed among miRNAs. The investigation revealed that abscisic acid (ABA)-response elements (ABRE, miR172 and miR393), ethylene-responsive element (ERE, miR172) and MYB-binding site involved in drought inducibility (miR167 and miR172), and defence- and stress-responsive elements (TC-rich repeats, miR167, miR172 and miR393), heat-stress-responsive elements (HSE, miR169, miR172 and miR397), light-responsive elements (G-Box, miR172, miR393 and miR397), and auxin-responsive elements AuxRR-core (miR167 and miR169) were present in miRNAs (Supplementary Table S1). When comparing miRNA cis-elements, it was found that these cis-acting regulatory elements were mainly related to two physiological processes: light cycle and hormonal/environmental responses ((b) and Supplementary Table S1). miR172 had 11 cis-acting elements related to hormonal/environmental responses, while there were two cis-acting elements in miR169. On the other hand, miR393 had six cis-acting elements related to light cycle, whereas miR169 had two cis-acting elements. In the analysis, it the least number of cis-acting elements were found in miR397, which coincided with the low level of expression. In addition, some other cis-acting elements were also found, such as those possibly involved in regulation in response to methyl jasmonate, gibberellic acid, ethylene and salicylic acid. The transcription levels of miR172 and miR167 were higher than those of other miRNAs. Presumably, this could be related to higher number of hormonal/environmental responsive cis-acting elements contained in them.
Predicted target genes
We also identified potential target genes. Currently, bioinformatics tools facilitate revealing of target sequences based on the high degree of homology between miRNAs and target genes.[Citation48] Most target genes are highly conserved across plants. The targets found in the analysis are shown in . Moreover, we investigated the target genes from which they had been previously identified in the literature. AFR6 targeted by miR167 [Citation49] and TIR targeted by miR393 [Citation31,Citation50] were included in target gene expression analysis.
Table 2. Putative miRNA target genes, expectation value and expression level of three tomato genotypes.
The inferred expression of homeobox–leucine zipper protein 22 (HD–ZIP III; SGN-U216828) and annexin (SGN-U214256) targeted by miR167 were more induced in cold-sensitive (LA4024) than in cold-tolerant tomato cultivars (LA1777 and LA3969) under cold treatment (). Similarly, HD–ZIP III expression was increased in cold-stored potato tubers.[Citation35] SGN-U214038 and SGN-U214039 encoding multi-drug resistance protein mtdK showed higher FC in cold-tolerant cultivar LA1777 (4.9 and 5.5 FC, respectively). However, their expression in cv. LA3969 (1.6 FC) displayed lower pattern than cv. LA1777 but higher than cv. LA4024. SGN-U215435 (receptor-like protein kinase; RLK) was highly up-regulated in all cultivars. Similarly, in Arabidopsis, RLK1 was induced by several environmental stresses, such as dehydration, high salt and cold treatment.[Citation51] Expression of AFR8 (SGN-U240026 and SGN-U227556) targeted by miR167 was repressed in cv. LA1777 and cv. LA4024. On the other hand, they were not significantly changed in cv. LA3969. Interestingly, the LA3969 cultivar showed higher expression profile of ARF6 (SGN-U230670) than others (1.7 FC). Following this observation, ARF6 transcript accumulation was decreased in LA1777, but it was not changed in LA4024. Although SGN-U214629 (ATP synthase I–like protein) was slightly up-regulated in cold-tolerant cultivars, it displayed constant expression level in the cold-sensitive cultivar. These findings suggest that gene expression profiles of cultivars may become differentiated in response to cold stress depending on the level of tolerance.
Moreover, we retrieved two nuclear transcription factors Y subunit A-3 (NF-Y) targeted by miR169, which was repressed in all genotypes. Similarly, miR169 was induced in response to drought stress and led to repression of the expression of three nuclear factor Y subunit genes.[Citation52] Contrary, multi-drug resistance protein ABC transporter was highly induced in all genotypes, especially in the most cold-tolerant tomato cultivar (LA1777; 5.8 FC). Multi-drug resistance-associated proteins (MRPs or ABCCs) are involved in cellular detoxification by transporting toxic compounds from cytosol into the vacuole.[Citation53] Considering that, cold resistance of tomato genotypes may likely have similar mechanisms in the way MRPs are regulated.
AP2-like transcription factor clade targeted by miR172 has many members. In the analysis, we found six AP2-like transcription factors in tomato as targets of miR172. Expression of AP2-like transcription factors differed based on the cultivars. AP2-like transcription factors such as SGN-U242104 and SGN-U218022 in cv. LA1777, SGN-U224195 and SGN-U226730 in cv. LA1777 and cv. LA4024 were down-regulated. However, SGN-U232455 in all cultivars, SGN-U218022 in cv. LA3969 and in cv. LA4024, and SGN-U214382 in cv. LA3969 showed over-expression. On the other hand, serine/threonine-protein kinase receptor (SGN-U219598) expression was highly induced in the LA3969 and LA4024 cultivars, but was not changed in cv. LA1777.
TIR and TIR-like are involved in the auxin-signalling pathway, and are targeted by miR393.[Citation32,Citation52] SGN-U236908 (TIR) was repressed in all three tomato genotypes. The degradation of TIR mRNAs may subsequently prevent depression of auxin-signalling pathway.[Citation7] Auxin F-box proteins 5 are another target of miR393. Auxin signalling primarily depends on F-box protein receptors and Arabidopsis has four redundant F-box proteins of the TIR1/AFB2 auxin receptor clade.[Citation54] Therefore, F-box proteins are closely related with TIR and TIR-like proteins. In addition to down-regulation of TIR, F-box protein (SGN-U213940 and SGN-U223813) accumulations were also decreased in all genotypes. As regards to SGN-U221001 (B3 domain-containing protein) and SGN-U231414 (U-box domain-containing protein) targeted by miR393, they showed slight changes in the expression levels.
SGN-U216617 (laccase) targeted by miR397 exhibited low level of expression in cv. LA1777 and cv. LA4024, while SGN-U216616 (laccase 7) was over-expressed in cv. LA1777. On the other hand, SGN-U223145 (laccase 5) was repressed in all cultivars, while SGN-U226352 (laccase 3) was not significantly changed in cold-tolerant cultivars. Translocase of chloroplast 34 (Toc34) (SGN-U214046 and SGN-U214047) expression levels were significantly changed in cold-tolerant cv. LA1777. Toc34 is a member of the translocase of the outer membrane complex, which mediates the initial stage of protein import into chloroplasts.[Citation55] Although studies on Toc34 are very limited, cold conditions did not seem to affect the function of Toc34. Interestingly, SGN-U224659 (1-phosphatidylinositol-4,5-bisphosphate phosphodiesterase; PIP2) was repressed in all cultivars. Contrary to our finding, heat stress activated PIP2 accumulation in tobacco.[Citation56]
Comparative analysis
Cold stress is one of the most severe abiotic stresses adversely affecting plants yields by restraining sowing time, causing tissue damage, and stunting growth.[Citation28] Tomato is an important vegetable crop grown worldwide and prone to cold stress. Stress-related functions of miRNAs may provide further insight in response to cold stress. However, little is known about the relation between the miRNAs and cold response.[Citation19]
In this study, miR167a, miR169a, miR172a and miR393 were up-regulated in response to initial phase of cold stress in tomato. Liu et al. [Citation19] found that miR167a, miR393a and miR397a were up-regulated in cold-treated Arabidopsis based on the RT–PCR analysis. Besides, they identified that miR169 and miR172 were induced as a stress response by microarray analysis. Contrary to our finding, miR169 was not detected in cold-stored potato tubers by deep sequencing and degradome analysis.[Citation36] Meanwhile, miR397 was not induced in tomato under cold treatment. On the other hand, the expression levels of miR172, miR393 and miR397 were 1.88, 1.56 and 2.08 FC, respectively, in Arabidopsis.[Citation19] In comparison to tomato, Arabidopsis miR397 was induced, whereas tomato miR397 showed nearly constant expression. In regard to miR393, it showed similar expression in both plants.
The expression of ARF8, targeted by miR167, was higher in the cold-tolerant LA3969 cultivar, while it was at a low level in other cultivars. Although ARF6, targeted by miR167, was induced in cv. LA3969, it did not show significant expression level in cv. LA1777 and cv. LA4024. Therefore, up- and/or down-regulation of miR167 might play a potential role in cold resistance by affecting auxin-signalling pathways.[Citation28] Also, miR393 targets TIR1 and other closely related F-box proteins in Arabidopsis.[Citation12] These proteins are auxin receptors that target repressors of ARF.[Citation31] The different expression profiles of ARF6 and ARF8, and down regulation of TIR1 and F-box protein in cold-tolerant tomato cultivars suggested that auxin perception and signalling may change in stress conditions based on tolerance. In addition to miR167, there could be a key regulatory role of miR393 in the auxin-signalling pathway under cold stress in tomato.
NF-Y is post-transcriptionally regulated by miR169.[Citation57] NF-Y is also known as heme-activated protein or CCAAT-binding factor. Moreover, CCAAT box is present in about 25% of eukaryotic gene promoters.[Citation57,Citation58] Over-expression of NFYB1 in Arabidopsis and maize conferred significantly increased drought resistance.[Citation59] In drought stress, expression of NF-Y genes appears to be decreased in wheat leaves.[Citation60] Similarly, NF-Y expression was down-regulated in response to cold stress in tomato genotypes. This result demonstrated that understanding the biological roles of NF-Y may have potential applications in enhancing stress tolerance in plants. Furthermore, laccase has been proposed as the target of miR397.[Citation8,Citation61] Expression analysis showed that accumulation of four laccase transcripts was differentiated in all cultivars. Laccase is involved in lignin metabolism and it has been reported to be induced under cold conditions in winter wheat.[Citation8] However, it is not clear what the role of laccase is in response to cold.[Citation62]
Stem–loop qRT–PCR analysis showed that miR172 was quickly activated by cold stress. Previous reports on miR172 and AP2 showed that miR172 act on both inhibition of the translation of AP2 and degradation of AP2 transcript.[Citation4] An important role of AP2 is related to the flowering time and phase transition.[Citation14] The expression profile of AP2-like fell within three categories in the analysis: up-regulated, down-regulated or slightly changed in the three tomato genotypes, which suggested the obscure behaviour of this transcription factor. AP2 transcript has been observed to be significantly reduced in tomato leaves agroinfected with tomato leaf curl New Delhi virus as miR172 transcript increased.[Citation63] On the other hand, AP2 expression showed similar expression profile under cold and control conditions in wheat.[Citation31] These data indicate that the detailed investigation on the role of AP2 under cold stress in tomato is needed.
Analysis of the promoter elements of miRNAs revealed that they contain various hormone-regulated cis-acting elements, such as ABRE, ERE, GARE and methyl responsive elements. miR172 and miR393 had GATA-box, which has been proposed to regulate light response [Citation64,Citation65] and to be related to cold stress.[Citation8,Citation66] In plants, most ABA-responsive genes have the conserved ABREs in their promoters, which are significant cis-acting elements for genes responsive to abiotic stress in Arabidopsis.[Citation67] ABA plays an important role in the process of cold acclimation. Although miR393 has an ABRE motif, its expression was not as highly induced as miR172 in tomato. Nevertheless, expression of a number of genes induced by both dehydration and cold has revealed broad variation in the timing of their induction and differences in their responsiveness to ABA.[Citation68] Another significant cold-related motif is the LTRE/DRE/C-repeats.[Citation7] Interestingly, this motif could not be found in our miRNAs. CBFs (C-repeat binding factor) can bind many cold-inducible genes, which have C-repeats/DRE regulatory element in promoters, and can activate their expression.[Citation36] It is known that tomato also has a complete CBF cold-response pathway but its CBF regulon differs from that of Arabidopsis,[Citation37] and the CBF cold-response pathway in tomato is not as important as in cold-acclimated plants.[Citation36] At this point, the absence of LTRE/DRE/C-repeats in the promoter of five analysed miRNAs suggested that ABA-dependent signalling pathways and/or ABA-independent pathways may get involved in the regulation of cold tolerance of tomato. Despite the genome sequencing of tomato, low numbers of miRNAs (48) have been deposited from tomato.[Citation69] In this study, we only analysed five miRNAs. It is also considered that the miRNA members in the same miRNA family often share the same cis-acting motifs in their promoter regions, suggesting that miRNA members of the same miRNA family may respond to the same biotic/abiotic or phytohormone stimuli.[Citation70]
Conclusions
In this study, we analysed some cold-stress-related miRNAs (miR167, miR169, miR172, miR393 and miR397) in tomato at early time points (0, 1, 4 and 16 h) of cold exposure by stem–loop qRT–PCR. In addition, we retrieved the expression profile of predicted target genes from three tomato genotypes, which were obtained from a public database. Different expression profiles of target genes were observed among cultivars. The result suggested that this differentiation among cultivars may highlight cold tolerance of tomato genotypes. Among the miRNAs assessed, miR167, miR169, miR172 and miR393 were up-regulated in early time points of cold stress in tomato. Moreover, the accumulation of the miR172 transcript was larger than that of others. Thus, it can be proposed that these miRNAs are activated in response to the initial phase of cold exposure in tomato. The level of miR397 was not significantly changed under cold conditions. Analysis of cis-elements showed that hormonal/environmental responses regulatory elements were predominant in the tested miRNAs. Furthermore, we proposed putative sly-miR393 with its secondary structure. Expression of predicted target genes showed different expression profile in three genotypes. Thus, we assumed that cold tolerance of tomato genotypes may affect the expression of target genes. However, further studies such as over-expression and/or RNAi strategies are needed to elucidate the precise role of these genes in tomato.
Supplemental data
Supplemental data for this article can be accessed at http://dx.doi.org/10.1080/13102818.2015.1061447.
TBEQ-2015-0097-Supplementary-TableS1.pdf
Download PDF (108.7 KB)Disclosure statement
No potential conflict of interest was reported by the authors.
References
- Axtell MJ, Bartel DP. Antiquity of microRNAs and their targets in land plants. Plant Cell. 2005;17:1658–1673.
- Zhang BH, Pan XP, Cannon CH, et al. Conservation and divergence of plant microRNA genes. Plant J. 2006;46:243–259.
- Sunkar R, Jagadeeswaran G. In silico identification of conserved microRNAs in large number of diverse plant species. BMC Plant Biol. 2008;8(1):37.
- Chen X. A microRNA as a translational repressor of APETALA2 in Arabidopsis flower development. Science. 2004;303:2022–2025.
- Brodersen P, Sakvarelidze-Achard, L, Bruun-Rasmussen M, et al. Widespread translational inhibition by plant miRNAs and siRNAs. Science. 2008;320:1185–1190.
- Varkonyi-Gasic E, Gould N, Sandanayaka, M, et al. Characterisation of microRNAs from apple (Malus domestica ‘Royal Gala’; vascular tissue and phloem sap. BMC Plant Biol. 2010;10:159.
- Zhou X, Wang G, Sutoh K, et al. Identification of cold-inducible microRNAs in plants by transcriptome analysis. Biochim Biophys Acta. 2008;1779:780–788.
- Sunkar R, Zhu J. Novel and stress-regulated microRNAs and other small RNAs from Arabidopsis. Plant Cell. 2004;16:2001–2019.
- Lu S, Sun YH, Shi R, et al. Novel and mechanical stress-responsive MicroRNAs in Populus trichocarpa that are absent from Arabidopsis. Plant Cell. 2005;17:2186–2203.
- Chiou TJ, Aung K, Lin SI, et al. Regulation of phosphate homeostasis by microRNA in Arabidopsis. Plant Cell. 2006;18:412–421.
- Sunkar R, Kapoor A, Zhu JK. Posttranscriptional induction of two Cu/Zn superoxide dismutase genes in Arabidopsis is mediated by downregulation of miR398 and important for oxidative stress tolerance. Plant Cell. 2006;18:2051–2065.
- Jones-Rhoades MW, Bartel DP. Computational identification of plant microRNAs and their targets, including a stress-induced miRNA. Mol Cell. 2004;14:787–799.
- Fujii H, Chiou TJ, Lin SI, et al. A miRNA involved in phosphate-starvation response in Arabidopsis. Curr Biol. 2005;15:2038–2043.
- Aukerman MJ, Sakai H. Regulation of flowering time and floral organ identity by a microRNA and its APETALA2-like target genes. Plant Cell. 2003;15:2730–2741.
- Palatnik JF, Allen E, Wu X, et al. Control of leaf morphogenesis by microRNAs. Nature. 2003;425:257–263.
- Vaucheret H, Vazquez F, Crété P, et al. The action of ARGONAUTE1 in the miRNA pathway and its regulation by the miRNA pathway are crucial for plant development. Genes Dev. 2004;18:1187–1197.
- Guo HS, Xie Q, Fei JF, et al. MicroRNA directs mRNA cleavage of the transcription factor NAC1 to downregulate auxin signals for Arabidopsis lateral root development. Plant Cell. 2005;17:1376–1386.
- Mallory AC, Bartel DP, Bartel B. MicroRNA-directed regulation of Arabidopsis AUXIN RESPONSE FACTOR17 is essential for proper development and modulates expression of early auxin response genes. Plant Cell. 2005;17:1360–1375.
- Liu HH, Tian X, Li YJ, et al. Microarray-based analysis of stress regulated microRNAs in Arabidopsis thaliana. RNA 2008;14:836–843.
- Zuo J, Zhu B, Fu D, et al. Sculpting the maturation, softening and ethylene pathway: the influences of microRNAs on tomato fruits. BMC Genomics. 2012;13:7.
- Liu N, Yang J, Guo S, et al. Genome-wide identification and comparative analysis of conserved and novel microRNAs in grafted watermelon by high-throughput sequencing. PLOS One. 2013;8(2):e57359.
- Fahlgren N, Howell MD, Kasschau KD, et al. High-throughput sequencing of Arabidopsis microRNAs: evidence for frequent birth and death of MIRNA genes. PLOS One. 2007;2(2):e219.
- Griffiths-Jones S, Saini HK, van Dongen S, et al. miRBase: tools for microRNA genomics. Nucleic Acids Res. 2007;36:D154–D158.
- Lu S, Sun YH, Chiang VL. Stress-responsive microRNAs in Populus. Plant J. 2008;55:131–151.
- Varkonyi-Gasic E, Wu R, Wood M, et al. Protocol: a highly sensitive RT-PCR method for detection and quantification of microRNAs. Plant Method. 2007;3:1–12.
- Chen C, Ridzon DA, Broomer AJ, et al. Real-time quantification of microRNAs by stem-loop RT-PCR. Nucleic Acids Res. 2005;33(20):e179.
- Tang F, Hajkova P, Barton SC, et al. MicroRNA expression profiling of singlewhole embryonic stem cells. Nucleic Acids Res. 2006;34(2):e9.
- Lv DK, Bai X, Li Y, et al. Profiling of cold-stress-responsive miRNAs in rice by microarrays. Gene. 2010;459:39–47.
- Thomashow MF. Plant cold acclimation: freezing tolerance genes and regulatory mechanisms. Annu Rev Plant Biol. 1999;50(1):571–599.
- Zhu J, Dong CH, Zhu JK. Interplay between cold-responsive gene regulation, metabolism and RNA processing during plant cold acclimation. Curr Opin Plant Biol. 2007;10(3):290–295.
- Tang Z, Zhang L, Xu C, et al. Uncovering small RNA-mediated responses to cold stress in a wheat thermosensitive genic male-sterile line by deep sequencing. Plant Physiol. 2012;159:721–738.
- Jian X, Zhang L, Li G, et al. Identification of novel stress-regulated microRNAs from Oryza sativa L. Genomics. 2010;95:47–55.
- Zhang J, Xu Y, Huan Q, et al. Deep sequencing of Brachypodium small RNAs at the global genome level identifies microRNAs involved in cold stress response. BMC Genomics. 2009;10:449.
- Levitt J. Responses of plants to environmental stresses: chilling, freezing and high temperature stresses. 2nd ed. Vol. 1, Physiological ecology. London: Academic Press; 1980.
- Ou Y, Liu X, Xie C, et al. Genome-wide ıdentification of microRNAs and their targets in cold-stored potato tubers by deep sequencing and degradome analysis. Plant Mol Biol Rep. 2015;33(3):584–597.
- Liu H, Ouyang B, Zhang J, et al. Differential modulation of photosynthesis, signaling, and transcriptional regulation between tolerant and sensitive tomato genotypes under cold Stres. PLOS One. 2012;7(1):e50785.
- Zhang X, Fowler SG, Cheng H, et al. Freezing sensitive tomato has a functional CBF cold response pathway, but a CBF regulon that differs from that of freezing-tolerant Arabidopsis. Plant J. 2004;39:905–919.
- Pfaffl MW. A new mathematical model for relative quantification in realtime RT-PCR. Nucleic Acids Res. 2001;29(9):e45.
- Kozomara A, Griffiths-Jones S. miRBase: annotating high confidence microRNAs using deep sequencing data. Nucleic Acids Res. 2014;42:D68–D73.
- Dai X, Zhao PX. psRNAtarget: a plant small RNA target analysis server. Nucleic Acids Res. 2011;39(2):W155–W159.
- Zhang BH, Pan XP, Cox SB, et al. Evidence that miRNAs are different from other RNAs. Cell Mol Life Sci. 2006;63:246–254.
- Lin D, Yang Y, Khalil R, et al. SlmiR393 controls the auxin receptor homologous genes expression, and regulates sensitivity to auxin in tomato root growth. Scientia Horticulturae. 2013;162:90–99.
- Etemadi M, Gutjahr C, Couzigou JM, et al. Auxin perception is required for arbuscule development in arbuscular mycorrhizal symbiosis. Plant Physiol. 2014; 166(1):281–292.
- Ambros V, Bartel B, Bartel DP, et al. A uniform system for microRNA annotation. RNA. 2003;9:277–279.
- Lee JY, Colinas J, Wang JY, Mace D, Ohler, U, Benfey PN. Transcriptional and posttranscriptional regulation of transcription factor expression in Arabidopsis roots. Proc Natl Acad Sci USA. 2006;103:6055–6060.
- Zhou X, Ruan J, Wang G, et al. Characterization and identification of microRNA core promoters in four model species. PLOS Comput Biol. 2007;3(3):e37.
- Bustos R, Castrillo G, Linhares F, et al. A central regulatory system largely controls transcriptional activation and repression responses to phosphate starvation in Arabidopsis. PLOS Genet. 2010;6(9):e1001102.
- Rhoades MW, Reinhart BJ, Lim LP, et al. Prediction of plant microRNA targets. Cell. 2002;110:513–520.
- Wu M, Tian Q, Reed J. Arabidopsis microRNA167 controls patterns of ARF6 andARF8 expression, and regulates both female and male reproduction. Development. 2006;133:4211–4218.
- Karlova R, van Haarst JC, Maliepaard C, et al. Identification of microRNA targets in tomato fruit development using high-throughput sequencing and degradome analysis. J Exp Bot. 2013;64:1863–1878.
- Hong SW, Jon JH, Kwak JM, et al. Identification of a receptor-like protein kinase gene rapidly induced by abscisic acid, dehydration, high salt, and cold treatments in Arabidopsis thaliana. Plant Physiol. 1997;113:1203–1212.
- Zhang X, Zou Z, Gong P, et al. Over-expression of microRNA169 confers enhanced drought tolerance to tomato. Biotechnol Lett. 2011;33:403–409.
- Klein M, Burla B, Martinoia E. The multidrug resistance-associated protein (MRP/ABCC) subfamily of ATPbinding cassette transporters in plants. FEBS Lett. 2006;580:1112–1122.
- Windels D, Bielewicz D, Ebneter M, et al. miR393 is required for production of proper auxin signalling outputs. PLOS One. 2014;9(4):e95972.
- Tsai LY, Tu SL, Li HM. Insertion of at Toc34 into chloroplastic outer membrane is assisted by at least two proteinaceous components in the import system. J Biol Chem. 1999;274:18735–18740.
- Mishkind M, Vermeer JE, Darwish E, et al. Heat stress activates phospholipase D and triggers PIP2 accumulation at the plasma membrane and nucleus. Plant J. 2009;60:10–21.
- Ni Z, Hu Z, Jiang Q, et al. GmNFYA3, a target gene of miR169, is a positive regulator of plant tolerance to drought stress. Plant Mol Biol. 2013;82:113–129.
- Mantovani R. The molecular biology of the CCAAT-binding factor NF-Y. Gene. 1999;239:15–27.
- Nelson DE, Repetti PP, Adams TR, et al. Plant nuclear factor Y (NF-Y) B subunits confer drought tolerance and lead to improved corn yields on water-limited acres. Proc Natl Acad Sci USA. 2007;104:16450–16455.
- Stephenson TJ, McIntyre CL, Collet C, et al. Genome-wide identification and expression analysis of the NF-Y family of transcription factors in Triticum aestivum. Plant Mol Biol. 2007;65:77–92.
- Luo Y, Zhou H, Li Y, et al. Rice embryogenic calli express a unique set of microRNAs, suggesting regulatory roles of microRNAs in plant postembryogenic development. FEBS Lett. 2006;580:5111–5116.
- Kruszka K, Pieczynski M, Windels D, et al. Role of microRNAs and other sRNAs of plants in their changing environments. J Plant Physiol. 2012;169:1664–1672.
- Naqvi AR, Haq QM, Mukherjee SK. MicroRNA profiling of tomato leaf curl New Delhi virus (tolcndv; infected tomato leaves indicates that deregulation of mir159/319 and mir172 might be linked with leaf curl disease. Virol J. 2010;7:281.
- Arguello-Astorga G, Herrera-Estrella L. Evolution of light-regulated plant promoters. Annu Rev Plant Physiol Plant Mol Biol. 1998;49:525–555.
- Teakle GR, Kay SA. The GATA-binding protein CGF-1 is closely related to GT-1. Plant Mol Biol. 1995;29:1253–1266.
- Swindell WR. The association among gene expression responses to nine abiotic stress treatments in Arabidopsis thaliana. Genetics. 2006;1824:1811–1824.
- Narusaka Y, Nakashima K, Shinwari ZK, et al. Interaction between two cis-acting elements, ABRE and DRE, in ABA-dependent expression of Arabidopsis rd29A gene in response to dehydration and high-salinity stresses. Plant J. 2003;34:137–148.
- Shinozaki K. Yamaguchi-Shinozaki K. Molecular responses to dehydration and low temperature: differences and cross-talk between two stress signaling pathways. Curr Opin Plant Biol. 2000;3:217–223.
- Gu M, Liu W, Meng Q, et al. Identification of microRNAs in six solanaceous plants and their potential link with phosphate and mycorrhizal signalings. J Integr Plant Biol. 2014;56:1164–1178.
- Ding D, Zhang L, Wang H, et al. Differential expression of miRNAs in response to salt stress in maize roots. Ann Botany. 2008;103:29–38.