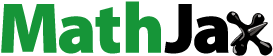
Abstract
Strain L2 (a Bacillus megaterium species that was isolated from tobacco's rhizosphere) was applied to inhibit the pathogen Alternaria alternata in an attempt to reduce tobacco brown spot disease. This study focused on inhibition experiments, in which culture filtrate inhibited A. alternata’s mycelium growth, its spore germination rate and its spore output. The results indicated that an approximately 1.5%–20% concentration of the bacteria-free filtrate, could effectively inhibit hyphae growth. Additionally, bacterial suppression of germ tubes and mycelium growth was observed, rather than lysis. Furthermore, the filtrate caused some of the germinal tubes to undergo vacuolization and even rupturing. The inhibitory effect was associated with the change of the concentration: the greater the concentration, the greater the impact on A. alternata.
Introduction
Alternaria alternata is a brown spot pathogen that was first discovered in the United States in the mid-1950s.[Citation1] Furthermore, the severe leaf spot disease is an economically important disease that develops on mature tobacco leaves during the middle to latter part of the growing season.[Citation2] Unfortunately, the pathogen causes dire economic consequences. For example, since A. alternata directly damages the tobacco yield and quality, the pathogen has caused estimated yearly losses of 3.9 to 21 million dollars to tobacco growers in North Carolina since 1959.[Citation3]
Controlling the disease should be an apparent priority, yet this has proved to be a difficult task. Furthermore, all flue-cured tobacco cultivars are susceptible to the disease.[Citation4] Over the past years, chemical-control methods have been the primary means of combating the brown spot.[Citation5] However, many of the chemicals that are used to control plants' fungal and bacterial diseases are detrimental to animal and human health alike. The truth is that the chemicals persist and eventually accumulate in the natural ecosystems. Consequently, chemical control may not be the most suitable means due to its high cost and environmental risks, associated with its application. As an alternative, several examples of controlling foliar fungal pathogens with bacteria have been reported.[Citation6] It is therefore desirable to replace these chemical agents with biological approaches that are more environmentally friendly.[Citation7,Citation8] Thus, biological control would be highly preferred for disease control in the future.[Citation9]
One option for biological control of the disease is a subset of the total rhizosphere bacteria. It is part of healthy plants' natural microflora and significantly assists health and general soil suppressiveness. In recognition of their beneficial nature, rhizobacteria were termed as plant growth-promoting rhizobacteria (PGPR). As their name suggests, PGPR benefit the host by stimulating plant growth; however, they can also function as a mean of biological disease control.[Citation10] The rhizobacteria also directly reap benefits from this relationship, because plant roots secrete metabolites that the bacteria can utilize as nutrients.[Citation11] This indirect promotion stimulates the biocontrol-nature of PGPR, which can suppress deleterious effects or prevent phytopathogen damage.[Citation7,Citation12–19] Accordingly, efforts to select and apply PGPR for controlling specific soilborne fungal pathogens have been reviewed.[Citation7,Citation20]
In this study, a beneficial microbe (that we termed strain L2) was separated from the rhizosphere of tobacco and rhizosphere soil. Additionally, the mechanism of action was preliminarily investigated and the generation stability of antibacterial substances was inspected. Breed evaluation indicated that the strain L2 is Bacillus megaterium, which is a part of PGPR. We primarily observed and measured the inhibition effect of cell-free filtrate against A. alternata in the Bijie, Guizhou province, in order to understand better how to biologically control the tobacco disease.
Materials and methods
Materials
The microorganisms used in the study were the B. megaterium strain L2 and A. alternata. The indicator fungi, A. alternata, was isolated and preserved by The Institute of Fungi Resource, College of Life Science, Guizhou University. The strain L2 was also isolated by The Institute of Fungi Resource, Guizhou and then preserved in the China Center for Type Culture Collection (Address: Wuhan University, WuHan, China). In order to sufficiently utilize the abundant microbe resources in the Guizhou province, we isolated the strain L2 of B. megaterium from 131 mature period soil samples from the eight prefectures (Qianxi, Dafang, Jinsha, Nayong, Hezhang, Zhijin, Weining countries and Bijie city), Bijie, Guizhou Province. The strain preservation number in the China Center for Type Culture Collection is CCTCCNO: M 2012381.
Media
The potato dextrose agar (PDA) medium consisted of the following: 200 mL potatoes extract (200 g potatoes were extracted in 1 L boiled water for 30 min and then were filtered through cotton gauze; after the extraction, the required distilled water was added to offset the evaporated water), 20 g glucose and 20 g agar, pH 7.0.
The nutrient agar (NA) medium consisted of the following: 10 g beef extract, 10 g peptone, 10 g NaCl, 20 g glucose, 17 g agar and 1000 mL distilled water, pH 7.0–7.2.
The nutrient broth (NB) medium consisted of the following: 10 g beef extract, 10 g peptone, 10 g NaCl, 20 g glucose and 1000 mL distilled water, pH 7.0–7.2.
The oat media plates consisted of the following: 20 g oat meal, 1 mL trace element solution, 18 g agar and 1000 mL distilled water, pH 7.0–7.2.
The trace element solution consisted of the following: 0.1 g FeSO4·7H2O, 0.1 g MnCl2, 0.1 g ZnSO4 and 100 mL distilled water.
Methods
Bacteria-free filtrate preparation
The strain L2 (stored at 4 °C) was inoculated onto plates with NA medium and then cultivated at 30 °C for 48 h. Next, the resulting mixture was inoculated into an NA culture solution and cultivated on a rotary shaker at 30 °C for 3 d (130 r/min). The medium filtrate solution was centrifuged at 5500 r/min for 25 min. The supernatant was collected and filtrated via a 0.22 μm bacterial filter to remove any bacteria. The bacteria-free medium filtrate was prepared and stored until later use.
Experiments exhibiting the inhibitory effects of strain L2 against Alternaria alternata
Alternaria alternata mycelial growth inhibition
After 3 d of cultivation in NA medium, the strain L2 was prepared via a colony diameter method onto culture plates (the final concentrations (V:V) in PDA were 1.5%, 3%, 6%, 10%, 15% and 20% bacteria-free filtrate) with PDA medium. An A. alternata colony (3 mm in diameter) was selected and inoculated onto the centre of the PDA plates; it was then cultivated in a thermostatic incubator at 28 °C. Then, A. alternata’s growing conditions at 3, 5 and 7 d were observed in order to measure and record the colony diameters (mm). For a control, an A. alternata colony with the same diameter was inoculated onto a PDA plate, and no L2 medium filtrate solution was added. Each experiment was repeated three times at 28 °C. The inhibited A. alternata colonies were selected for a microscopic (CX31 Olympus) observation of the mycelial growth. The inhibition rate of the mycelial growth was also observed,
Spore germination inhibition rate
The bacteria-free filtrates (100%, 60% and 20% (V:V), diluted with sterile water) were mixed with A. alternata spore suspensions at a ratio of 1:1 (V:V) (final contents of the bacteria-free filtrates in PDA were 50%, 30% and 10%). Moreover, to serve as control, an NA culture solution that did not have bacteria added was mixed with the A. alternata spore suspension at the same proportions. The mixtures were cultured at 26 °C on concave slides. The spore germination was microscopically (CX31 Olympus) observed after 6, 12 and 24 h. The germination was determined when the length of a germ tube exceeded half of the small-end diameter of the conidia. Each observation was repeated three times for computing the spore germination inhibition rate. At least 150–200 spores were examined in each visual field.
After the L2 bacteria-free filtrates were diluted with sterile water, we determined five concentrations: medium filtrate stock solution, 1:2, 1:4, 1:8 and 1:16 (Vbacteria-free filtrates:Vsterile water). Moreover, an A. alternata conidia suspension was prepared (A. alternata colony after 7 d was scraped into the sterile water and then the hypha was filtered by filtration), with each microliter containing 40–50 spores. The mixtures were cultured on concave slides at 26 °C. After 8–10 h, the conidia's germination was observed under a microscope (CX31 Olympus). The conidia suspension that had medium filtrate broth added to it was used as a control,
Alternaria alternata spore number reduction and sporulation inhibition rate
The inhibitory effects of the bacteria-free filtrates on A. alternata spore production were measured via the mycelial soaking method [Citation21] at different dilution factors: ×1, ×10, ×20, ×40 and ×80. The A. alternata spores were coated onto oat media plates at 26 °C for 2 d. The mycelial plates were soaked in 20 mL of the bacteria-free filtrates for 1 h. After the redundant liquids had been poured out, the plates were blow-dried aseptically and then cultured in the incubator at 26 °C. The control was soaked in the NB culture solution. Each process was repeated three times. At 7 and 14 d, the plates were washed with a 2% sterile glucose liquid and then water was added to 20 mL. The spores were counted, and the inhibition rate was calculated. The conidiophores and conidia chains were observed under a microscope (CX31 Olympus),
Statistical analysis
The data were analysed with Duncan's multiple range test by using standard statistical software (SAS Version 9.2) to assess statistically significant differences among the various treatments.
Results and discussion
The effect of bacteria-free filtrate on Alternaria alternata mycelial growth and morphology
The L2 bacteria-free filtrates in concentrations of 1.5%, 3%, 6%, 10%, 15% and 20% (V:V) severely inhibited the growth of A. alternata’s mycelia (). In the presence of the filtrates, they grew slowly. However, as the bacteria-free filtrates content was increased, the fungal colonies grew in an inversely proportional manner (). After 7 d, the inhibition rate reached 76%, which expressed the good effect of A. alternata on the mycelial growth inhibition. Under a microscopic observation, the A. alternata mycelial growth in the medium containing the bacteria-free filtrate, was obviously affected (A)). The mycelia were swollen (B)), deformed to tubercles (C)) and had obviously gathered bioplasm (D)). Then, the mycelia became swollen into bead-like shapes, the cells ruptured and the cytoplasm oozed out. Moreover, even at low concentrations (1.5%), the bacteria-free filtrate led to the aforementioned changes in the young mycelia (E)). In contrast, the mycelia in the control group were uniformly thin and long with smooth tops (F)). This result was the same as the one in the study of Lin et al. [Citation22] and had a significantly better inhibition effect when compared to the Solanum nigrum extract component.
Figure 1. The inhibitory effects of culture filtrate on A. alternata mycelial growth after seven days. (A) Control; (B) 1.5%; (C) 3.0%; (D) 6.0%; (E) 10.0%; (F) 15.0%; (G) 20.0%.

Table 1. The inhibitory effects of bacteria-free filtrate on A. alternata mycelial growth.
Figure 2. Morphology of L2-inhibited A. alternata mycelium under a microscope. Microscopic magnification (10×) of (A) the inhibited pathogenic mycelium; (B) expansion of the pathogenic mycelium; (C) abnormal growth of the pathogenic mycelium; (D) protoplast aggregation; (E) influence of bacteria-free filtrate (1.5%) on young mycelial morphology; and (F) control.
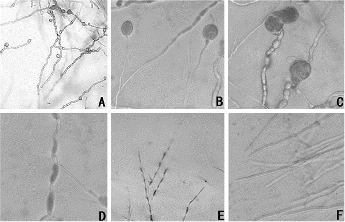
Inhibition of Alternaria alternata spore germination by bacteria-free filtrate
illustrates how the L2 bacteria-free filtrates inhibited the germination of the A. alternata spores. During the germination, the spores first expand upon imbibing water and then grow germ tubes. The standard germination is when the germ tubes' length exceeds half of the spore's diameter. Clearly, after being treated with the stock solution, the majority of the spores was vacuolated and ruptured with cytoplasm exosmosis and thus could not develop into normal mycelia (A)). After treatment with 1:4 bacteria-free filtrates, the spores germinated abnormally and the germinating sites expanded and developed into round bubbles (B)). Regarding the germination time, the spores that were treated with the 1:4 bacteria-free filtrate germinated much more slowly than the control group, but if the abnormal germ tubes were subsequently treated with the stock solution, the previously-inhibited germ tubes were also vacuolated and ruptured along with the increased concentration of bacteria-free filtrates (C)). In contrast, the mycelia in the control group were uniformly thin and long with smooth tops (D)). Therefore, treating A. alternata spores with the L2 bacteria-free filtrates ultimately led to two consequences: (1) the spores vacuolated and ruptured, (2) the germ tubes developed abnormally and could continue to grow, but the germination time was significantly longer than a normal germination time. In conclusion, the bacteria-free L2 filtrates could obviously restrain the spore germination.
Figure 3. The effect of bacteria-free filtrate on A. alternata spore germination (640×). (A) Germ tube vacuolization; (B) abnormal germination of germ tubes; (C) germ tube vacuolization with high-concentration treatment; (D) control.
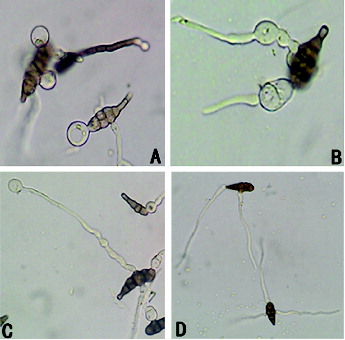
shows that the L2 bacteria-free filtrates could all inhibit the spore germination at concentrations of 50%, 30% and 10%, but at 10%, the majority of spores developed germ tubes with only a small segmentation of the mycelia. Furthermore, the germ tubes were thick-short, slightly expanded and could not develop into mycelia. Obviously, the 10% concentration still inhibited the germination of A. alternata spores.
Table 2. The effect of culture filtrate on A. alternata spore germination.
Inhibition of Alternaria alternata sporulation by bacteria-free filtrate
According to , the bacteria-free filtrate's inhibition of A. alternata spore germination declined when the dilutions increased. Different final concentrations of the bacteria-free filtrates had different inhibition effect against A. alternata spore germination. At greater concentrations, the inhibition was more obvious. Besides, according to the data, the inhibition effect was not observed after the bacteria-free filtrates were diluted 80 times. Furthermore, the inhibition after 14 d still had a great effect, compared with the inhibition after 7 d. Through the examination under a microscope, we found that the conidiophores and conidia chains, which were disposed to the coarse metabolites, were sparse and short. The inhibited conidiophores were teratogenic (A)). Meanwhile, the quantity of the spore germinates clearly declined and the inhibited spore chains were been teratogenic ((B)).
Table 3. A. alternata spore number reduction and sporulation inhibition rate.
Final remarks
During biological prevention, the key to bio-controlling antagonistic bacteria is to control the diseases at an early stage. The most fundamental work concerning bio-controlling methods incorporate the screening of antagonistic bacteria (the best bio-control factor).[Citation23] Furthermore, Bacillus has a set of distinctive advantages that makes it suitable for a bio-control material; this claim is demonstrated in the following explanations. Bacillus is easily screened because it is autologous, heat-resisting, generates spores, rhabditiform and it is Gram (+). Those and other factors, may make Bacillus a great bio-control material in the future. On the other hand, most of Bacillus' excellent bio-control properties are closely associated with the strong antagonistic substance that is generated by the bacterial strains. According to Elliott et al.,[Citation24] field applied chemical pesticides did not produce any negative effects for Bacillus strains. This indicates that the integrated use of biological and chemical-control measures were steady. Using bio-control agents to effectively suppress plant diseases is a key to control the early development of a disease.[Citation25]
Conduction of research works into a larger field is encouraged in order to successfully biocontrol a regulated environment. Practical field control will depend on having an adequate introduction of the control agent at the appropriate times.[Citation7]
Conclusions
In this study, the bacterial suppression of germ tube and mycelium growth of A. alternata was observed, rather than lysis. Our results indicated that the antagonistic substances produced by L2 were effective on the young mycelia even at very low concentrations. They also obviously inhibited the germination of A. alternata spores so that the spores were vacuolated, ruptured, or abnormally developed, thus slowing the germination. L2 most likely produced an antibacterial substance that inhibited the early germination, the A. alternata spores forming and the extension of germ tubes. Therefore, the antimicrobial substances were very stable and effective. This is the first time to illustrate the inhibition of A. alternata spore germination by antagonistic bacterium metabolites. It clearly showed the effect of antagonistic bacterium metabolites against pathogenic fungus growth. Currently, we have applied strain L2 medium filtrate to a Guizhou Province field and have verified the effect stepwise. Further work is necessary to determine the effective substances of this bacterium for pathogen inhibition and for growth-promoting effects on plants.
Acknowledgements
Zhu Li and Bokai Guo contributed equally to this article and should be considered co-first authors.
Disclosure statement
No potential conflict of interest was reported by the authors.
Additional information
Funding
References
- Lucas GB. Diseases of tobacco. Santa Cruz: Biological Consulting Associates; 1975. p. 32–57.
- Spurr HW. Protective applications of conidia of nonpathogenic Alternaria sp. isolates for control of tobacco brown spot disease. Phytopathol. 1977;77:128–132.
- Stavely JR, Main CE. Influence of temperature and other factors on initiation of tobacco brown spot. Phytopathol. 1970;60(11):1591–1596.
- Todd FA. Tobacco disease control practices for 1970. Raleigh: NC Agronomic Extension Services Miscellaneous Pubication; 1969. p. 28–45.
- Chen RT. China tobacco cultivation. Shanghai: Shanghai Scientific & Technical Publishers; 1987.
- Fravel DR, Spurr HW Jr. Biocontrol of tobacco brown-spot disease by Bacillus cereus subsp. mycoides in a controlled environment. Phytopathol. 1977;67(7):930–932.
- Glick BR, Bashan Y. Genetic manipulation of plant growth-promoting bacteria to enhance biocontrol of phytopathogens. Biotechnol Adv. 1997;15(2):353–378.
- Sindhu SS, Rakshiya Y, Sahu G. Biological control of soilborne plant pathogens with rhizosphere bacteria. Delhi: Research India; 2009. p. 311–330.
- Nguyen MT, Ranamukhaarachchi SL, Hannaway DB. Efficacy of antagonist strains of Bacillus megaterium, Enterobacter cloacae, Pichia guilliermondii and Candida ethanolica against bacterial wilt disease of tomato. Phytol J. 2011;3(2):1–10.
- Kloepper J, Rodriguez-Kabana R, Zehnder GW, et al. Plant root-bacterial interactions in biological control of soilborne diseases and potential extension to systemic and foliar diseases. Australas Plant Pathol. 1999;28(1):21–26.
- Lugtenberg B, Kamilova F. Plant-growth-promoting rhizobacteria. Annu Rev Phytopathol. 2009;63:541–556.
- O'sullivan DJ, Fergal OG. Traits of fluorescent Pseudomonas spp. involved in suppression of plant root pathogens. Rev Microbiol. 1992;56(4):662–676.
- Sivan A, Chet I. Microbial control of plant diseases. New York (NY): Wiley-Liss; 1992. p. 335–354.
- Sutton JC, Gang P. Manipulation and vectoring of biocontrol organisms to manage foliage and fruit diseases in cropping systems. Annu Rev Phytopathol. 1993;31(1):473–493.
- Cook RJ. Making greater use of introduced microorganisms for biological control of plant pathogens. Annu Rev Phytopathol. 1993;31(1):53–80.
- Chet I, Inbar J. Biological control of fungal pathogens. Appl Biochem Biotechnol. 1994;48(1):37–43.
- Dowling DN, O'Gara F. Metabolites of Pseudomonas involved in the biocontrol of plant disease. Trends Biotechnol. 1994;12(4):133–141.
- Pankhurst CE, Lynch JM. The role of soil microbiology in sustainable intensive agriculture. Adv Plant Pathol. 1995;11:229–247.
- McLaughlin RJ, Wilson L, Chalutz E. Inhibiting plant pathogens with an antagonistic microorganism(s). Biotechnol Adv. 1996;14(3):385.
- Kloepper JW, Metting FB Jr. Plant growth-promoting rhizobacteria as biological control agents. New York (NY): M. Dekker; 1992. p. 255–274.
- Fang Dunhuang, Wu Zujian, Deng Yunlong, et al. Screening of antagonistic rhizospheric spore-producing bacilli against tobacco brown spot. Acta Phytopathologica Sinica. 2006;36(6):551–561. Chinese.
- Lin TC, Fan MC, Wang SY, et al. Identification of the Solanum nigrum extract component involved in controlling cabbage black leaf spot disease. Agric Food Chem J. 2011;59(5):1667–1672.
- Yang XY. Study of the biological control of non pathogenic bacteria to brown spot. Chin Tob Sci. 2000;3:47–48. Chinese.
- Elliott ML, Des Jardin EA, Batson WE Jr, et al. Viability and stability of biological control agents on cotton and snap bean seeds. Pest Manag Sci. 2001;57(8):695–706.
- Guetsky R, Shtienberg D, Elad Y, et al. Combining biocontrol agents to reduce the variability of biological control. Phytopathol. 2001;91(7):621–627.